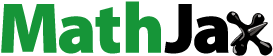
Abstract
Smart phase transformation systems@hard capsule (SPTS@hard capsule) based on lyotropic liquid crystalline (LLC) were developed for oral sustained release in this study. Doxycycline hydrochloride (DOXY) and meloxicam (MLX) were used as hydrophilic and hydrophobic model drug, respectively. Two systems were added with different additives, that is, gelucire 39/01, PEG 1000 and Tween 80 to adjust their melting point and release profiles. The phase transformation of these systems could be triggered by water as well as temperature. They could spontaneously transform into cubic phase or hexagonal phase when coming across with water, to achieve the 24 h sustained release profile. In addition, the obtained systems could switch between semisolid state and liquid state when temperature changed within room temperature and body temperature, which facilitated the phase transformation in gastrointestinal tract and during their encapsulation into hard capsules. LLC-based SPTS@hard capsule revealed potential for the industrialization of its oral administration on account of its drugs accommodation with different solubility, controllable release profile and simple preparation process.
Introduction
Lyotropic liquid crystalline (LLC) is self-assembled nanostructured gel that can be formed by amphiphilic lipids and surfactant in aqueous environment (Wang et al., Citation2019). It has multiple crystal structures, that is, lamellar, cubic and hexagonal (Qian et al., Citation2015; Mei et al., Citation2017a). The special structures provide incorporation of drugs with different solubility, including hydrophilic, hydrophobic and amphiphilic molecules (Conn & Drummond, Citation2013; Rajabalaya et al., Citation2017) and also tunable release rate (Rizwan & Boyd, Citation2015; Abd-Allah et al., Citation2016; Huang & Gui, Citation2018). LLC exhibits perfect performance on mucosal (Huang et al., Citation2017), transdermal (Peng et al., Citation2015; Musa et al., Citation2017), periodontal (Mei et al., Citation2017a; Zheng et al., Citation2018), subcutaneous (Mei et al., Citation2017b) and other parenteral drug delivery (Wan et al., Citation2015; Qin et al., Citation2016), due to its high drug-loading capacity, appropriate viscoelasticity and good biocompatibility (Abd-Allah et al., Citation2016; Kulkarni & Thareja, Citation2016).
However, the oral application of LLC is still limited by its high viscosity. Cubosomes or hexasomes with reduced viscosity (Peng et al., Citation2015), prepared by high-energy fragmentation (e.g. ultrasonic, high-speed shear and high-pressure homogenization), are limited by the practical hurdles associated with both preparation and storage instability, which results in drug delivery failure (Mei et al., Citation2017c). Despite the LLC precursor solution of low viscosity could be formulated with organic solvent, such as N-methyl pyrrolidone, N, N-dimethyl acetamide and ethanol for injection (Pan et al., Citation2013; Thakur et al., Citation2014), this strategy does not make sense for oral administration owing to its irritation and unsafety to gastrointestinal tract (GI tract). Moreover, the capsulation of precursor solution into soft capsules is accompanied with the risk of leakage and instability (Venkatesh et al., Citation2014).
In order to realize the application of LLC in oral sustained release administration, smart phase transformation systems (SPTSs) with two types of phase transformation properties triggered by water as well as temperature were developed. Appropriate additives were introduced to adjust their melting point (MP) and release profiles for hydrophilic and hydrophobic drugs, respectively. In this approach, the obtained systems could switch between semisolid state and liquid state when temperature changed within room to body temperature. That means under the room temperature, the content within the hard capsules is semi-solid status, during the encapsulation of the LLC. After the oral administration, the content within the hard capsule is prone to change to liquid status, which may facilitate the phase transformation from lamellar phase or cubic phase to hexagonal phase triggered by water. The transformation into the viscous hexagonal phase helped to achieve the 24 h sustained release profile. On the other hand, they would exhibit liquid state when temperature rose during the preparation process and turned into semisolid state after encapsulation into hard capsules. Compared with cubosomes and hexasomes, LLC-based SPTSs were free of the instability issues brought by high energy fragmentation process. Also, the subsequent storage instability issues could be overcome as the SPTSs were stored in a semisolid stable form. Moreover, the transformation of LLC-based SPTSs from lamellar phase to cubic or hexagonal phase would be triggered by water uptake after the semisolid-liquid transformation.
Glyceryl monooleate (GMO) and phytantriol (PYT) were the most common materials for the fabrication of LLC (Leung & Leal, Citation2019; Jie et al., Citation2019). GMO was chosen as the model lipid material in present study, which possessed lower MP and desirable biodegradability comparing with PYT (Qin et al., Citation2016; Owens et al., Citation2016). GMO was proved as excipients for oral administration in many countries (Nardin & Köllner, Citation2019). It could be cleaved by pancreatic lipase into oleic acid and glycerol, due to the ester bond in GMO (Zhou et al., Citation2014). Therefore, the biosafety of oral administration of SPTSs could be secured. The designed SPTSs was based on GMO. Doxycycline hydrochloride (DOXY) and meloxicam (MLX) as hydrophilic and hydrophobic model drug were selected for two SPTS@hard capsules, respectively (Pokharkar et al., Citation2015; Chaturvedi et al., Citation2017). Appropriate additives were utilized in the two SPTSs, that is, gelucire39/01, PEG 1000 and Tween 80 to adjust their MP and release profiles. Two SPTS@hard capsules with 24 h sustained release profiles would be established with smart phase transformation triggered by both water and temperature. The novel SPTSs provided a valuable insight on the industrialization of oral application of LLC, on account of their accommodation to drugs with different solubility, controllable release profile and simple preparation process.
Materials and methods
Materials
Glycerol monooleate was obtained from Danisco Co., Ltd. (GMO, 4011022826, Shanghai, China). Doxycycline hydrochloride (DOXY, 0911047, 99.2%, Suhai Co., Ltd., Jiangsu, China) and meloxicam (MLX, CML20090313H, Wuhan Chengyuan Tec Co., Ltd., Hubei, China) were selected model drugs. Gelucire 39/01 (7E1406-2, Gattefosse SAS Co., Ltd., Shanghai, China), polyethylene glycol 1000 (PEG 1000, Shantou Xilong Che Co., Ltd., Guangdong, China) and polysorbate (Tween 80, 20090701, Damao Che Co., Ltd., Tianjin China) were additives. DOXY tablets and MLX tablets for reference were obtained from South China Pharmaceutical Group Co., Ltd. (50 mg/tablet) and Boehringer Ingelheim (7.5 mg/table), respectively. All other chemicals were of chemical grade.
Saturated solubility
The saturated solubility of DOXY and MLX in different media was measured. One gram of DOXY and MLX was added into 100 mL of pH 1.2 HCl, pH 7.2 PBS and deionized water (pH 6.9), respectively. The suspension was shaken at 37 °C for 24 h. The supernatant filtered through 0.45-μm micro-porous was assayed by HPLC-UV at corresponding wavelengths. The wavelengths were determined according to the maximum absorption without interference of excipients. For DOXY, the wavelengths in pH 1.2 HCl, pH 7.2 PBS and deionized water (pH 6.9) were 268 nm, 274 nm and 273 nm, respectively. Those for MLX were 349 nm, 362 nm and 361 nm, respectively.
Preparation of SPTS@hard capsules
Mixtures of GMO, DOXY and gelucire 39/01, or mixtures of GMO, MLX, PEG 1000 and Tween 80 at different ratios were melted, vortex-mixed and stirred homogeneously in 45 °C water bath for 3 h, and dropped into gelatin capsules (0#, Lot. 12834221, Suzhou Capsule Co., Ltd.) when they were hot, then stayed overnight at room temperature. The homogeneity of the mixtures in capsules was visually inspected.
Melting properties of SPTSs and excipients
Differential scanning calorimetry (DSC, STA 409PC, Nestal Co. Ltd., Germany) was used to determine the melting properties of SPTSs and drugs. Approximately 5 ∼ 10 mg of samples was accurately weighed into aluminum pans, and then examined at a heating rate of 5 °C/min from 20 °C to 50 °C under nitrogen purge of 20 mL/min.
In vitro drug release and swelling behavior of SPTS@hard capsules
In vitro release of MLX and DOXY from the SPTS capsules were measured by the paddle method (Ch.P. 2015, appendix XC) under a rotate speed of 100 rpm and temperature of 37 ± 0.5 °C. The dissolution mediums were phosphate-buffered saline (pH 7.2) for MLX and degassed distilled water for DOXY, respectively, which were selected according to Ch.P 2015. At certain time intervals, 5 mL release medium was withdrawn and replaced by fresh medium. The sample solutions were analyzed by ultraviolet spectroscopy (UV, TU-1901, Beijing general Co. Ltd., China). The influence of drug solubility, drug loading as well as proportion of additives (PEG 1000, Tween 80 and gelucire 39/01) on release profiles of SPTS@hard capsules were investigated.
The optimized SPTS@hard capsules for DOXY and MLX were compared with commercial DOXY and MLX tablets, respectively, in terms of release profile. Their drug release data were plotted according to mathematic kinetics of zero order, first-order, Higuchi and Hixson-Crowell model.
To explore the in vitro drug release mechanism, the swelling behaviors of the SPTSs were investigated by determining their water uptake. Briefly, SPTSs were molded and weighed into glass bottles. 8 mL deionized water was gently added into the precursors, then the samples were horizontally shaken at 100 rpm in 37 ± 0.5 °C water bath. At fixed time intervals, the weight of the SPTSs was determined after removal of excess liquid by toppling downward the tube and gently wiping the sample with filter paper. Then 8 mL fresh deionized water was added on the surface of the matrix. The weight data were plotted versus time according to mathematic kinetics of first-order and second-order kinetic equations (Souza et al., Citation2014). The influence of drug solubility, drug loading, proportions of additives (PEG 1000, Tween 80 and gelucire 39/01) on swelling behaviors of SPTSs were investigated.
Phase transformation study of SPTSs
The phase transformation of SPTS from semisolid to liquid and from precursor to gel was comprehensively explored by visual, polarized light microscope (PLM, Mshot Instruments Ltd, Guangzhou, China) and small angle X-ray scattering (SAXS, SAXSee, Anton Paar, UK) method. For the visual test, each SPTS@hard capsules was placed in vials with 5 mL distilled water or hydrochloric acid solution (pH 1.2) under 37 ± 0.5 °C. Changes of appearance at certain time intervals were photographic recorded.
The dynamic phase transformation process of SPTSs upon contacting with distilled water or PBS was observed with PLM at 37 ± 0.5 °C by observing the birefringence texture. SPTS@hard capsules of DOXY and MLX were withdrawn from distilled water and PBS respectively at different time intervals. The photographs were taken using a MD50 digital camera.
The crystalline structures of the SPTSs were characterized by the SAXS at 37 ± 0.5 °C. Samples were measured at 50 mA and 40 kV using a PW3830 X-ray generator (PANalytical) with the X-ray source of Cu Kα radiation (λ = 0.1542 nm). The intensity versus scattering factor (q) was recorded.
Results and discussion
Preparation and melting properties of SPTS@hard capsules
To establish the SPTS, which could be triggered by temperature as well as water uptake, materials selected in this study had low MP around body temperature to realize its semisolid–liquid transformation in GI tract. The MP of GMO was 34.1 °C. Hence, excipients with low MP including gelucire 39/01 with MP of 39.5 °C and PEG 1000 with MP of 39.8 °C were selected for the GMO-DOXY and GMO-MLX systems to regulate the release profiles, respectively. Stable and homogeneous lipids with moderate viscosity could be obtained after stirring in 45 °C water bath for 3 h. The mixtures of GMO-DOXY-gelucire39/01 and GMO-MLX-PEG 1000-Tween 80 showed no explicit MP which may disappeared due to the amorphous state, while their broad melting peak shift from 25 °C to 39 °C when the proportion changes. No phase separation, degradation and oxidation were observed from the results of DSC thermograms (seen in supporting information). Since the MP of DOXY and MLX were 159.92 °C and 265.45 °C, respectively (Bashiri-Shahroodi et al., Citation2008; Legendre et al., Citation2012). Solid solution or suspension of drugs was speculated to be formed.
In vitro release behavior and mechanism
To offer stable drug concentrations, the optimal sustained drug delivery system was expected to deliver definite amount of drug at a desirable rate over a duration time. Drug and additives had great impact on the in vitro release behaviors. Therefore, the in vitro drug release behavior was evaluated with drug solubility, drug loading, proportion of PEG 1000, Tween 80 and gelucire 39/01 as variables.
The saturated solubility of DOXY and MLX in different media was summarized in . It was indicated that DOXY was hydrophilic and MLX was hydrophobic. In addition, higher saturated solubility could be observed in deionized water for DOXY than in PBS and HCl, while MLX had the highest saturated solubility in PBS. Therefore, deionized water and PBS was chosen as release mediums for DOXY and MLX in further study.
Table 1. Saturated solubilities of drugs in different medium (n = 3).
For the DOXY-SPTS@hard capsules, DOXY exhibited obvious initial burst release (). While for the water-insoluble loaded system, MLX exhibited an incomplete release at 24 h (). Drug release from DOXY-SPTS@hard capsules increased with the increase of drug loading, which was conversely applied to MLX-SPTS@hard capsules. Similar phenomenon was found in previous researches (Kumar & Mishra, Citation2006; Khampieng et al., Citation2014; Iqbal et al., Citation2017).
Figure 1. Drug release and water uptake of GMO-DOXY system (A, C) and GMO-MLX system (B, D) (drug loading: 2.5%, 5%, 7.5%, 37 °C, n = 3).
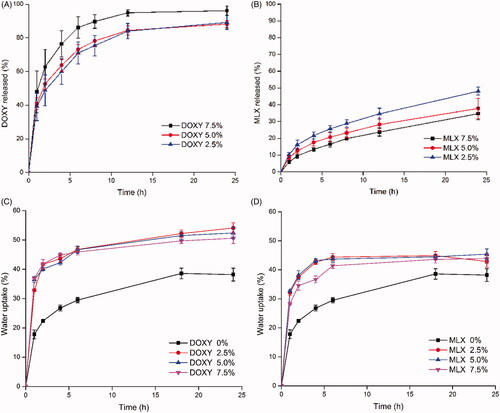
GMO possesses the capability of swelling in the presence of water and forming various types of liquid crystalline phases. The kinetic of water uptake of different systems were also investigated. showed a quick water uptake in the first 2 h and a slow-down for GMO. The equilibrium water uptake of GMO-matrix was 38.2%. The slow-down might be attributed to the formation of cubic phase or hexagonal phase, which was highly viscous and its close packed structure of crystalline cell retarded further absorption of water. For the drug involved SPTS, the initial water-uptake raised sharply with the incorporation of drug. While the release rate increased with the increasing DOXY content and the decreasing MLX content.
Hydrophilic molecules insert into the polarity side of continuous bilayers, and enlarge the size of water channel. The enlarged water channel could accelerate the drug release rate in LLC system. Large hydrophilic molecules such as PEG1000 can hardly insert into bilayers due to its large molecular dimension and had strong potency to enlarge the water channel. For the hydrophilic small molecular drug DOXY, the enlarged water channel accelerated its release rate. Yet, the release of DOXY would be retardant due to its interaction with the polarity side of continuous bilayers.
For LLC system with 2.5% and 5% DOXY loading, drug tends to be distributed on the surface of the polarity side of continuous bilayers. Compared with the LLC system with 2.5% DOXY loading, a higher drug-bilayer interaction may occur in the LLC system with 5% DOXY loading, leading to a looser structure of bilayers. The loosen structure of bilayers was benefit to drug release, while the interaction with bilayers was against the drug release. For LLC system with 7.5% DOXY loading, a considerable portion of drug located in the enlarged water channel besides of those on the surface of the polarity side of continuous bilayers. Therefore, the systems with 2.5% and 5% drug loading showed comparable release of DOXY. And the system with 7.5% drug loading showed enhanced drug release.
By plotting the data of water uptake during 24 h according to the second-order kinetics (Li et al., Citation2014), linear relationship was obtained with linear correlation coefficients over 0.99 for both systems. The equilibrium water-uptakes after 24 h were approximately 52.5% and 45.1% for GMO-DOXY matrix and GMO-MLX matrix, respectively. The incorporation of DOXY had greater impact on the water uptake of GMO system.
Three stages are mainly included for drug release: penetration of release medium into SPTS, dissolution of drug, diffusion of drug solution from SPTS into the release medium (). The rapid initial water-uptake of GMO system incorporated with drug referred to the first stage. In this step, the semisolid–liquid transformation triggered by temperature would accelerate the penetration of release medium into SPTS, as well as the transition from lamellar phase into cubic or hexagonal phase with high viscosity. As for the step 2, dissolution of DOXY took place rapidly since it has high solubility in medium, resulting in the initial burst release. For MLX, the dissolution process may be difficult due to its poor water solubility. Additionally, the possible formation of MLX solution in melted lipids in GMO-MLX system could help the dissolution process of drug. Therefore, the GMO-MLX system did not show slow drug release rate as expected. Subsequently, the generated cubic or hexagonal phase with high viscosity hindered the diffusion of drug solution from SPTS into the release medium to slow down the water uptake and provide sustained release profiles.
The property of drug had great impact on their distribution in crystalline cell of cubic or hexagonal phase. Water channels and lipid bilayers built up the crystalline cell. Hydrophilic DOXY was most distributed in the water channels, where the release medium could penetrate in, and hence a rapid release was expected. Hydrophobic MLX would migrate into the lipid bilayers. Thus, its released from the crystalline cell had to break through the oil–water interface first, resulting a low release rate and incomplete release content. In addition, the hydrophilic property of DOXY was against the transformation process from lamellar phase with low viscosity to cubic or hexagonal phase with high viscosity, which benefited the water uptake and drug release. On the contrary, the attendance of MLX favored this process. The influence of hydrophilic molecules and hydrophobic molecules on the phase transformation of LLC can be explained in terms by the surfactant packing parameter P:
(1)
(1)
where v is the partial volume of the hydrophobic group, a is the area of head group, and l is the average length of the hydrophilic group (Mulet et al., Citation2013; Yamada, Citation2015).
The hydrophilic DOXY mainly located in the hydrated region and might have interaction with the polar group of lipid, which increased volume proportion of polar region, namely a lower p value. Thus, it provided the tendency of maintaining lamellar phase with high capacity of water channels. On the contrary, the addition of hydrophobic MLX increased the p value by decreasing the volume proportion of polar region, and drove the transformation from lamellar to cubic or hexagonal phase (Landh, Citation1994).
The same impact on the phase transformation could be expected with the incorporation of additives with hydrophilic or hydrophobic property. As mentioned above, GMO-DOXY system exhibited obvious initial burst release and GMO-MLX exhibited an incomplete release at 24 h. Therefore, additives with different properties were necessary for the SPTSs to adjust the phase transformation and drug release. The drug loading 5% for DOXY system and 2.5% for MLX system were selected for the further study, repsectively.
For GMO-DOXY system, hydrophobic additive gelucire 39/01 whose MP was around 39 °C was cooperated to accelerate the phase transformation from lamellar phase to cubic or hexagonal phase and reduce the initial burst release. With DOXY content fixed, gelucire 39/01 range from 0% to 15% was added in the GMO-DOXY system. revealed that a significant reduce in the initial water uptake was caused by the addition of gelucire 39/01, which had no concern with the amount of gelucire 39/01. GMO-DOXY with 5% and 10% gelucire 39/01 showed the lowest initial water uptake. SPTSs with 5% gelucire 39/01 exhibited the lowest drug release rate with a complete release at 24 h (). The water uptake and drug release profile were satisfactory when the content of gelucire 39/01 was set as 15%.
Figure 3. Effect of gelucire 39/01 content on drug release (A) from GMO- DOXY- gelucire 39/01 system and water uptake (B) (DOXY loading 5%, 37 °C, n = 3).
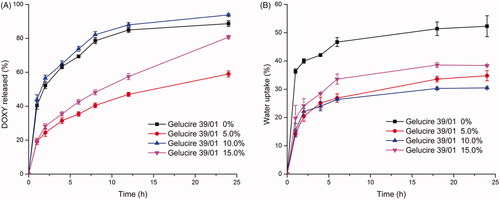
For GMO-MLX system, hydrophilic additive PEG 1000 whose MP was around 39 °C was cooperated to weaken the tendency of phase transformation from lamellar phase to cubic or hexagonal phase, and accelerate the water uptake and drug release rate. With MLX content fixed to 5%, 0% to 15% PEG 1000 were added in the GMO-MLX system. revealed that a significant increase in the water uptake was caused by the addition of PEG 1000, which was in positive correlation with the additive amount of PEG 1000. In corresponding, the drug release rate increased with the increment of PEG 1000 (). GMO-MLX with 15% PEG 1000 showed the highest increase in water uptake as well as the highest drug release rate. However, an incomplete release at 24 h was still observed with the addition of PEG 1000. Thus, Tween 80, a hydrophilic surfactant was selected to facilitate the complete release of MLX with the content of PEG 1000 fixed at 15%.
Figure 4. Effect of PEG 1000 content on drug release (A) from GMO- MLX- PEG 1000 system and water uptake (B) (MLX loading 2.5%, 37 °C, n = 3).
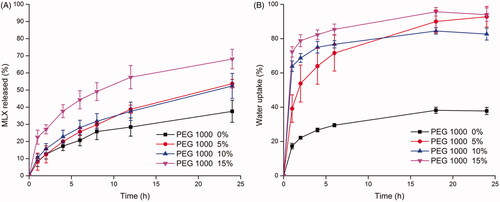
As shown in , the presence of Tween 80 further increased the water uptake of GMO-MLX-PEG 1000 system. While the amount of Tween 80 exhibited no difference in the water uptake profiles. Water uptake with above 45% at 1 h and equilibrium at 6 h were obtained for the Tween 80 incorporated system. As to the drug release profiles, a complete release at 24 h of about 80% MLX were obtained with the presence of Tween 80. Similarly, the amount of Tween 80 exhibited no difference in the drug release profiles. Tween 80, as a nonionic surfactant, has certain irritation due to its damage to cell membrane (Hua et al., Citation2018). Although it was reported that no obvious toxicity was observed in rats and mice after a long time administration of fodder with 5% Tween 80 (Shah & Paradkar, Citation2005), 2.5% Tween 80 was chosen for the sake of less probability of toxicity and side effect.
Figure 5. Effect of Tween 80 content on drug release (A) from GMO-MLX-PEG 1000-Tween 80 system and water uptake (B) (MLX loading 2.5%, PEG 1000 15%, 37 °C, n = 3).
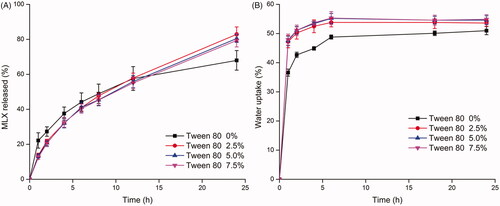
It is interesting that it has a good chance for the synergistic release behaviors occurring in a LLC system loaded with both hydrophilic and hydrophobic drugs. According to the results above, hydrophilic drug may present an initial burst release, while for the hydrophobic drug may exhibit a rather slow release. For a LLC system loaded with both hydrophilic and hydrophobic drugs, the appearance of hydrophilic drug may facilitate the release of the hydrophobic drug by promoting the water uptake and further retarding the phase transition from lamellar phase/cubic phase into hexagonal phase. On the contrary, the appearance of hydrophobic drug may retard the release of the hydrophilic drug by decreasing the water uptake and further promoting the phase transition from lamellar phase/cubic phase into hexagonal phase. To be specific, the synergistic release behaviors rely on the balance between the opposite impaction from the two drugs. The balance could be influenced by many factors, such as the drug loading, the logP value of drug and so on. In our previous study, a LLC system loaded with silver nanoparticles and chlorhexidine acetate was established by us. No synergistic release behaviors were observed due to the same hydrophilic property and the huge different in size of two drugs (Zheng et al., Citation2018). Further study on the LLC system loaded with both the hydrophilic and hydrophobic drugs is meaningful and to be conducted in the near further.
In vitro release of optimized SPTS@hard capsules compared with commercial tablets
From the above, the optimized DOXY-SPTS@hard capsules was consisted of 5% DOXY, 80% GMO and 15% gelucire 39/01, and MLX-SPTS@hard capsules was consist of 2.5% MLX, 80% GMO, 15% PEG 1000 and 2.5% Tween 80. In vitro release profiles of the optimized SPTS@hard capsules and their corresponding commercial tablets were presented in . SPTS@hard capsules for both DOXY and MLX exhibited sustained release profiles with less than 20% drug release in 1 h and more than 80% in 24 h. It was proved that the SPTS for hydrophilic DOXY overcame the high initial burst release issue, and the SPTS for hydrophobic MLX achieved complete release in 24 h. However, the cumulative release amount of both DOXY-SPTS@hard capsules and MLX-SPTS@hard capsules were lower than those of commercial tablets. It might be a result of the interaction between drugs and the LLC materials, which was corresponding with reports (Nazaruk et al., Citation2018).
Figure 6. Release profiles of optimized SPTS@hard capsules and commercial tablets: DOXY in water (A) and MLX in pH 7.2 PBS (B) (37 °C, n = 3).
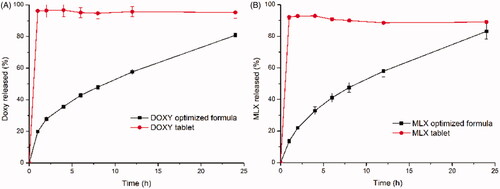
The drug release data from DOXY-SPTS@hard capsules with different ratio of gelucire 39/01, and MLX-SPTS@hard capsules with different ratio of PEG 1000 and Tween 80, were plotted according to mathematic kinetics of zero order, second order, Higuchi and Hixson-Crowell model. Several linear relationships were obtained (data not shown). Among them, the linear relationships following Higuchi model had the highest linear correlation coefficients, 0.9991 for DOXY-SPTS@hard capsules and 0.9990 for MLX-SPTS@hard capsules. The release mechanism for SPTS@hard capsules was mainly to diffusion, and subsidiarity dissolution, which was in conformance to the skeleton sustained release preparation (Lampis et al., Citation2018; Kumari et al., Citation2018).
Phase transformation study
Change of appearance of the SPTS@hard capsules from semisolid to liquid and from precursor to gel was observed by visual at certain time intervals in different medium of 37 °C (). The medium showed no appreciable impact on the phase transformation process. SPTS@hard capsules transformed from semisolid to liquid, which was evidenced by the shallowed color of SPTSs at 0.5 min and the leakage of SPTSs along with the dissolution of capsule shells at 5 min. The mediums became turbid at 1 h, but the SPTS@hard capsules maintained its integrated outline as a capsule after 24 h, indicating the phase transition from ‘liquid’ into ‘gel’, that is from lamellar phase into cubic or hexagonal phase with high viscosity.
Figure 7. Changes of appearance of the SPTS@hard capsules (1: GMO-Gelucire 39/01 = 80:15, 2: GMO-PEG 1000-Tween 80 = 80:15:2.5, 3: GMO-DOXY-Gelucire 39/01 = 80:5:15, 4: GMO-MLX-PEG 1000-Tween 80 = 80:2.5:15:2.5) in pH 1.2 HCl (A) and water (B) (Time intervals: 0, 0.5, 5, 60, 120 min and 24 h respectively).
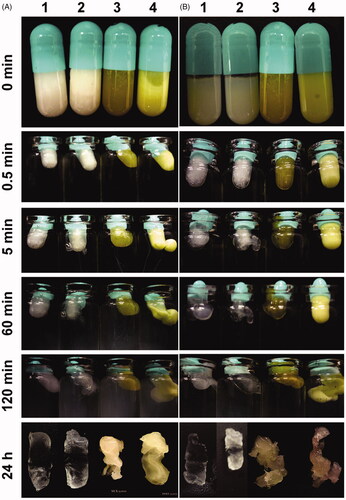
Texture of PLM images of DOXY SPTS at 1 h () showed an atypical filiform, indicating intermediate form of lamellar phase and other crystal phase. Texture at 24 h () was an anomaly hexagonal pyramid, indicating intermediate form of hexagonal phase and other crystal phase. The SAXS diffractogram of DOXY SPTS showed single Bragg diffraction peak at 1 h (), supporting the lamellar phase of DOXY SPTS at 1 h. Three Bragg diffraction peaks (q1 = 0.1128, q2 = 0.1946, q3 = 0.2240) at 24 h (), was in accordance with hexagonal phase (q1: q2: q3 = 1: √3: 2). The phase transformation was a dynamic process. In our previous study, GMO-DOXY system could form cubic phase with 30% water uptake (data not show). The weight gain of DOXY SPTS () at 1 h was about 18%, which was not enough to form cubic phase. The lamellar phase of DOXY SPTS contributed its initial burst release. The hydrophobic additive could favor the transformation from lamellar phase into cubic phase and subsequently into reversed hexagonal phase (Kumar et al., Citation2004). Therefore, the initial burst release reduced with the increase proportion of gelucire 39/01.
Figure 8. Polarizing microscope photos and SAXS diffractograms of optimized SPTS@hard capsules containing DOXY and MLX after 1 h and 24 h release studies. (Polarizing microscope photos: A, DOXY 1 h; B, DOXY, 24 h; C, MLX 1 h; D, MLX 24 h; SAXS diffractograms: E, DOXY 1 h; F, DOXY, 24 h; G, MLX 1 h; H, MLX 24 h).
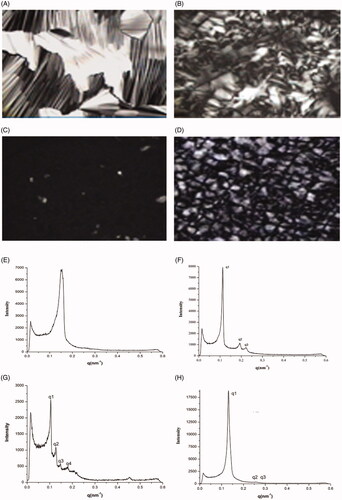
For MLX SPTS, the texture of PLM images at 1 h () presented a dark view, which indicated a cubic phase. Texture at 24 h () was hexagonal pyramid, indicating it was in hexagonal phase. The results of SAXS were in accordance with those of PLM. Four Bragg diffraction peaks (q1 = 0.9993, q2 = 0.1215, q3 = 0.1406, q4 = 0.1716, q1: q2: q3: q4 = √2: √3: √4: √6) at 1 h () and three Bragg diffraction peaks (q1 = 0.1399, q2 = 0.2415, q3 = 0.2788, q1: q2: q3 = 1: √3: 2) at 24 h (), which showed typical Pn3m cubic phase and hexagonal phase, respectively. The SPTS that triggered by water and temperature was successfully established.
Conclusion
LLC-based SPTS@hard capsules were developed to conquer the barriers of LLC in application in oral administration. The phase transformation of SPTSs could be triggered by water as well as temperature. The phase transformation from semisolid state to liquid state and from lamellar phase into cubic phase or hexagonal phase enabled their encapsulation into hard capsules and provided a 24 h sustained release profiles. Appropriate hydrophilic and hydrophobic additives were the key factors to adjust the MP and release profiles of SPTSs. SPTS@hard capsules based on LLC revealed potential for the industrialization of its oral administration on account of its accommodation to drugs with different solubility, controllable release profile and simple preparation process.
Supplemental Material
Download ()Disclosure statement
Xuejuan Zhang, Yujun Xiao, Zhengwei Huang, Jintian Chen, Yingtong Cui, Boyi Niu, Ying Huang, Xin Pan and Chuanbin Wu declared no conflicts of interest.
Additional information
Funding
References
- Abd-Allah H, Kamel AO, Sammour OA. (2016). Injectable long acting chitosan/tripolyphosphate microspheres for the intra-articular delivery of lornoxicam: optimization and in vivo evaluation. Carbohydr Polym 149:263–73.
- Bashiri-Shahroodi A, Nassab PR, Szabó-Révész P, Rajkó R. (2008). Preparation of a solid dispersion by a dropping method to improve the rate of dissolution of meloxicam. Drug Dev Ind Pharm 34:781–8.
- Chaturvedi M, Kumar M, Pathak K, et al. (2017). Surface solid dispersion and solid dispersion of meloxicam: comparison and product development. Adv Pharm Bull 7:569–77.
- Conn CE, Drummond CJ. (2013). Nanostructured bicontinuous cubic lipid self-assembly materials as matrices for protein encapsulation. Soft Matter 9:3449–64.
- Hua T, Zhang X, Tang B, et al. (2018). Tween-20 transiently changes the surface morphology of PK-15 cells and improves PCV2 infection. BMC Vet Res 14:138.
- Huang J, Peng T, Li Y, et al. (2017). Ocular cubosome drug delivery system for timolol maleate: preparation, characterization, cytotoxicity, ex vivo, and in vivo evaluation. AAPS PharmSciTech 18:2919–26.
- Huang Y, Gui S. (2018). Factors affecting the structure of lyotropic liquid crystals and the correlation between structure and drug diffusion. Rsc Adv 8:6978–87.
- Iqbal H, Ali M, Zeeshan R, et al. (2017). Chitosan/hydroxyapatite (HA)/hydroxypropylmethyl cellulose (HPMC) spongy scaffolds-synthesis and evaluation as potential alveolar bone substitutes. Colloid Surfaces B 160:553–63.
- Jie H, Liu L, Shuangying G, et al. (2019). A novel phytantriol-based in situ liquid crystal gel for vaginal delivery. AAPS PharmSciTech 20:185.
- Khampieng T, Wnek GE, Supaphol P. (2014). Electrospun DOXY-h loaded-poly(acrylic acid) nanofiber mats: in vitro drug release and antibacterial properties investigation. J Biomater Sci-Polym Ed 25:1292–305.
- Kulkarni S, Thareja P. (2016). Rheology of colloidal particles in lyotropic hexagonal liquid crystals: the role of particle loading, shape, and phase transition kinetics. Rheol Acta 55:23–36.
- Kumar MK, Shah MH, Ketkar A, et al. (2004). Effect of drug solubility and different excipients on floating behaviour and release from glyceryl monooleate matrices. Int J Pharm 272:151–60.
- Kumar SGV, Mishra DN. (2006). Preparation, characterization and in vitro dissolution studies of solid dispersion of meloxicam with PEG 6000. Yakugaku Zasshi - J Pharm Soc Japan 126:657–64.
- Kumari S, Bargel H, Anby MU, et al. (2018). Recombinant spider silk hydrogels for sustained release of biologicals. ACS Biomate Sci Eng 4:1750–9.
- Lampis S, Carboni M, Steri D, et al. (2018). Lipid based liquid-crystalline stabilized formulations for the sustained release of bioactive hydrophilic molecules. Colloid Surfaces B 168:35–42.
- Landh T. (1994). Phase behavior in the system pine needle oil monoglycerides-poloxamer 407-water at 20. degree. J Phys Chem 98:8453–67.
- Legendre AO, Silva LRR, Silva DM, et al. (2012). Solid state chemistry of the antibiotic doxycycline: structure of the neutral monohydrate and insights into its poor water solubility. Crystengcomm 14:2532–40.
- Leung SSW, Leal C. (2019). The stabilization of primitive bicontinuous cubic phases with tunable swelling over a wide composition range. Soft Matter 15:1269–77.
- Li L, Shao C, Lin TF, et al. (2014). Kinetics of cell inactivation, toxin release, and degradation during permanganation of Microcystis aeruginosa. Environ Sci Technol 48:2885–92.
- Mei L, Xie Y, Huang X, et al. (2017a). An injectable in situ gel with cubic and hexagonal nanostructures for local treatment of chronic periodontitis. Drug Deliv 24:1148–58.
- Mei L, Xie Y, Huang Y, et al. (2017b). Injectable in situ forming gel based on lyotropic liquid crystal for persistent postoperative analgesia. Acta Biomater. 67:99–110.
- Mei L, Xie Y, Jing H, et al. (2017c). A novel design for stable self-assembly cubosome precursor-microparticles enhancing dissolution of insoluble drugs. Drug Dev Ind Pharm 43:1239–43.
- Mulet X, Boyd BJ, Drummond CJ. (2013). Advances in drug delivery and medical imaging using colloidal lyotropic liquid crystalline dispersions. J Colloid Interf Sci 393:1–20.
- Musa MN, David SR, Zulkipli IN, et al. (2017). Development and evaluation of exemestane-loaded lyotropic liquid crystalline gel formulations. Bioimpacts 7:227–39.
- Nardin I, Köllner S. (2019). Successful development of oral SEDDS: Screening of excipients from the industrial point of view. Adv Drug Delivery Rev 142:128–40.
- Nazaruk E, Majkowska-Pilip A, Godlewska M, et al. (2018). Electrochemical and biological characterization of lyotropic liquid crystalline phases – retardation of drug release from hexagonal mesophases. J Electroanal Chem 813:208–15.
- Owens GJ, Singh RK, Foroutan F, et al. (2016). Sol–gel based materials for biomedical applications. Prog Mater Sci 77:1–79.
- Pan X, Han K, Peng X, et al. (2013). Nanostructured cubosomes as advanced drug delivery system. Curr Pharm Design 19:6290–97.
- Peng X, Zhou Y, Han K, et al. (2015). Characterization of cubosomes as a targeted and sustained transdermal delivery system for capsaicin. Drug Des Devel Ther 9:4209–18.
- Pokharkar V, Patil V, Mandpe L. (2015). Engineering of polymer-surfactant nanoparticles of doxycycline hydrochloride for ocular drug delivery. Drug Deliv 22:955–68.
- Qian Z, Yue X, Yi S, et al. (2015). Unique lamellar lyotropic liquid crystal phases of nonionic phytosterol ethoxylates in glycerol. Rsc Adv 5:101393–400.
- Qin L, Mei L, Shan Z, et al. (2016). Phytantriol based liquid crystal provide sustained release of anticancer drug as a novel embolic agent. Drug Dev Ind Pharm 42:307–16.
- Rajabalaya R, Musa MN, Kifli N, David SR. (2017). Oral and transdermal drug delivery systems: role of lipid-based lyotropic liquid crystals. Dddt 11:393–406.
- Rizwan S, Boyd B. (2015). Cubosomes: structure, preparation and use as an antigen delivery system. In: Foged C, Rades T, Perrie Y, Hook S, eds. Subunit vaccine delivery. Advances in delivery science and technology. New York: Springer, 125–40.
- Shah MH, Paradkar A. (2005). Cubic liquid crystalline glyceryl monooleate matrices for oral delivery of enzyme. Int J Pharm 294:161–71.
- Souza C, Watanabe E, Borgheti‐Cardoso LN, et al. (2014). Mucoadhesive system formed by liquid crystals for buccal administration of poly (hexamethylene biguanide) hydrochloride. J Pharm Sci 103:3914–23.
- Thakur RR, McMillan HL, Jones DS. (2014). Solvent induced phase inversion-based in situ forming controlled release drug delivery implants. J Control Release 176:8–23.
- Venkatesh B, Indira S, Size PSF, et al. (2014). Formulation and evaluation of glimepiride oral capsules. Int J Pharm Sci Inv 3:1–13.
- Wan T, Xu T, Pan J, et al. (2015). Microemulsion based gel for topical dermal delivery of pseudolaric acid B: in vitro and in vivo evaluation. Int J Pharm 493:111–20.
- Wang D, Feng L, Song B, et al. (2019). Viscoelastic lyotropic liquid crystals formed in a bio-based trimeric surfactant system. Soft Matter 15:4208–14.
- Yamada S. (2015). Surfactant assemblies (micelles, vesicles, emulsions, films, etc.), an overview. In: Kobayashi S, Müllen K, eds. Encyclopedia of Polymeric Nanomaterials. Berlin, Heidelberg: Springer Berlin Heidelberg, 2431–6.
- Zheng T, Huang X, Chen J, et al. (2018). A liquid crystalline precursor incorporating chlorhexidine acetate and sliver nanoparticles for root canal disinfection. Biomater Sci. 6:596–603.
- Zhou Y, Wang Q, Wang Y, et al. (2014). Biological artificial fluid-induced non-lamellar phases in glyceryl monooleate: the kinetics pathway and its digestive process by bile salts. Drug Dev Ind Pharm. 40:178–85.