Abstract
Chemotherapy drugs have been used for a long time in the treatment of cancer, but serious side effects are caused by the inability of the drug to be solely delivered to the tumor when treating cancer with chemotherapy. Natural products have attracted more and more attention due to the antitumor effect in multiple ways, abundant resources and less side effects. Therefore, the combination of natural active ingredients and chemotherapy drugs may be an effective antitumor strategy, which can inhibit the growth of tumor and multidrug resistance, reduce side effects of chemotherapy drugs. Nano-drug co-delivery system (NDCDS) can play an important role in the combination of natural active ingredients and chemotherapy drugs. This review provides a comprehensive summary of the research status and application prospect of nano-delivery strategies for the combination of natural active ingredients and chemotherapy drugs, aiming to provide a basis for the development of anti-tumor drugs.
1. Introduction
Cancer is a major public health problem worldwide (Siegel et al., Citation2021). In nearly 100 countries around the world, regardless of the level of development, cancer is one of the highly prevalent malignant diseases which is a major cause of morbidity and mortality. Cancer will become the leading cause of death in every country in the 21st century and the most important obstacle to extending life expectancy (Sarisozen et al., Citation2016; Bray et al., Citation2018). The traditional methods of cancer treatment include surgical resection, chemotherapy and radiation therapy. Immunotherapy (Fu et al., Citation2022) and photothermal therapy (Dai et al., Citation2022) have also emerged in recent years.
Chemotherapeutics, also known as cytotoxic drugs, have been used in antitumor therapy since the 1940s. They played an important role in tumor treatment. The mechanism of chemotherapeutics is complex, including affecting the chemical structure of DNA, inhibiting nucleic acid synthesis, acting on nucleic acid transcription and DNA replication and interfering with mitotic tubulin synthesis (Xu et al., Citation2019a). However, the target of chemotherapy drugs is also very important for normal cells, which can cause inevitable damage to the body during chemotherapy, such as hair loss and gastrointestinal toxicity. Therefore, combination, synergistic chemotherapy is a common strategy, and has been recommended for tumor treatment due to its promoted therapeutic effect and reduced systemic toxicity (Wan et al., Citation2019; Maleki et al., Citation2021; Liu et al., Citation2021b). Nevertheless, co-administration therapy may also have additive or synergistic effects resulted from interaction with several distinct targets at reduced administrated doses (Eftekhari et al., Citation2019). At present, there have been studies on nano-drug delivery system (NDDS) for the co-delivery of chemotherapy drugs with photosensitizers (Xiao et al., Citation2021; Zhang et al., Citation2021b), and natural active ingredients.
In recent years, natural products have become the top priority of antitumor drug research and development due to their clear antitumor effectiveness and richness of candidate resources (Yin et al., Citation2019). Natural drugs are safe and have little side effects (Liu et al., Citation2020b), which can enhance immunity and improve chemotherapy sensitivity. More attractively, the synergistic combination therapy with natural chemotherapy sensitizers is becoming a promising strategy for conquering multidrug resistance and reducing the side effects of chemotherapy drugs (Wang et al., Citation2015a). Therefore, the combination of natural active ingredients and chemotherapy drugs may be an effective antitumor strategy. Co-delivery of mutiple drugs via the same vehicle may improve the chemotherapy of tumors by synchronizing their exposure to the drugs and achieving synergistic pharmacological action in the tumor cells (Wan et al., Citation2019). Additionally, NDDS usually have good biocompatibility (Sun et al., Citation2021b), low side effects (Zhu et al., Citation2019), targeting (Lan et al., Citation2021b), controlled release characteristics (Chen et al., Citation2020a), which have brought promising prospect in cancer therapies due to their uniquely appealing properties (Maleki et al., Citation2021; Liu et al., Citation2021b). To date, advancements in nanotechnology provide more significant improvements and valuable information for drug co-delivery systems (Zhang et al., Citation2021a), including nanoparticles, liposomes, polymer micelles, polymer drug conjugates (Majidinia et al., Citation2020). More importantly, the antitumor drug delivery system based on nanocarriers clearly shows the potential to overcome the problems related to traditional chemotherapy (Sohail et al., Citation2021). In recent years, with the continuous development of nano drug carriers, some of them have been tested in clinical trials or used for disease diagnosis and treatment (Zang et al., Citation2021). Nano drug co-delivery system (NDCDS), which loads at least two anticancer drugs with different physicochemical and pharmacological properties into a delivery system, is designed for the purpose of clinical combination chemotherapy (Qi et al., Citation2017). In this paper, the research status and application characteristics of NDCDS of natural active ingredients combined with chemotherapy drugs are reviewed and analyzed, which aim to provide a basis for the research and development of natural active ingredients and chemotherapy drugs for cancer treatment.
2. The effect of natural active ingredients combined with chemotherapy drugs
Studies have confirmed that the combination of natural active ingredients and chemotherapy drugs exert a synergistic antitumor effect through a variety of mechanism. Besides direct antitumor effect, natural active ingredients also can inhibit tumor multidrug resistance (MDR), decrease side effects of chemotherapy drugs, and modulate immune function.
2.1. Induce tumor cell apoptosis and inhibit tumor cell proliferation
Some natural active ingredients, such as schisandrin B (Sch B), β-elemene (β-ELE), betulinic acid (BA), quercetin (Que) and curcumin (CUR), can directly exert antitumor effect through inducing tumor cell apoptosis and inhibiting tumor cell proliferation when combined with chemotherapy drugs. Sch B could inhibit the invasion and metastasis of lung cancer cells by inhibiting vascular endothelial growth factor. At the same time, it could also enhance the cytotoxicity of doxorubicin (DOX) and further promote cell apoptosis (Cai et al., Citation2020). β-ELE could inhibit cell proliferation, arrest cell cycle and induce apoptosis (Zhai et al., Citation2019). BA had an effective antitumor effect on paclitaxel (PTX)-resistant human lung cancer cells (H460) through G2/M cell cycle arrest and induced mitochondrial apoptosis (Zhan et al., Citation2018). BA may also inhibit the proliferation, migration, invasion and tumorigenesis of pancreatic cancer cells by activating AMPK signal, and it combined with gemcitabine (GEM) had an antitumor effect on pancreatic cancer cells (Sun et al., Citation2019). Que combined with PTX significantly inhibited cell proliferation and increased cell apoptosis, blocked cell cycle at G2/M phase, inhibited cell migration, induced endoplasmic reticulum stress, and increased reactive oxygen species (ROS) production (Zhang et al., Citation2020b). CUR could improve PTX-induced apoptosis of HPV-positive human cervical cancer cells through NF-κB-p53-caspase-3 pathway, and it combined with PTX may have a better therapeutic effect in the treatment of human cervical cancer (Dang et al., Citation2015). Cheng et al. (Citation2018) found that CUR could improve the antitumor effect of Cisplatin (CDDP). The mechanism was related to ROS, and the content of ROS was positively correlated with the inhibition of cell proliferation. The combined treatment of RES and 5-fluorouracil (5-FU) could enhance the anti-proliferation effect on colorectal cancer cells (HCT116 and DLD1), induce cell cycle arrest and increase apoptosis in S phase, inhibit pAkt and pSTAT3 signal transduction, and reduce telomerase activity (Chung et al., Citation2018). RES could activate TRPM2 channels in DBTRG glioblastoma cells to enhance PTX apoptosis and oxidation by increasing intracellular steady-state ROS levels and mitochondrial dysfunction (Ozturk et al., Citation2019). In addition, β-ELE promoted the anti-proliferation and apoptosis of CDDP in gingival squamous cell carcinoma (GSCC) in vitro and in vivo by inhibiting STAT3 and blocking JAK2-STAT3 signaling pathway (Huang & Yu, Citation2017). β-ELE inhibited the proliferation of bladder cancer cells in vitro through ROS-5’AMP-activated protein kinase (AMPK) signaling pathway and enhanced CDDP-induced mitochondrial-dependent apoptosis (Gan et al., Citation2020). Rhein and DOX played a synergistic antitumor effect by reducing mitochondrial energy metabolism in hepatocellular carcinoma cells (Wu et al., Citation2020a). BCL combined with DTX inhibited tumor growth, increased cell apoptosis, and reduced tumor angiogenesis in vivo, and enhanced the antitumor effect of DTX on non-small cell lung cancer (NSCLC) in a β-catenin-dependent manner (Lu et al., Citation2020a). Oridonin and DOX presented a synergistic cytotoxic effect in osteosarcoma cells. Oridonin increased the accumulation of intracellular DOX and the rate of apoptosis (Kazantseva et al., Citation2022). Compared with brusatol (BR) or CDDP alone, CDDP and BR could exert synergistic anti-tumor effect by increasing the release of cytochrome c in CT-26 cells, decreasing the expression of caspase-3 and caspase-9, and increasing the ratio of the B-cell lymphoma 2 (Bcl-2)-associated X protein/Bcl-2 (Chen et al., Citation2018). CDDP and triptolide (TPL) combination treatment could induce apoptosis by increasing the expression of caspase-3, 8 and 9, PARP and cytochrome c (Ho et al., Citation2015).
2.2. Inhibit tumor multidrug resistance
Tumor multidrug resistance (MDR) refers to the phenomenon that tumor cells are resistant to a series of chemotherapy drugs with different structures and mechanisms when they are resistant to a kind of chemotherapeutic drug, which is an important reason for the failure of chemotherapy in clinic (Kunjachan et al., Citation2013; Cao et al., Citation2018). The mechanisms of MDR include elevated metabolism of xenobiotics, enhanced efflux of drugs, growth factors, increased DNA repair capacity, and genetic factors (gene mutations, amplifications, and epigenetic alterations) (Bukowski et al., Citation2020). Several factors could be associated with drug resistance in cancer such as overexpression of P-glycoprotein (P-gp), cancer stem cells (CSCs), defect in apoptosis, mutation and alteration in DNA repair pathways, angiogenesis, autophagy, and modulation in metabolic enzymes (Mohammad et al., Citation2020). One of the advantages of co-loading natural active ingredients with chemotherapy drugs is to reverse MDR. Many natural components, such as resveratrol (RES) (Zhang et al., Citation2016b), tetrandrine (TET) (Liao et al., Citation2019; Li et al., Citation2020e), epigallocatechin gallate (EGCG) (Cheng et al., Citation2016), pachymaric acid and dehydrotudouic acid (PT) (Li et al., Citation2020f), quinine hydrochloride (QN) (Shen & Qiu, Citation2017), β-ELE (Zhang & Guo, Citation2021), ferulic acid (FA) (Muthusamy et al., Citation2016), naringin (Jabri et al., Citation2019), baicalein (BCL) (Li et al., Citation2018b), Que (Lu et al., Citation2020b) and Sch B (Wang et al., Citation2017), could inhibit MDR by inhibiting the ABC transport, including P-gp, BCRP, ABCB1, etc. In addition, natural components can also inhibit MDR effects by inhibiting epithelial-mesenchymal transition through other pathways, such as RES (Buhrmann et al., Citation2015; Xu et al., Citation2017b), EGCG (Yuan et al., Citation2017) and BCL (Yu et al., Citation2017). At the same time, some natural components can inhibit MDR effects by acting on genetic factors, such as Que (Sang et al., Citation2014), CUR (Lu et al., Citation2017a; Xu et al., Citation2020), RES (Vinod et al., Citation2015), cinnamaldehyde (CA) (Abbasi et al., Citation2020) and chrysin (Lee et al., Citation2021). Rhein could increase the accumulation of DOX in SMMC-7721/DOX cells by inhibiting energy metabolism and inducing the opening of mitochondrial permeability transition pore (mPTP), and reverse the drug resistance of SMMC-7721/DOX cells (Wu et al., Citation2019a). A summary of the mechanism of reversing MDR of chemotherapy drugs by different natural active ingredients is shown in .
Table 1. Mechanism of natural active ingredients reversing MDR of chemotherapy drugs.
2.3. Decrease side effects of chemotherapy drugs
Chemotherapy drugs can also damage normal tissues or cells of the body while treating tumors, resulting in toxic and side effects. Natural active ingredients combined with chemotherapy drugs can affect on tumor tissues from multiple targets and pathways, and reduce its toxicity by reducing the dosage of chemotherapy drugs. Zeng et al. (Citation2019) found that β-ELE could replace part of cabazitaxel (CBX) and reduce the dosage of CBX, thereby reducing toxicity. Jiang et al. (Citation2016) found that the antitumor activity of CUR combined with etoposide (ETP) was higher than that of CUR and ETP alone, and the dosage was reduced.
Some natural active ingredients can also directly decrease the side effects of chemotherapy drugs, improve the safety of clinical medication. Many natural active ingredients, such as berberine (BER) (Coelho et al., Citation2017; Wu et al., Citation2019b), EGCG (Cheng et al., Citation2016), honokiol (Huang et al., Citation2017; Pillai et al., Citation2017; Huang et al., Citation2020), RES (Gu et al., Citation2018), glycyrrhizin (GL) () (Lv et al., Citation2020), Que (Chen et al., Citation2019) and Sch B (Thandavarayan et al., Citation2015; Cai et al., Citation2020), could reduce DOX-induced cardiotoxicity. In addition, BER (Wang et al., Citation2021) could reduce irinotecan-induced gastrointestinal toxicity. RES could reduce PTX-induced neuropathic pain (Li et al., Citation2019c). Chrysin could reduce methotrexate (MTX)-induced Hepatotoxicity (Ali et al., Citation2014). Angelica polysaccharide (ASP) could protect bone marrow stromal cells from 5-FU chemotherapy damage (Xiao et al., Citation2017). CUR could improve CDDP-induced spatial learning and memory impairment (Oz et al., Citation2015) and nerve oxidative damage (Ozkaya & Naziroglu, Citation2020). CUR (Soetikno et al., Citation2019) and oleanolic acid (OA) (Potocnjak et al., Citation2019) could reduce CDDP-induced nephrotoxicity. A summary of the mechanism of natural active ingredients reducing side effects of chemotherapy drugs is shown in .
Figure 1. Schematic of GL attenuating DOX-induced cardiotoxicity. Reprinted with permission from Lv et al. (2020).
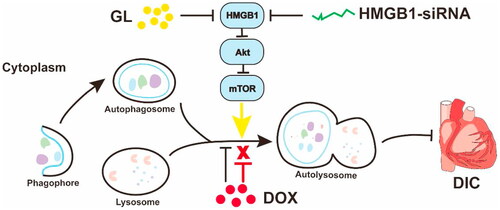
Table 2. Mechanism of natural active components reducing side effects of chemotherapy drugs.
2.4. Immunomodulating activity
The immune function of the body has a great influence on the occurrence and development of tumors. Tumor immunotherapy can achieve the purpose of identifying and killing tumor cells by regulating the immune ability of patients, and minimize the toxicity (Zhang et al., Citation2016c).
The study has shown that natural polysaccharides can activate T lymphocytes, B lymphocytes, macrophages and other immune cells to exert their immune activities (Chen & Huang, Citation2018). ASP could restore the balance of Th1/Th2 immune response, improve tumor microenvironment immunosuppression and enhance antitumor immune function, resulting in synergistic antitumor effect with DOX (Wang et al., Citation2020). Astragalus polysaccharides (APS) could exert anti-tumor effects by restoring immune organs, regulating cellular immune response and increasing the levels of cytokines IL-2, TNF-α and IFN-γ. In addition, APS combined with 5-FU could improve the anti-tumor effect accompanied by the immunosuppressive alleviation of 5-FU on immune system, which may be suitable as an immune adjuvant for chemotherapy (Li et al., Citation2020c). Que could reshape the tumor microenvironment (TM) by reducing the expression of collagen, and promote the penetration of DOX into deep tumor tissues, so as to obtain better antitumor effect (Li et al., Citation2019b). Quagliariello et al. (Citation2017) found that the combination of rapamycin (RAP) and Que could significantly reduce the levels of IL-8, IL-6 and IL-19 cytokines, suggesting that the combination could regulate the immune state of the body and enhance tumor immunity. Guo et al. (Citation2016) found that andrographolide combined with bleomycin could reduce the levels of IL-1β, TNF-α, IL-6 and TGF-β1 cytokines, regulate the immune state of tumor cells, indicating that andrographolide can be used as an adjuvant drug for bleomycin.
3. Advantages of NDCDS of natural drug active ingredients and chemotherapy drugs
3.1. Increase the capacity of targeting delivery of drugs to tumors
Targeted therapy in cancer is the primary role of NDCDS of natural drug active ingredients and chemotherapy drugs. The efficiency of nano-drug targeting tumor depends on the physical and chemical properties of nanocarriers (such as size and surface chemical properties) and the pathophysiological characteristics of target tissues. At present, the clinical application of nano-drugs mostly relies on passive targeting (Arranja et al., Citation2017). Additionally, nanocarriers can be modified by surface modification, and target molecules can be combined with receptors with high expression on the surface of tumor cells to achieve the purpose of active targeting delivery of drugs. Common targeted modification molecules include folate (FT) (Hu et al., Citation2015), hyaluronic acid (HA) (Zhang et al., Citation2019a), lactoferrin (Lf) (Fang et al., Citation2014), polypeptide (Yan et al., Citation2017; Zan et al., Citation2019; Deng et al., Citation2020), wheat germ lectin (Wang et al., Citation2019c), fucoidan (Chu et al., Citation2019), folic acid (FA) (Rawal et al., Citation2020a), prostate-specific membrane antigen targeted ligand (Sun et al., Citation2021a), rituximab (Varshosaz et al., Citation2021), etc.
Guo et al. developed star-shaped polyester-based folic acid-modified nanoparticles (DOX + CUR-FA-NPs) to co-deliver DOX and CUR to enhance tumor targeting selectivity. In vitro and in vivo experiments showed (DOX + CUR)-FA-NPs not only had remarkable tumor targeting and anticancer efficacy, but also had less side effects on normal tissues (Guo et al., Citation2021). Deng et al. (Citation2020) developed a pH-responsive targeting nanosystem modified with T7 peptide, which co-loaded DTX and CUR for the treatment of esophageal cancer. This T7 peptide-modified targeting nanosystem released loaded drugs in the acidic microenvironment of tumor and played a synergistic antitumor effect. Wang et al. (Citation2016) prepared arginine-glycine aspartic acid (RGD) modified lipid-coated PLGA nanoparticles (RGD-SRF-Que NPs) for targeted delivery of sorafenib (SRF) and Que to treat liver cancer. The results showed that the efficacy of SRF combined with Que preparations in both the NPs group and the solution group were better than that of a single drug preparation. And RGD-SRF-Que NPs had a more significant tumor growth inhibitory effect than non-RGD modified SRF-Que NPs. Chu et al. (Citation2019) used dual nanosystems to jointly deliver EGCG and CUR. A dual targeting system was established by using HA and fucoidan as targeting agents for CD44 on prostate cancer cells and P-selectin in tumor vascular system, respectively. It was found that compared with EGCG/CUR combined solution, EGCG/CUR loaded nanoparticles were more absorbed into prostate cancer cells, resulting in better antitumor efficiency.
3.2. Maintain the optimal proportion of combined drug administration
In combination antitumor therapy, the optimal proportion of drugs has a great influence on the antitumor effect. The inappropriate proportion of drugs may cause antagonism of combined drug administration and reduce the therapeutic effect. NDCDS can change the original pharmacokinetic characteristics of drugs, so as to ensure that the combined drugs enter tumor cells with a constant proportion, which is conducive to the synergy between drugs.
At present, in order to maintain the optimal ratio of combined drug administration, the optimal ratio of the two drugs was first screened through in vitro cytotoxicity experiments and calculation of the combination index. Then the nano-formulations are prepared according to the proportions. Finally, in vitro release and in vivo pharmacokinetic studies were used to verify whether the optimal proportion was maintained in the nano-formulations. Jia et al. (Citation2015) prepared multifunctional mesoporous silica nanoparticles (MSN) co-delivery of PTX and TET. When the molar ratio of PTX/TET was 4.4:1, the drug resistance of MCF-7/ADR cells to PTX was completely reversed, and it was more effective than PTX-CTAB @ MSN or free PTX in inhibiting the growth of tumor cells. Chen et al. (Citation2014) developed PLGA nanoparticles co-delivery of VCR and verapamil (VRP). In multidrug resistant MCF-7/ADR cells, the 1:250 molar ratio of VCR/VRP showed a strong synergistic effect, which could enhance the efficacy of multidrug resistant breast cancer and reduce the toxicity to normal cells. Chen et al. (Citation2017) prepared bovine serum albumin (BSA) coated supermagnetic iron oxide nanoparticles (SPIOs) by co-precipitation method. CUR and sunitinib (Sun) were co-loaded in BSA-SPIOs (denoted as SPIO-SC) to achieve synergistic treatment. The study showed that the proportion range of the initial designed optimal concentration of CUR and Sun was maintained at the tumor target site (Sun:CUR = 0.5–0.25), resulting in the optimal synergistic effect and more effective treatment results. Rawal et al. (Citation2020a) developed FA modified NLCs to promote the targeting and proportional co-delivery of DTX and CUR, namely FA-DTCR-NLCs. In vivo pharmacokinetic study, DTX and CUR showed a synergistic ratio of 1:2 throughout the cycle. The in vivo toxicity evaluation of FA-DTCR-NLCs showed that DTX-related side effects were significantly reduced. The results of preclinical studies in vitro and in vivo proved the excellent therapeutic and safety of FA-DTCR-NLCs in the treatment of NSCLC.
Liposomes can also be used to maintain the required proportion of synergistic drugs and coordinate the release of co-delivery drugs to achieve enhanced antitumor activity in vivo (Shen & Qiu, Citation2017). Cheng et al. (Citation2018) prepared CUR and CDDP co-loaded liposomes (CDDP/CUR-Lip) for the treatment of hepatocellular carcinoma. MTT method and median effect method were used to determine the optimal synergistic drug loading ratio when the molar ratio of CDDP to CUR was 1:8. In vitro release experiments showed that CDDP and CUR had similar release rates within 24 h, indicating that the ratio of the two drugs can remain stable to ensure the optimal synergistic ratio at all time points. In vivo pharmacokinetic studies also showed that CDDP/CUR-Lip in plasma at different time points could maintain a synergistic drug ratio of 1:8. Zucker and Barenholz (Citation2010) prepared topotecan and VCR-loaded liposomes (LipoViTo). Pharmacokinetics and biodistribution studies showed that LipoViTo simultaneously delivered two drugs to tumors and released them in a predetermined proportion. LipoViTo were more effective in two mouse tumor models than free drugs and liposomes alone or in combination.
3.3. Control the sequence of drug release
The multi-stage NDDS can control the sequence and process of drug combination therapy by regulating the release response mechanism and release rate of combined drugs under different stimuli, so as to achieve more accurate drug delivery process and improve the effect and specificity of combination. Lin et al. (Citation2019) designed a pH and redox two-stage response nanocarrier for co-delivery of phosphorylated curcumin (p-CUR) and DOX. MSNs nanocarriers () were functionalized by specific cuttable PEGylation and disulfide cross-linked hydrogel coatings: MSNs were encapsulated as core-loaded DOX, p-CUR in hydrogel coatings. In the blood circulation, PEGylated nanocarriers had a longer time. In tumor tissues, polyethylene glycol (PEG) shell was cracked due to its sensitivity to pH, and cationic hydrogel coating was exposed to improve cell uptake. In tumor cells, the hydrogel coating could be lysed and released by glutathione. Thus, the double-response shell endowed the nanocarrier with the cell uptake and specific cancer cell target release triggered by the extracellular pH of tumor cells, and the synergistic effect of p-CUR and Dox enhanced the apoptosis of HeLa cells. In order to kill cancer stem-like cells (CSCs) in tumors, Shen et al. (Citation2021) prepared nanoparticles to co-load all-trans retinoic acid and camptothecin (CPT). All-trans retinoic acid is released under hypoxic conditions, resulting in CSCs differentiation under hypoxic conditions. In differentiated CSCs, ROS levels increased, leading to CPT release and subsequent cell death. This dual strategy could control drug release in stem cells, reduce stem cell-related drug resistance, and enhance chemotherapy response. It could inhibit tumor growth and prevent postoperative tumor recurrence and metastasis in breast tumor mouse models.
Figure 2. Illustration of the dual responsive nanocarriers for co-delivery p-Cur and Dox. Reprinted with permission from Elsevier (Lin et al., 2019).
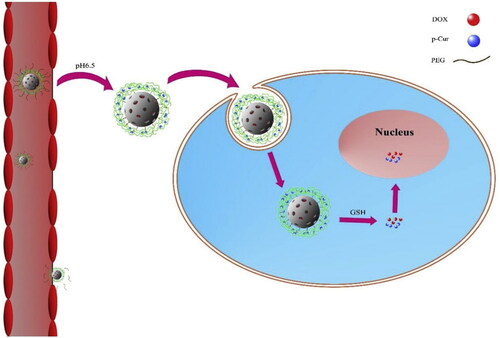
Figure 3. Proposed schematic diagram of AP-PP-DOX (Angelica polysaccharide-peptide-doxorubicin) nanoparticles for antitumor drug delivery. Reprinted with permission from Wang et al. (2020).
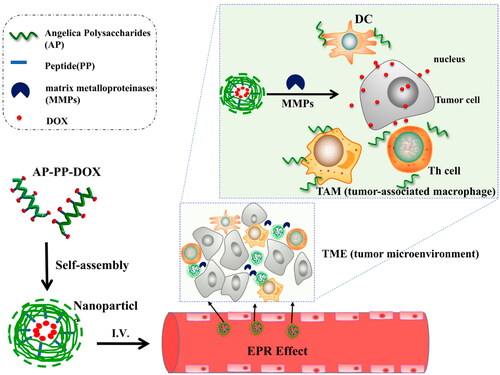
Figure 4. Structure of lactobionic/folate dual-targeted amphiphilic maltodextrin-based micelles for targeted delivery of sulfasalazine and resveratrol. Reprinted with permission from American Chemical Society (Anwar et al., 2018).
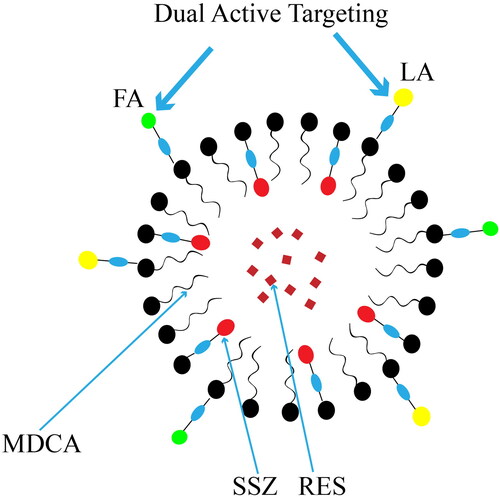
Figure 5. Two strategies for NDCDS of natural active ingredients and chemotherapy drugs: physical encapsulation and carrier-linked prodrug delivery system.
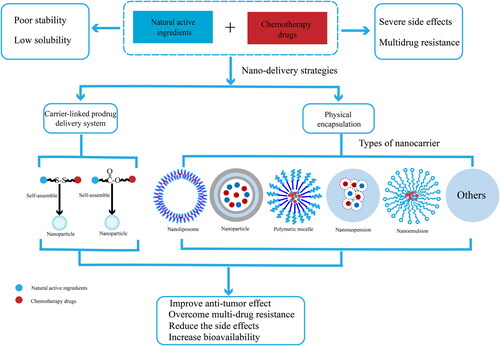
Figure 6. Illustration of the preparation, dual-drugs loading, pH-responsive intracellular release of MSNCA@GODOX-HA nanoparticles and the apoptosis induction in MCF-7 cells. International Journal of Nanomedicine 2020 15 10285-10304’ Originally published by and used with permission from Dove Medical Press Ltd.
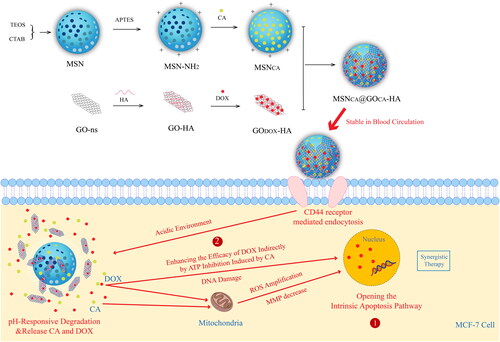
3.4. Used as a drug carrier
Some natural active ingredients have not only the same drug encapsulation ability as synthetic nanomaterials, but also better biodegradability, biocompatibility and safety than synthetic nanomaterials. They can be used as natural drug carrier for nano-drug delivery, which can improve the bioavailability and efficacy of drugs. At present, ASP (Wang et al., Citation2020), bletilla polysaccharide (Wang et al., Citation2019a), Ganoderma lucidum polysaccharide (Zheng et al., Citation2021a), ursodeoxycholic acid (MDCA) (Anwar et al., Citation2018), Semen Armeniacae Amarum protein (Lin et al., Citation2020) have been successfully used for the delivery of DOX, MTX, sulfasalazine and PTX. A summary of the studies of natural active components as drug carriers in NDCDS is shown in .
Table 3. Studies of natural active components as drug carriers in NDDS.
4. Types and characteristics of NDCDS
NDCDS have shown strong advantages in the delivery of natural active ingredients and chemotherapy drugs, including high encapsulation efficiency, prolonging circulation time, controlling release and improving therapeutic effect. At present, there are mainly two strategies for NDCDS of natural active ingredients and chemotherapy drugs (), physical encapsulation and carrier-linked prodrug delivery system, and physical encapsulation include liposomes, nanoparticles, polymer micelles, polymer drug conjugates, nanosuspensions, nanoemulsions, etc.
4.1. Liposomes
Liposomes have the characteristics of nanoscale, biofilm-like structure, good biocompatibility, and relatively stable. Moreover, their surface modification properties make the application of liposomes truly extend to targeted and environmentally sensitive delivery systems (Li et al., Citation2019a). The aqueous phase and lipid bilayer of liposomes can contain a variety of drugs. For example, hydrophilic drugs can be encapsulated in the core of hydrophilicity. Hydrophobic drugs can be encapsulated in the lipid membrane. Amphoteric drugs can be located on the phospholipids in the aqueous phase and membrane. A summary of the liposomes used to co-deliver chemotherapy drugs and natural active ingredients is shown in .
Table 4. The researches of liposomes used to co-deliver chemotherapy drugs and natural active ingredients.
4.1.1. Passive targeting liposomes
The distribution of the passive targeting preparation in the body after it is administered intravenously is determined by the size of the microparticles. Small-size nanoparticles usually have strong tissue permeability and small renal excretion (Sohail et al., Citation2021).
Jiang et al. (Citation2016) prepared the nano-lipid carrier (ETP-CUR-NLC) containing ETP and CUR, which showed low cytotoxicity in normal tissues and high cytotoxicity in tumor tissue in vivo. Meng et al. (Citation2016a) co-encapsulated Res and PTX in PEGylated liposomes, which could produce strong cytotoxicity to drug-resistant MCF-7/ADR tumor cells in vitro, and improve the bioavailability of drugs and tumor retention ability in vivo.
4.1.2. Active targeting liposomes
Through the modification of the special ligands, liposomes can also achieve active targeting. HA can modify the surface of liposomes to give them targeting. This may be because the specific adhesion of cancer cells to HA ligands is enhanced, and the activity and permeability of HA administration are increased, resulting in the improvement of the efficacy of standard dosage, reduction of side effects, and overcoming drug resistance. Mahira et al. (Citation2019) prepared cationic liposomes of CBX and silibinin (SIL) by ethanol injection method, and performed surface functionalization with anionic HA through electrostatic interaction. The results showed that HA-coated liposomes were more effective, and CBX and SIL showed a synergistic effect on prostate cancer. Liu et al. (Citation2016b) constructed HA modified nanoliposomes (NLCs) as a carrier for co-delivery of BCL and DOX. In vitro cytotoxicity experiments showed that HA-modified NLCs were better than BCL/DOX-NLCs in reducing the activity of breast cancer (MCF-7/ADR) cells, which may be due to the fact that HA ligands on the surface of particles can target HA receptors on the surface of tumor cells, thereby promoting the entry of BCL and DOX into cancer cells. Lu et al. (Citation2017b) prepared the targeted NLCs modified by HA for co-delivery of GEM and BCL precursors by nanoprecipitation technology. In vivo antitumor experiments showed that HA-GEM-BCL NLCs had the strongest antitumor effect on pancreatic cancer animals compared with other liposomes, and had less systemic toxicity to tumor treatment in vivo. At the same time, the cell uptake efficiency of HA-GEM-BCL NLCs in vitro was significantly higher than that of other NLCs.
4.1.3. pH-sensitive liposomes
When liposomes deliver therapeutic drugs in vivo, the change of pH can make some new liposomes release drugs in specific pathological tissues. pH-sensitive liposomes are widely used environmental-sensitive liposomes (Li et al., Citation2019a). Cao et al. (Citation2019a) developed a pH-sensitive nanostructured lipid carrier (DOX/ELE Hyd NLCs) loaded with DOX and β-ELE for the treatment of lung cancer. When NLCs were transported to acidic tumor sites, acid-sensitive hydrazone bonds may decompose and promote drug release. Compared with non pH-sensitive NLCs and single drug-loaded NLCs, pH-sensitive and double drug-loaded NLCs showed higher cytotoxicity and tumor inhibition rate.
4.1.4. Ultra-deformable liposomes
Liposomes can increase the penetration rate of drugs through the skin, but they are limited by their positioning in the stratum corneum during use. Ultra-deformable liposomes composed of phospholipids and edge activators are used to increase the skin penetration of different biologically active substances, which can achieve obvious drug localization even in the bloodstream. Cosco et al. (Citation2015) co-encapsulated RES and 5-FU in ultra-deformable liposomes. Compared with the free drug form and a single encapsulant, the ultra-deformable liposomes co-encapsulated by the two drugs had increased antitumor activity in skin cancer cells. This effect may depend on super-deformable liposomes, which may accumulate in deeper skin layers, thereby creating a skin reservoir from which RES and 5-FU can be gradually released.
4.2. Nanoparticles
4.2.1. Lipid nanoparticles
Lipid nanoparticles have stable properties, simple preparation, and a certain sustained and controlled release effect, which can reduce the toxicity of drug loading. Targeted lipid nanoparticles with specific modifications can actively target the diseased tissues (Tapeinos et al., Citation2017). Li et al. (Citation2020g) established an active targeting drug delivery system (FA-LB-MSNs) for FA modified PEGylated lipid bilayer modified mesoporous silica nanoparticles, which jointly delivered PTX and tanshinone IIA (Tan IIA). The bioadhesion between FA and its receptors significantly increased the uptake of FA-LB-MSNs by NB4 cells. Compared with uncoated MSNs, FA-LB-MSNs showed sustained drug release, and PTX and Tan IIA were released synchronously from the carrier. Gao et al. (Citation2019) prepared MSNs with a layer of polyethylene glycol lipid bilayer (PL) on the surface, which was used to co-load PTX and CUR, and to control the release of loaded drugs from the mesoporous. Cell uptake and localization studies showed that MSNs with a layer of polyethylene glycol lipid bilayer could effectively carry drugs into cancer cells with sustained release characteristics, reduce the clinical dosage of the drug, reduce its side effects, and showed good targeting characteristics for breast cancer.
4.2.2. Active targeting nanoparticles
In order to improve the targeting of nanoparticles, researchers have tried to functionalize their surface to better achieve the purpose of active targeting in recent years. Dong et al. (Citation2020) developed mesoporous silica nanoparticles () coated with graphene oxide modified by HA for the combined administration of CA and DOX to enhance their combined therapeutic effect on tumor cells. CA and DOX co-loaded graphene oxide coated mesoporous silica nanoparticles (MSNCA @ GODOX-HA) actively targeted tumor cells through the “ligand receptor” affinity between HA and CD44 receptors, improved the advantages of CA and DOX, limited their shortcomings, so as to achieve effective treatment of cancer. Li et al. (Citation2017c) prepared Tf-modified DTX and BCL loaded solid lipid nanoparticles (Tf-D/B-SLNs) for lung cancer combined chemotherapy, which had better antitumor efficiency than unmodified SLNs and single drug loaded SLNs. Gao et al. (Citation2021) prepared PLGA NPs encapsulated antitumor drugs DOX and CUR by solvent evaporation method. Then, the extracted TE10 cell membrane and DSPE-PEG were self-assembled to wrap the drug-loaded PLGA NPs, so that the drug carrier had homologous targeting function, significantly improved the drug concentration of the target site. The results showed that PMPNs could be specifically taken up by TE10/DOX cells, and exhibited good antitumor effect in vitro. At the same time, it had excellent targeting and therapeutic effect in TE10/DOX xenograft mice. Wang et al. (Citation2015b) synthesized PTX and BCL prodrugs containing FA and HA dual targeting ligands, and prepared multifunctional self-assembled nanoparticles for delivery of PTX prodrug and BCL prodrug. HA and FA on the surface of the complex could bind to CD44 receptor and folate receptor respectively. The results showed that PTX-BCL nanoparticles exhibited good antitumor activity in a wide range of drug concentration and had obvious synergistic effect. In addition, nanoparticles with folate receptor (Hiremath et al., Citation2019; Guo et al., Citation2021) active targeting and dual targeting (Wang et al., Citation2015b; Cui et al., Citation2016) were also studied.
4.2.3. pH-sensitive nanoparticles
The pH-sensitive polymer nanocarriers are developed by using the acidic microenvironment in tumor tissues and tumor cell/lysosomes to achieve the efficient and rapid release of antitumor drugs tumors. Martinez-Edo et al. (Citation2020) prepared a glycyrrhetinic acid (GA)-modified pH-triggered MSN based nanocarrier for the delivery of DOX/CPT-polyethylene glycol(CPT-PEG). GA modified drug delivery system could be selectively absorbed by HepG2 cells. Under acidic conditions (pH = 4), DOX could be rapidly released and CPT-PEG could be gradually released. The results showed that the presence of this system reduced the systemic toxicity of combined treatment, but still maintained the effective cytotoxicity of liver cancer. Gao et al. (Citation2017a) prepared a new type of pH-sensitive prodrug nanoparticles by synthesizing the self-assembly of amphiphilic macromolecular prodrugs for selective co-delivery of DOX and CUR. Compared with the neutral environment (pH = 7.4), CUR-DOX-NPs could release DOX and CUR more effectively in acidic environments (pH = 5.0). CUR-DOX-NPs were easily absorbed by cells, and selectively released drugs into human breast cancer cell line MCF-7 with significant cytotoxicity. Compared with the free DOX at the same level, CUR-DOX-NPs had lower cardiac toxicity and were safer for zebrafish. Khan et al. (Citation2019b) prepared pH-sensitive CDDP/OA calcium carbonate nanoparticles (LCC NPs) by microemulsion method. In vitro release studies showed that LCC NPs released more drugs at acidic pH than at alkaline pH, and it was pH-sensitive and ideal carrier for chemotherapy drugs. Cell viability test and toxicity evaluation showed that CDDP/OA-LCC NPs could not only reduce CDDP-induced hepatotoxicity, but also effectively treat hepatocellular carcinoma. Zeng et al. employed HA-conjugated CUR and D-α-tocopheryl acid polyethylene glycol succinate as selective drug-carrying carriers to deliver dasatinib to cancer cells. The nanoparticles were pH sensitive and could accelerate drug release at low pH conditions. In vitro and in vivo experiments showed that the nanoparticles had obvious cytotoxicity to HepG2 cells and could inhibit the growth of solid tumors in mice (Zeng et al., Citation2022).
4.2.4. Redox-responsive nanoparticles
In addition to pH-responsive nanoparticles, redox-responsive nanoparticles were also used for the co-delivery of natural active ingredients and chemotherapy drugs. Xue et al. prepared citronellol-cabazitaxel conjugate self-assembled nanoparticles (CSNPs) by conjugating cabazitaxel with citronellol via the disulfide bond that was redox-sensitive to the high concentration of glutathione within tumor cells (Xue et al., Citation2016). CSNPs could rapidly release cabazitaxel in tumor cells. The in vivo pharmacokinetics of CSNPs could be apparently improved, and CSNPs had a target effect for accumulating at the tumor site. Li et al. developed a novel pH and redox dual-sensitive polypeptide-calcium phosphate hybrid nanoparticles (Li et al., Citation2021b). With the disulfide-crosslinked interlayer and the Calcium phosphate (CaP) shell, the polypeptide-calcium phosphate hybrid nanoparticles encapsulated CUR into the hydrophobic core of micelles and loaded DOX on the hydrophilic segment of micelles as well as CaP shell. The premature leakage of drugs from the nanoparticles at physiological pH was efficiently restrained because of the enhanced structure integrity, whereas at acidic and hypoxia microenvironment the release of both drugs was promoted due to the rapid dissolution of the CaP shell and the break of the disulfide crosslinked network.
A summary of the researches of nanoparticles used to co-deliver chemotherapy drugs and natural active ingredients is shown in .
Table 5. The researches of nanoparticles used to co-deliver chemotherapy drugs and natural active ingredients.
4.3. Polymeric micelles
Polymeric micelles are a macromolecular assembly formed by synthetic block copolymer or graft copolymer, which have a two-phase structure of a spherical core and a shell (Yokoyama, Citation2014). Polymer micelles have core-shell structures. Hydrophobic nuclei are commonly used to encapsulate poorly water-soluble or hydrophobic drugs, which can improve the solubility and bioavailability of encapsulated drugs and avoid rapid degradation of drugs in vivo. Hydrophilic shells can prolong circulation time and improve spatial stability by reducing hydrolysis in blood circulation. Polymeric micelles can selectively and effectively accumulate in tumors by enhancing the permeability and retention (EPR) effect, thus improving the therapeutic effect of chemotherapy drugs. A summary of the researches of polymer micelles used to co-deliver chemotherapy drugs and natural active ingredients is shown in .
Table 6. The researches of polymeric micelles used to co-deliver chemotherapy drugs and natural active ingredients.
4.3.1. Passive targeting polymer micelles
Sabzi et al. (Citation2020) synthesized a novel biodegradable polyε-caprolactone-co-maleic anhydride grafted citric acid copolymer micelles (PCL-co-P(MA-g-CA)) for co-delivery of DOX-CUR to eradicate MDA-MB231 cells. The unique micelle structure allowed the simultaneous loading of hydrophilic DOX and hydrophobic CUR, and the loading efficiency of each drug was above 98 %. DOX@CUR loaded micelles showed synergistic effect, and the combined treatment of DOX and CUR nanoparticles enhanced the cytotoxicity of MDA-MB231 cells by promoting apoptosis. Lv et al. (Citation2016b) prepared polyethylene glycol-bock-polylactic acid micelles for co-delivery of DOX and CUR to multidrug-resistant breast cancer (MCF-7/ADR) cells. In vitro experiments showed that (DOX + CUR)-micelles could reduce the DOX efflux rate through the inhibitory effect of CUR on P-gp, significantly increase the uptake of DOX by MCF-7/ADR cells, thereby enhancing the in vitro cytotoxicity of DOX. In vivo experiments showed that compared with (DOX)-micelles, (CUR)-micelles, free (DOX + CUR), free DOX, and free CUR, (DOX + CUR)-micelles had more tumor accumulation and better antitumor effect in drug-resistant MCF-7/ADR xenograft model. Zheng et al. (Citation2021b) developed a polymer nano micelle for co-delivery of TPL and an antitumor chemotherapeutic drug SN38. Amphiphilic SN38 prodrug polymeric micelles (PSN38) and encapsulated the hydrophobic esterase-responsive prodrug of TPL, TPL-naphthalene sulfonamide (TPL-nsa), were synthesized to form PSN38@TPL-nsa nanoparticles. PSN38@TPL-nsa showed strong antitumor effect and reshaped tumor microenvironment in cancer-related fibroblasts-rich peritoneal disseminated tumor and patient-derived xenograft model of gastric cancer.
4.3.2. Active targeting polymer micelles
The polymer micelles are surface modified to achieve the active targeting of tumor sites. Sarisozen et al. (Citation2016) developed PEG-PE-based polymeric micelles modified with single-chain fragment variable region of glucose transporter-1 antibody as ligand for the synergistic delivery of DOX and CUR for the treatment of glioblastoma to promote blood-brain barrier transport and glioblastoma targeting. Sabra et al. (Citation2018) prepared a new type of natural self-assembly micelles with hydrophilic Lf as the micelle corona to co- deliver RAP and wogonin (WOG). Lf corona enhanced tumor targeting and prolonged the cycling of nanocarriers. This combined NDDS maximized the synergistic cytotoxicity of RAP and WOG in tumor inhibition of MCF-7 breast cancer cells and Ehrlich ascites tumor animal models.
4.3.3. pH-sensitive polymeric micelles
Multistage pH-responsive micelles can better control drug release in tumor sites. Yang et al. (Citation2017b) synthesized a pH-sensitive polymer polyethylene glycol-benzoic acidimine-poly(γ-benzyl-L-aspartate)-b-poly (1-vinylimidazole) block copolymer (PPBV), and developed a pH-responsive micelle system () for PTX and CUR co-delivery and synergistic removal of breast cancer stem cells (bCSCs) and non-bCSCs. The results of in vitro release experiments showed that the benzoic acid-imine bond was relatively stable in neutral medium, and the hydrophilic PEG layer prevented the outward diffusion of hydrophobic drugs and limited the drug release. However, in the acidic extracellular environment of tumor cells, the PEG layer gradually separated from the PPBV micelles and the volume of the PPBV micelles was reduced. The EPR effect was used to effectively target breast tumors, thereby promoting their cellular uptake and tumor deep penetration, and triggering the rapid release of PTX and CUR. These advantages were also conducive to the maximum efficacy of the combined treatment of PTX and CUR, achieving superior tumor inhibitory activity and effective bCSCs killing ability in vivo. Qi et al. (Citation2018) developed a novel pH and redox dual-sensitive nanocarrier loaded with CUR and antitumor peptide (AP). Amphiphilic block copolymers were prepared by triphenylphosphine (TPP)/oligomeric hyaluronic acid (oHA)/disulfide-menthone 1,2-glycerolone (SM), referred to as TPP-oHSM. In vitro release and cellular uptake experiments showed that C/A@TM targeted mitochondria and CD44 receptors, and it was sensitive to pH and redox. In addition, C/A@TM showed satisfactory cytotoxicity to MDA-MB-231 cells and MCF-7 cells. Finally, it showed good therapeutic effect in clinical application.
4.3.4. Redox-responsive self-assembled polymer micelles
Self-assembled micelles can adjust the ratio of the two drugs and optimize the synergistic effect of drug combinations by using a simple co-assembly strategy, which is simple and feasible. Xu et al. (Citation2019b) prepared redox-sensitive rod-like micelles by co-assembly of CPT-disulfide bond-PEG2000-4-carboxyphenylboronic acid and CPT-disulfide bond-GEM to achieve controllable redox and tumor-targeted synergistic self-delivery of CPT and GEM. In vitro drug release studies verified the redox triggering and synchronous rapid release of CPT and GEM by the co-assembled nano micelles. Nano micelles had obvious synergistic anti-proliferation effect on MCF-7/ADR and 4T1 cells in vitro. In addition, biodistribution nano micelles preferentially aggregated in the breast tumor site, which could improve the therapeutic effect and reduce the side effects of nonselective antitumor drugs.
4.4. Polymer-drug conjugates
Polymer-drug conjugates are designed to release drugs in tumor tissues or cells. They are mostly designed and manufactured to release drugs in tumor tissues or cells triggered by different stimuli to reduce systemic toxicity of parent drugs and improve their therapeutic effects (Feng & Tong, Citation2016). Polymer-drug conjugates remain stable in the process of transport in the body. Through the reasonable design of the connecting group, the physiological environment such as pH value, enzyme, temperature, and magnetic sensitivity is obtained, so as to realize the effective release of drugs in tumor targeting sites. A summary of the researches of polymer-drug conjugates used to co-deliver chemotherapy drugs and natural active ingredients is shown in .
Table 7. The researches of polymer-drug conjugates used to co-deliver chemotherapy drugs and natural active ingredients.
4.4.1. Passive targeting
Graft copolymers have the characteristics of easy preparation, high drug loading, good stability, and tumor accumulation, which are often used as delivery carriers of antitumor drugs. Tai et al. (Citation2014) designed a new graft copolymer, which can form polymer nanocarriers similar to protein folding for co-delivery of CPT and DOX. CPT was polymerized on the catenary segment of the graft polymer, while DOX was non-covalently wrapped in the hydrophobic core. In vivo studies have shown that compared with free drugs, nanocarriers show strong accumulation in tumor sites, and have significant antitumor activity in lung cancer xenograft mouse models. Huo et al. (Citation2020) successfully prepared a dextran-deoxycholic acid (Dex-DOCA) amphiphilic polymer for co-delivery of PTX and silybin (SB). Dex-DOCA had good encapsulation efficiency for PTX and SB, and the drug loading was adjustable. The release of PTX and SB at a fixed dose ratio was consistent with the initial drug loading ratio of co-donor nanoparticles (PTX:SB = 1:1.4), which was conducive to the generation of synergistic effect. In vivo studies showed that co-loaded nanoparticles could effectively accumulate in the tumor site through passive targeting, and ultimately improve the permeability of nanoparticles to tumors in the A549 xenograft model by enhancing the intratumoral permeability and the sensitization of SB to PTX. Zou et al. (Citation2017) used one-step nanoprecipitation method to co-load PTX and natural compound Borneol (BNL) in the prepared PEG-PAMAM NPs, denoted as PB/NPs. The results showed that PB/NPs and P/NPs + BNL had high cellular uptake and cytotoxicity on A2780/PTX cells, and could improve the apoptosis rate. More importantly, although PB/NPs and P/NPs + BNL showed similar tumor accumulation in tumor-bearing mice, PB/NPs could significantly decrease tumor growth of A2780/PTX tumor-bearing mice, in comparison to P/NPs + BNL.
4.4.2. Active targeting polymer-drug conjugates
When nanoparticles are modified with various ligands, they can specifically target components in the tumor microenvironment, including dendritic cells, macrophages, fibroblasts, tumor vascular system (Yang et al., Citation2021). In recent years, polymer dendrimers have been widely used for targeted delivery of antitumor drugs due to their homogeneity and biocompatibility. Anbazhagan et al. (Citation2021) prepared polyamidoamine (PAMAM) dendrimers co-loaded FA and PTX coupled with arginine-glycine-aspartic acid (RGD) to overcome the multidrug resistance of oral cancer cells overexpressing P-gp. In vitro drug uptake data showed that RGD-PAMAM-FP could deliver more PTX than PAMAM-FP in KB CH-R8-5 cells. This indicated that RGD promoted the accumulation of intracellular PTX through active targeting on multidrug-resistant KBCH-R8-5 cells. In vitro toxicity experiments showed that when FA and PTX were loaded on RGD-PAMAM nanocarriers, more KBCH-R8-5 cell apoptosis might be induced than loaded on PAMAM nanocarriers. Zhang et al. (Citation2017b) prepared iRGD peptide modified lipid-polymer hybrid nano system (LPN) to couple PTX and TET in a 1:1 ratio. PTX was conjugated to the PLGA polymer core through disulfide bonds, and TET was encapsulated in the hydrophobic polymer core. Due to the enhanced TET-mediated cellular uptake and P-gp inhibition, the accumulation of PTX in A2780/PTX cells treated with PTX + TET/iRGD LPNs was significantly higher than that in free drugs or non-iRGD modified LPNs. PTX + TET/iRGD LPNs had the highest cytotoxicity to A2780/PTX cells, especially promoting ROS production, enhancing apoptosis, and cell cycle arrest.
4.5. Nanosuspension
Nanosuspensions are nanoscale, heterogeneous aqueous dispersions of insoluble drug particles stabilized by surfactants (Jacob et al., Citation2020). Nanosuspension technology is the only alternative when many disadvantages of drug molecules such as the inability to form salt, high molecular weight, and dosage hinder the development of appropriate dosage forms. This technique is attractive due to the reduced use of excipients, which usually cause toxicity or side effects. NS made from hydrophobic drugs have the advantages of increasing drug loading, improving solubility, improving bioavailability (Sahu et al., Citation2016), and reducing side effects (Wang et al., Citation2019d).
Wang et al. (Citation2019d) prepared PTX-BA hybrid nanosuspension to induce early apoptosis of MCF-7 cells through the decrease of mitochondrial membrane potential (Jiang et al., Citation2015). Compared with PTX-NS and BA-NS, PTX-BA-NS has the strongest effect on MCF-7 cell apoptosis, which may be partly attributed to the enhanced accumulation of PTX-BA-NS in cells and the synergistic effect of PTX and BA. Sahu et al. (Citation2016) prepared nanosuspension of poorly water-soluble CUR and DTX by precipitation homogenization technology, and modified it with polyethylene glycol to increase its solubility and bioavailability, thereby improving the efficacy. Chen et al. (Citation2015) and Zhao et al. (Citation2015b) chose 10-hydroxycamptothecin (HCPT) and DOX to prepare HD nanoparticles (HD NPs) by “green” and convenient self-assembly method, which successfully improved the water solubility of HCPT. Due to the higher chemical sensitization and improving intracellular drug accumulation induced by DOX/HCPT combination, HD NPs showed synergistic therapeutic effect and enhanced inhibitory effect on breast cancer cells and drug-resistant cancer cells in vitro. Liu et al. (Citation2021a) prepared multifunctional nanosuspension of CUR and irinotecan hydrochloride by precipitation using carrier-free self-assembly strategy, which showed better antitumor effect on gastric cancer cells.
4.6. Nanoemulsion
Nanoemulsions (NM) usually include aqueous and oil phases, which can overcome the shortcomings of low drug solubility by improving bioavailability, increasing drug stability, and reducing side effects when used as drug carriers (Yukuyama et al., Citation2017). Guo et al. (Citation2020a) developed a novel NM as an oral 5-FU and CUR synergistic delivery system (CUR/5-FU-NM) for synergistic treatment of liver cancer. The comprehensive ratio analysis showed that when the molar ratio of CUR:5-FU was 2:1, CUR and 5-FU had the greatest synergistic effect. CUR /5-FU-NM enhanced the solubility and permeability of CUR and 5-FU, and improved the oral bioavailability of CUR and 5-FU, thereby enhancing the anti-hepatocellular carcinoma effect of CUR and 5-FU in vitro and in vivo. Meng et al. (Citation2016b) encapsulated PTX and BCL in nanoemulsion (PTX/BA NM) to treat breast cancer. The experiment showed that the synergistic effect of PTX and BA in combination with the weight ratio of 1:1 was the strongest. In vitro cytotoxicity test and in vivo antitumor study showed that PTX/BA NM had better antitumor effect on MCF-7/Tax cells than other PTX preparations. Pangeni et al. (Citation2018) designed an oral co-administration system of pemetrexed (PMX) and Que based on oil-in-water NM (PMX/Nα-deoxycholyl-L-lysyl-methylester (DCK)-Que-NM). PMX/DCK-QCN-NE had good intestinal permeability and increased cell absorption of PMX/DCK and Que. In vivo experiments showed that compared with free PMX and QCN, the oral bioavailability of PMX and QCN in rats was significantly improved, and PMX/DCK-QCN-NE had the strongest inhibitory effect on tumor growth in A549 tumor-bearing mice.
4.7. Carrier-free NDCDS
Carrier-free NDCDS is a kind of covalent binding prodrug delivery system, and two identical or different drug molecules can be coupled together through chemical bonds to form the inactive precursor of the drug. When it reaches the tumor site, drug activation or biotransformation through environmental stimulation can prevent drug leakage in the process of in vivo circulation. Carrier-free NDCDS can improve drug loading, drug stability, and bioavailability, and increase drug safety, so as to achieve high flexibility in drug response release and synergistic combination therapy (Huang et al., Citation2021; Karaosmanoglu et al., Citation2021). A summary of the researches of carrier-free NDCDS used to co-deliver chemotherapy drugs and natural active ingredients is shown in .
Table 8. The researches of carrier-free NDCDS of natural active ingredients and chemotherapy drugs.
Some natural active ingredients and chemotherapy drugs can be coupled together via ester bonds or acetal bonds to form carrier-free nano-systems to obtain high drug loading and synergistic antitumor effects, such as PTX and succinic acid (SA) (Xu et al., Citation2017a), 5-FU and CA (Fang et al., Citation2021), MTX and ursolic acid (UA) () (Lan et al., Citation2021a). Additionally, natural active ingredients can also be coupled with chemotherapy drugs through disulfide bonds to form redox-sensitive carrier-free nano-system to achieve the controlled release of drugs in tumor-specific microenvironment, such as CPT and arabinoside (Ara-C) (He et al., Citation2017), CPT and GEM (Hou et al., Citation2017), CPT and PTX (Gao et al., Citation2020).
Figure 8. Schematic representation of the carrier-free nanoparticles (NPs) via co-assembly between UA and MTX. International Journal of Nanomedicine 2021 16 1775-1787’ Originally published by and used with permission from Dove Medical Press Ltd.
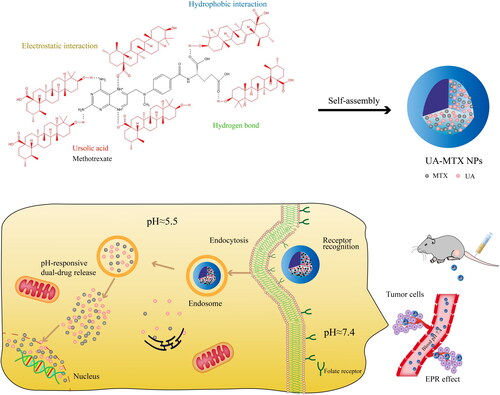
What is more, carrier-free NDCDS can maintain the optimal ratio of natural active ingredients and chemotherapy drugs. For example, one mole of the natural active ingredients and one mole of the chemotherapeutic drug can be linked together by ester bonds or disulfide bonds. Carrier-free nano-systems coupled by ester or disulfide bonds can release drugs in a fixed proportion, which have better antitumor effect. Li et al. (Citation2017d) coupled CPT and MTX by ester bond to synthesize MTX-CPT conjugates. In the tumor/lysosomal environment, the ester bond in the conjugate can be rapidly lysed by acid hydrolysis and/or enzymatic hydrolysis, and the dual drugs can be released synchronously at a fixed proportion. In vitro and in vivo studies suggested that the MTX-CPT NPs exhibited a superior synergistic effect, and could improve the therapeutic efficiency significantly with reduced toxicity compared to either individual free drug or a combination of both free drugs. Xu et al. (Citation2018) prepared a novel redox-sensitive Janus nanoprecursor drug synthesized by disulfide bond connection to achieve self-administration and synergistic anti-proliferation of CPT and GEM. Due to the hydrophobicity of CPT and the hydrophilicity of GEM, the prepared amphiphilic prodrug CPT-SS-GEM can self-assemble into organized nanoparticles to realize the self-delivery of CPT and GEM without additional materials. At the same time, the dose ratio of CPT and GEM was always 1:1 when CPT-SS-GEM was degraded by high concentration of glutathione in tumor cells, resulting in synergistic antitumor effect.
4.8. Other nanocarriers
In recent years, studies have shown that Graphene (Deb et al., Citation2018), gold nanocages (Zhang et al., Citation2018b), hydrogels (Ren et al., Citation2019; Wang et al., Citation2019b; Abedi et al., Citation2021), prodrug nanogel (Ma et al., Citation2022), microspheres (Liu et al., Citation2017; Xu et al., Citation2021) and nanoclusters (He et al., Citation2020) can also be used for the co-delivery of natural active ingredients of and chemotherapy drugs. A summary of the researches of other nanocarriers used to co-deliver chemotherapy drugs and natural active ingredients is shown in .
Table 9. The researches of other nanocarriers used to co-deliver chemotherapy drugs and natural active ingredients.
5. Discussion
With the deepening of the research on the mechanism of tumorigenesis and development, drug combination therapy shows obvious advantages in tumor treatment, and the development of nanotechnology in the field of pharmaceutics has brought broad application prospects. NDCDS of natural active ingredients and chemotherapy drugs also have advantages and limitations in tumor treatment.
Firstly, natural products are a key source for the development of innovative anti-cancer medicines that may be used both preventively and therapeutically, and natural active ingredients have effects on cellular processes and signaling pathways (Chavda et al., Citation2022), which can directly or indirectly affect tumor cells. Therefore, the natural active ingredients can play a synergistic role in combination with chemotherapy drugs. However, the potential regulation mechanism of some natural active components on tumor microenvironment is still in the preliminary research stage. A better understanding of the synergistic antitumor effect of natural active ingredients and chemotherapy drugs can develop more effective anti-tumor drugs. Secondly, although the combination of natural active ingredients and chemotherapy drugs can reduce the side effects of chemotherapy drugs by reducing the intake of chemotherapy drugs and directly acting on certain targets, the nanocarrier itself can also cause toxicity, and the toxicological properties of nanomaterials have gradually been paid attention to. Therefore, the biodegradable and biocompatibility materials will become the first choice for nanocarriers in the future, and the carrier-free NDCDS will also become the focus of attention. In addition, due to the limitations of safety, the complexity of the preparation process, and the controllability of precise drug release, the co-delivery nanocarrier preparations of natural active ingredients and chemotherapy drugs have not yet entered the clinical stage. Before the clinical stage, the formulation or technology of nanopreparations faces great challenges in achieving universal applicability and achieving effective loading, targeted delivery and sustained release of the two drugs at the required proportion, and there are several problems to be considered in formulating an ideal NDCDS.
First, the optimal ratio of natural active ingredients and chemotherapy drugs is the primary issue. Some studies have not determined the optimal ratio of natural active ingredients and chemotherapy drugs, others had carried out to investigate on the optimal ratio of natural active ingredients and chemotherapy drugs by in vitro cell tests, but it is often difficult to obtain the optimal ratio in vivo.
Second, selecting appropriate nano carriers to realize the effective encapsulation of natural active ingredients and chemotherapy drugs is the important procedure. A suitable nano carrier can maintain the ratio of the multiple drugs constant and deliver them stably to the tumor tissue. At present, multifunctional mesoporous silica nanoparticles, PLGA nanoparticles, bovine serum albumin-coated superparamagnetic iron oxide nanoparticles, liposomes, and carrier-free NDCDS help to control proportional release. At the same time, due to the fact that at least two drugs are loaded on NDCDS, and the water-solubility and physicochemical characteristics of drugs are different, so the procedure for drug loading needs to be carefully considered in preparation. In addition, during the preparation process, the loading of one drug may affect the encapsulation efficiency and drug loading of another drug.
Third, how to achieve the targeted delivery of multiple drugs requires precise design, which puts forward higher requirements for the complex structure of carriers. A delivery system with multi-target modified and multiple environmental responses is designed by encapsulating two or more stimulus response units, which is expected to achieve higher targeting efficiency and improve the efficacy.
Fourth, sequential and precise drug release in vivo is another impactful parameter of determining the synergistic action of co-delivery drugs. However, many studies had carried out to investigate on the drug release of NDCDS in model microenvironment with pH buffer of normal body fluid, or tumor tissue and cell, which is difficult to show the real extent of drug release in vivo.
Fifth, although NDCDS of natural active ingredients and chemotherapy drugs works well in the experiment, the clinical efficacy is still limited. More in-depth and effective pharmacodynamic evaluation methods are needed to explain the rationality of the combined use of natural active ingredients and chemotherapy drugs in NDCDS.
Although faced with many difficulties, it is believed that with the continuous revelation of the mechanism of action of natural active ingredients and the continuous development of nanotechnology, the NDCDS of natural active ingredients and chemotherapy drugs will show a promising prospect in antitumor therapy.
Disclosure statement
The authors report there are no competing interests to declare.
Correction statement
This article was originally published with errors, which have now been corrected in the online version. Please see Correction (http://dx.doi.org/10.1080/10717544.2022.2127213)
Additional information
Funding
References
- Abbasi A, Hajialyani M, Hosseinzadeh L, et al. (2020). Evaluation of the cytotoxic and apoptogenic effects of cinnamaldehyde on U87MG cells alone and in combination with doxorubicin. Res Pharm Sci 15:26–35.
- Abd Elwakil MM, Mabrouk MT, Helmy MW, et al. (2018). Inhalable lactoferrin-chondroitin nanocomposites for combined delivery of doxorubicin and ellagic acid to lung carcinoma. Nanomedicine (Lond) 13:2015–35.
- Abedi F, Davaran S, Hekmati M, et al. (2021). An improved method in fabrication of smart dual-responsive nanogels for controlled release of doxorubicin and curcumin in HT-29 colon cancer cells. J Nanobiotechnology 19:18.
- Ali N, Rashid S, Nafees S, et al. (2014). Beneficial effects of chrysin against Methotrexate-induced hepatotoxicity via attenuation of oxidative stress and apoptosis. Mol Cell Biochem 385:215–23.
- Amjadi S, Hamishehkar H, Ghorbani M. (2019). A novel smart PEGylated gelatin nanoparticle for co-delivery of doxorubicin and betanin: a strategy for enhancing the therapeutic efficacy of chemotherapy. Mater Sci Eng C Mater Biol Appl 97:833–41.
- Anbazhagan R, Muthusamy G, Krishnamoorthi R, et al. (2021). PAMAM G4.5 dendrimers for targeted delivery of ferulic acid and paclitaxel to overcome P-glycoprotein-mediated multidrug resistance. Biotechnol Bioeng 118:1213–23.
- Anwar DM, Khattab SN, Helmy MW, et al. (2018). Lactobionic/folate dual-targeted amphiphilic maltodextrin-based micelles for targeted codelivery of sulfasalazine and resveratrol to hepatocellular carcinoma. Bioconjug Chem 29:3026–41.
- Arranja AG, Pathak V, Lammers T, et al. (2017). Tumor-targeted nanomedicines for cancer theranostics. Pharmacol Res 115:87–95.
- Baek JS, Cho CW. (2017). A multifunctional lipid nanoparticle for co-delivery of paclitaxel and curcumin for targeted delivery and enhanced cytotoxicity in multidrug resistant breast cancer cells. Oncotarget 8:30369–82.
- Baghbani F, Moztarzadeh F. (2017). Bypassing multidrug resistant ovarian cancer using ultrasound responsive doxorubicin/curcumin co-deliver alginate nanodroplets. Colloids Surf B Biointerfaces 153:132–40.
- Bian Y, Guo D. (2020). Targeted therapy for hepatocellular carcinoma: co-delivery of sorafenib and curcumin using lactosylated pH-responsive nanoparticles. Drug Des Devel Ther 14:647–59.
- Borges GSM, Silva JO, Fernandes RS, et al. (2019). Sclareol is a potent enhancer of doxorubicin: evaluation of the free combination and co-loaded nanostructured lipid carriers against breast cancer. Life Sci 232:116678.
- Bray F, Ferlay J, Soerjomataram I, et al. (2018). Global cancer statistics 2018: GLOBOCAN estimates of incidence and mortality worldwide for 36 cancers in 185 countries. CA Cancer J Clin 68:394–424.
- Buhrmann C, Shayan P, Kraehe P, et al. (2015). Resveratrol induces chemosensitization to 5-fluorouracil through up-regulation of intercellular junctions, epithelial-to-mesenchymal transition and apoptosis in colorectal cancer. Biochem Pharmacol 98:51–68.
- Bukowski K, Kciuk M, Kontek R. (2020). Mechanisms of multidrug resistance in cancer chemotherapy. Int J Mol Sci 21:3233.
- Cai FY, Yao XM, Jing M, et al. (2020). Enhanced antitumour efficacy of functionalized doxorubicin plus schisandrin B co-delivery liposomes via inhibiting epithelial-mesenchymal transition. J Liposome Res 31:113–129.
- Cao C, Wang Q, Liu Y. (2019a). Lung cancer combination therapy: doxorubicin and beta-elemene co-loaded, pH-sensitive nanostructured lipid carriers. Drug Des Devel Ther 13:1087–98.
- Cao M, Long M, Chen Q, et al. (2019b). Development of beta-elemene and cisplatin co-loaded liposomes for effective lung cancer therapy and evaluation in patient-derived tumor xenografts. Pharm Res 36:121.
- Cao XF, Meng LH, Liu Z, et al. (2018). Research progress in reversing multidrug resistance of tumor by nanocarrier-mediated combined administration. China Pharmacy 29:716–20.
- Chavda VP, Patel AB, Mistry KJ, et al. (2022). Nano-drug delivery systems entrapping natural bioactive compounds for cancer: recent progress and future challenges. Front Oncol 12:867655.
- Chen F, Huang G. (2018). Preparation and immunological activity of polysaccharides and their derivatives. Int J Biol Macromol 112:211–6.
- Chen F, Zhao Y, Pan Y, et al. (2015). Synergistically enhanced therapeutic effect of a carrier-free HCPT/DOX nanodrug on breast cancer cells through improved cellular drug accumulation. Mol Pharm 12:2237–44.
- Chen HM, Lai ZQ, Liao HJ, et al. (2018). Synergistic antitumor effect of brusatol combined with cisplatin on colorectal cancer cells. Int J Mol Med 41:1447–54.
- Chen S, Liang Q, Liu E, et al. (2017). Curcumin/sunitinib co-loaded BSA-stabilized SPIOs for synergistic combination therapy for breast cancer. J Mater Chem B 5:4060–72.
- Chen S, Song Z, Feng R. (2020a). Recent development of copolymeric nano-drug delivery system for paclitaxel. Anticancer Agents Med Chem 20:2169–89.
- Chen X, Niu S, Bremner DH, et al. (2020b). Co-delivery of doxorubicin and oleanolic acid by triple-sensitive nanocomposite based on chitosan for effective promoting tumor apoptosis. Carbohydr Polym 247:116672.
- Chen X, Peng X, Luo Y, et al. (2019). Quercetin protects cardiomyocytes against doxorubicin-induced toxicity by suppressing oxidative stress and improving mitochondrial function via 14-3-3gamma. Toxicol Mech Methods 29:344–54.
- Chen Y, Zheng XL, Fang DL, et al. (2014). Dual agent loaded PLGA nanoparticles enhanced antitumor activity in a multidrug-resistant breast tumor xenograft model. Int J Mol Sci 15:2761–72.
- Cheng C, Sui B, Wang M, et al. (2020). Carrier-free nanoassembly of curcumin-erlotinib conjugate for cancer targeted therapy. Adv Healthc Mater 9:e2001128.
- Cheng T, Liu J, Ren J, et al. (2016). Green tea catechin-based complex micelles combined with doxorubicin to overcome cardiotoxicity and multidrug resistance. Theranostics 6:1277–92.
- Cheng Y, Zhao P, Wu S, et al. (2018). Cisplatin and curcumin co-loaded nano-liposomes for the treatment of hepatocellular carcinoma. Int J Pharm 545:261–73.
- Choi JY, Ramasamy T, Kim SY, et al. (2016). PEGylated lipid bilayer-supported mesoporous silica nanoparticle composite for synergistic co-delivery of axitinib and celastrol in multi-targeted cancer therapy. Acta Biomater 39:94–105.
- Chu PY, Tsai SC, Ko HY, et al. (2019). Co-delivery of natural compounds with a dual-targeted nanoparticle delivery system for improving synergistic therapy in an orthotopic tumor model. ACS Appl Mater Interfaces 11:23880–92.
- Chung SS, Dutta P, Austin D, et al. (2018). Combination of resveratrol and 5-flurouracil enhanced anti-telomerase activity and apoptosis by inhibiting STAT3 and Akt signaling pathways in human colorectal cancer cells. Oncotarget 9:32943–57.
- Coelho AR, Martins TR, Couto R, et al. (2017). Berberine-induced cardioprotection and Sirt3 modulation in doxorubicin-treated H9c2 cardiomyoblasts. Biochim Biophys Acta Mol Basis Dis 1863:2904–23.
- Cosco D, Paolino D, Maiuolo J, et al. (2015). Ultradeformable liposomes as multidrug carrier of resveratrol and 5-fluorouracil for their topical delivery. Int J Pharm 489:1–10.
- Cui T, Zhang S, Sun H. (2017). Co-delivery of doxorubicin and pH-sensitive curcumin prodrug by transferrin-targeted nanoparticles for breast cancer treatment. Oncol Rep 37:1253–60.
- Cui Y, Zhang M, Zeng F, et al. (2016). Dual-targeting magnetic PLGA nanoparticles for codelivery of paclitaxel and curcumin for brain tumor therapy. ACS Appl Mater Interfaces 8:32159–69.
- Dai H, Cheng Z, Zhang T, et al. (2022). Boron difluoride formazanate dye for high-efficiency NIR-II fluorescence imaging-guided cancer photothermal therapy. Chin Chem Lett 33:2501–6.
- Dang YP, Yuan XY, Tian R, et al. (2015). Curcumin improves the paclitaxel-induced apoptosis of HPV-positive human cervical cancer cells via the NF-kappaB-p53-caspase-3 pathway. Exp Ther Med 9:1470–6.
- Davaran S, Fazeli H, Ghamkhari A, et al. (2018). Synthesis and characterization of novel P(HEMA-LA-MADQUAT) micelles for co-delivery of methotrexate and chrysin in combination cancer chemotherapy. J Biomater Sci Polym Ed 29:1265–86.
- Deb A, Andrews NG, Raghavan V. (2018). Natural polymer functionalized graphene oxide for co-delivery of anticancer drugs: in-vitro and in-vivo. Int J Biol Macromol 113:515–25.
- Deng L, Zhu X, Yu Z, et al. (2020). Novel T7-modified pH-responsive targeted nanosystem for co-delivery of docetaxel and curcumin in the treatment of esophageal cancer. Int J Nanomedicine 15:7745–62.
- Dhanavel S, Nivethaa EAK, Narayanan V, et al. (2017). In vitro cytotoxicity study of dual drug loaded chitosan/palladium nanocomposite towards HT-29 cancer cells. Mater Sci Eng C Mater Biol Appl 75:1399–410.
- Dong K, Zhao ZZ, Kang J, et al. (2020). Cinnamaldehyde and doxorubicin co-loaded graphene oxide wrapped mesoporous silica nanoparticles for enhanced MCF-7 cell apoptosis. Int J Nanomedicine 15:10285–304.
- Eftekhari RB, Maghsoudnia N, Samimi S, et al. (2019). Co-delivery nanosystems for cancer treatment: a review. Pharm Nanotechnol 7:90–112.
- Fan X, Wang T, Ji Z, et al. (2021). Synergistic combination therapy of lung cancer using lipid-layered cisplatin and oridonin co-encapsulated nanoparticles. Biomed Pharmacother 141:111830.
- Fang J, Zhang S, Xue X, et al. (2018). Quercetin and doxorubicin co-delivery using mesoporous silica nanoparticles enhance the efficacy of gastric carcinoma chemotherapy. Int J Nanomedicine 13:5113–26.
- Fang JH, Lai YH, Chiu TL, et al. (2014). Magnetic core-shell nanocapsules with dual-targeting capabilities and co-delivery of multiple drugs to treat brain gliomas. Adv Healthc Mater 3:1250–60.
- Fang Q, Xu X, Yang L, et al. (2021). Self-assembled 5-fluorouracil-cinnamaldehyde nanodrugs for greatly improved chemotherapy in vivo. J Biomater Appl 36:592–604.
- Feng Q, Tong R. (2016). Anticancer nanoparticulate polymer-drug conjugate. Bioeng Transl Med 1:277–96.
- Fu L, Ma X, Liu Y, et al. (2022). Applying nanotechnology to boost cancer immunotherapy by promoting immunogenic cell death. Chin Chem Lett 33:1718–28.
- Gan D, He W, Yin H, et al. (2020). β-elemene enhances cisplatin-induced apoptosis in bladder cancer cells through the ROS-AMPK signaling pathway. Oncol Lett 19:291–300.
- Gao C, Tang F, Gong G, et al. (2017a). pH-responsive prodrug nanoparticles based on a sodium alginate derivative for selective co-release of doxorubicin and curcumin into tumor cells. Nanoscale 9:12533–42.
- Gao J, Fan K, Jin Y, et al. (2019). PEGylated lipid bilayer coated mesoporous silica nanoparticles co-delivery of paclitaxel and curcumin leads to increased tumor site drug accumulation and reduced tumor burden. Eur J Pharm Sci 140:105070.
- Gao J, Ma X, Zhang L, et al. (2020). Self-assembled disulfide bond bearing paclitaxel-camptothecin prodrug nanoparticle for lung cancer therapy. Pharmaceutics 12:1169.
- Gao X, Yu T, Xu G, et al. (2017b). Enhancing the anti-glioma therapy of doxorubicin by honokiol with biodegradable self-assembling micelles through multiple evaluations. Sci Rep 7:43501.
- Gao Y, Zhu Y, Xu X, et al. (2021). Surface PEGylated cancer cell membrane-coated nanoparticles for codelivery of curcumin and doxorubicin for the treatment of multidrug resistant esophageal carcinoma. Front Cell Dev Biol 9:688070.
- Gu J, Fan YQ, Zhang HL, et al. (2018). Resveratrol suppresses doxorubicin-induced cardiotoxicity by disrupting E2F1 mediated autophagy inhibition and apoptosis promotion. Biochem Pharmacol 150:202–13.
- Guo F, Yu N, Jiao Y, et al. (2021). Star polyester-based folate acid-targeting nanoparticles for doxorubicin and curcumin co-delivery to combat multidrug-resistant breast cancer. Drug Deliv 28:1709–21.
- Guo H, Zhang Z, Su Z, et al. (2016). Enhanced anti-tumor activity and reduced toxicity by combination andrographolide and bleomycin in ascitic tumor-bearing mice. Eur J Pharmacol 776:52–63.
- Guo P, Pi C, Zhao S, et al. (2020a). Oral co-delivery nanoemulsion of 5-fluorouracil and curcumin for synergistic effects against liver cancer. Expert Opin Drug Deliv 17:1473–84.
- Guo W, Song Y, Song W, et al. (2020b). Co-delivery of doxorubicin and curcumin with polypeptide nanocarrier for synergistic lymphoma therapy. Sci Rep 10:7832.
- Guo X, Zhao Z, Chen D, et al. (2019). Co-delivery of resveratrol and docetaxel via polymeric micelles to improve the treatment of drug-resistant tumors. Asian J Pharm Sci 14:78–85.
- Han NN, Li X, Tao L, et al. (2018). Doxorubicin and rhein loaded nanomicelles attenuates multidrug resistance in human ovarian cancer. Biochem Biophys Res Commun 498:178–85.
- He W, Hu X, Jiang W, et al. (2017). Rational design of a new self-codelivery system from redox-sensitive camptothecin-cytarabine conjugate assembly for effectively synergistic anticancer therapy. Adv Healthcare Mater 6:1700829.
- He Y, Shao L, Usman I, et al. (2020). A pH-responsive dissociable mesoporous silica-based nanoplatform enabling efficient dual-drug co-delivery and rapid clearance for cancer therapy. Biomater Sci 8:3418–29.
- Hiremath CG, Heggnnavar GB, Kariduraganavar MY, et al. (2019). Co-delivery of paclitaxel and curcumin to foliate positive cancer cells using pluronic-coated iron oxide nanoparticles. Prog Biomater 8:155–68.
- Ho JN, Byun SS, Lee S, et al. (2015). Synergistic antitumor effect of triptolide and cisplatin in cisplatin resistant human bladder cancer cells. J Urol 193:1016–22.
- Hou M, Xue P, Gao YE, et al. (2017). Gemcitabine-camptothecin conjugates: a hybrid prodrug for controlled drug release and synergistic therapeutics. Biomater Sci 5:1889–97.
- Hu J, Wang J, Wang G, et al. (2016). Pharmacokinetics and antitumor efficacy of DSPE-PEG2000 polymeric liposomes loaded with quercetin and temozolomide: analysis of their effectiveness in enhancing the chemosensitization of drug-resistant glioma cells. Int J Mol Med 37:690–702.
- Hu L, Pang S, Hu Q, et al. (2015). Enhanced antitumor efficacy of folate targeted nanoparticles co-loaded with docetaxel and curcumin. Biomed Pharmacother 75:26–32.
- Hu Y, Ran M, Wang B, et al. (2020). Co-delivery of docetaxel and curcumin via nanomicelles for enhancing anti-ovarian cancer treatment. Int J Nanomedicine 15:9703–15.
- Huang C, Yu Y. (2017). Synergistic cytotoxicity of beta-elemene and cisplatin in gingival squamous cell carcinoma by inhibition of STAT3 signaling pathway. Med Sci Monit 23:1507–13.
- Huang L, Zhang K, Guo Y, et al. (2017). Honokiol protects against doxorubicin cardiotoxicity via improving mitochondrial function in mouse hearts. Sci Rep 7:11989.
- Huang L, Zhao S, Fang F, et al. (2021). Advances and perspectives in carrier-free nanodrugs for cancer chemo-monotherapy and combination therapy. Biomaterials 268:120557.
- Huang PP, Fu J, Liu LH, et al. (2020). Honokiol antagonizes doxorubicininduced cardiomyocyte senescence by inhibiting TXNIP mediated NLRP3 inflammasome activation. Int J Mol Med 45:186–94.
- Huo M, Wang H, Zhang Y, et al. (2020). Co-delivery of silybin and paclitaxel by dextran-based nanoparticles for effective anti-tumor treatment through chemotherapy sensitization and microenvironment modulation. J Control Release 321:198–210.
- Jabri T, Imran M, Aziz A, et al. (2019). Design and synthesis of mixed micellar system for enhanced anticancer efficacy of Paclitaxel through its co-delivery with naringin. Drug Dev Ind Pharm 45:703–14.
- Jacob S, Nair AB, Shah J. (2020). Emerging role of nanosuspensions in drug delivery systems. Biomater Res 24:3.
- Jain A, Sharma G, Kushwah V, et al. (2017). Methotrexate and beta-carotene loaded-lipid polymer hybrid nanoparticles: a preclinical study for breast cancer. Nanomedicine (Lond) 12:1851–72.
- Jia L, Li Z, Shen J, et al. (2015). Multifunctional mesoporous silica nanoparticles mediated co-delivery of paclitaxel and tetrandrine for overcoming multidrug resistance. Int J Pharm 489:318–30.
- Jiang H, Geng D, Liu H, et al. (2016). Co-delivery of etoposide and curcumin by lipid nanoparticulate drug delivery system for the treatment of gastric tumors. Drug Deliv 23:3665–73.
- Jiang H, Li ZP, Tian GX, et al. (2019). Liver-targeted liposomes for codelivery of curcumin and combretastatin A4 phosphate: preparation, characterization, and antitumor effects. Int J Nanomedicine 14:1789–804.
- Jiang L, Li L, He X, et al. (2015). Overcoming drug-resistant lung cancer by paclitaxel loaded dual-functional liposomes with mitochondria targeting and pH-response. Biomaterials 52:126–39.
- Kabary DM, Helmy MW, Elkhodairy KA, et al. (2018). Hyaluronate/lactoferrin layer-by-layer-coated lipid nanocarriers for targeted co-delivery of rapamycin and berberine to lung carcinoma. Colloids Surf B Biointerfaces 169:183–94.
- Karaosmanoglu S, Zhou M, Shi B, et al. (2021). Carrier-free nanodrugs for safe and effective cancer treatment. J Control Release 329:805–32.
- Katiyar SS, Muntimadugu E, Rafeeqi TA, et al. (2016). Co-delivery of rapamycin- and piperine-loaded polymeric nanoparticles for breast cancer treatment. Drug Deliv 23:2608–16.
- Kazantseva L, Becerra J, Santos-Ruiz L. (2022). Oridonin enhances antitumor effects of doxorubicin in human osteosarcoma cells. Pharmacol Rep 74:248–56.
- Khaledi S, Jafari S, Hamidi S, et al. (2020). Preparation and characterization of PLGA-PEG-PLGA polymeric nanoparticles for co-delivery of 5-fluorouracil and Chrysin. J Biomater Sci Polym Ed 31:1107–26.
- Khan I, Joshi G, Nakhate KT, et al. (2019a). Nano-co-delivery of berberine and anticancer drug using PLGA nanoparticles: exploration of better anticancer activity and in vivo kinetics. Pharm Res 36:149.
- Khan MM, Madni A, Tahir N, et al. (2020). Co-delivery of curcumin and cisplatin to enhance cytotoxicity of cisplatin using lipid-chitosan hybrid nanoparticles. Int J Nanomedicine 15:2207–17.
- Khan MW, Zhao P, Khan A, et al. (2019b). Synergism of cisplatin-oleanolic acid co-loaded calcium carbonate nanoparticles on hepatocellular carcinoma cells for enhanced apoptosis and reduced hepatotoxicity. Int J Nanomedicine 14:3753–71.
- Kunjachan S, Rychlik B, Storm G, et al. (2013). Multidrug resistance: physiological principles and nanomedical solutions. Adv Drug Deliv Rev 65:1852–65.
- Lan JS, Qin YH, Liu L, et al. (2021a). A carrier-free folate receptor-targeted ursolic acid/methotrexate nanodelivery system for synergetic anticancer therapy. Int J Nanomedicine 16:1775–87.
- Lan M, Lu W, Zou T, et al. (2021b). Role of inflammatory microenvironment: potential implications for improved breast cancer nano-targeted therapy. Cell Mol Life Sci 78:2105–29.
- Lan Y, Sun Y, Yang T, et al. (2019). Co-delivery of paclitaxel by a capsaicin prodrug micelle facilitating for combination therapy on breast cancer. Mol Pharm 16:3430–40.ma ceut.9b00209
- Lee S, Lee SK, Jung J. (2021). Potentiating activities of chrysin in the therapeutic efficacy of 5-fluorouracil in gastric cancer cells. Oncol Lett 21:24.
- Li C, Ge X, Wang L. (2017a). Construction and comparison of different nanocarriers for co-delivery of cisplatin and curcumin: a synergistic combination nanotherapy for cervical cancer. Biomed Pharmacother 86:628–36.
- Li C, Lin J, Wu P, et al. (2018a). Small molecule nanodrug assembled of dual-anticancer drug conjugate for synergetic cancer metastasis therapy. Bioconjug Chem 29:3495–502.
- Li D, Cui R, Xu S, et al. (2020a). Synergism of cisplatin-oleanolic acid co-loaded hybrid nanoparticles on gastric carcinoma cells for enhanced apoptosis and reversed multidrug resistance. Drug Deliv 27:191–9.
- Li H, Li M, Chen C, et al. (2015a). On-demand combinational delivery of curcumin and doxorubicin via a pH-labile micellar nanocarrier. Int J Pharm 495:572–8.
- Li J, Duan B, Guo Y, et al. (2018b). Baicalein sensitizes hepatocellular carcinoma cells to 5-FU and epirubicin by activating apoptosis and ameliorating P-glycoprotein activity. Biomed Pharmacother 98:806–12.
- Li J, Zhang J, Wang Y, et al. (2017b). Synergistic inhibition of migration and invasion of breast cancer cells by dual docetaxel/quercetin-loaded nanoparticles via Akt/MMP-9 pathway. Int J Pharm 523:300–9.
- Li M, Du C, Guo N, et al. (2019a). Composition design and medical application of liposomes. Eur J Med Chem 164:640–53.
- Li M, Yue X, Wang Y, et al. (2019b). Remodeling the tumor microenvironment to improve drug permeation and antitumor effects by co-delivering quercetin and doxorubicin. J Mater Chem B 7:7619–26.
- Li N, Guo W, Li Y, et al. (2020b). Construction and anti-tumor activities of disulfide-linked docetaxel-dihydroartemisinin nanoconjugates. Colloids Surf B Biointerfaces 191:111018.
- Li Q, Fu D, Zhang J, et al. (2021a). Poly(aspartic acid)-based pH-responsive targeting co-delivery nanoparticles. J Biomater Appl 36:579–91.
- Li Q, Fu D, Zhang J, et al. (2021b). Dual stimuli-responsive polypeptide-calcium phosphate hybrid nanoparticles for co-delivery of multiple drugs in cancer therapy. Colloids Surf B Biointerfaces 200:111586.
- Li S, Wang L, Li N, et al. (2017c). Combination lung cancer chemotherapy: design of a pH-sensitive transferrin-PEG-Hz-lipid conjugate for the co-delivery of docetaxel and baicalin. Biomed Pharmacother 95:548–55.
- Li W, Hu X, Wang S, et al. (2020c). Characterization and anti-tumor bioactivity of astragalus polysaccharides by immunomodulation. Int J Biol Macromol 145:985–97.
- Li WM, Chiang CS, Huang WC, et al. (2015b). Amifostine-conjugated pH-sensitive calcium phosphate-covered magnetic-amphiphilic gelatin nanoparticles for controlled intracellular dual drug release for dual-targeting in HER-2-overexpressing breast cancer. J Control Release 220:107–18.
- Li X, Yang S, Wang L, et al. (2019c). Resveratrol inhibits paclitaxel-induced neuropathic pain by the activation of PI3K/Akt and SIRT1/PGC1alpha pathway. JPR 12:879–90. S185873
- Li X, Yu N, Li J, et al. (2020d). Novel "carrier-free" nanofiber codelivery systems with the synergistic antitumor effect of paclitaxel and tetrandrine through the enhancement of mitochondrial apoptosis. ACS Appl Mater Interfaces 12:10096–106.
- Li Y, Li D, Wang P, et al. (2020e). Tetrandrine partially reverses multidrug resistance of human laryngeal cancer cells. J Int Med Res 48:300060520944706.
- Li Y, Li X, Lu Y, et al. (2020f). Co-delivery of Poria cocos extract and doxorubicin as an ‘all-in-one’ nanocarrier to combat breast cancer multidrug resistance during chemotherapy. Nanomedicine 23:102095.
- Li Y, Lin J, Ma J, et al. (2017d). Methotrexate-camptothecin prodrug nanoassemblies as a versatile nanoplatform for biomodal imaging-guided self-active targeted and synergistic chemotherapy. ACS Appl Mater Interfaces 9:34650–65.
- Li Z, Zhang Y, Zhu C, et al. (2020g). Folic acid modified lipid-bilayer coated mesoporous silica nanoparticles co-loading paclitaxel and tanshinone IIA for the treatment of acute promyelocytic leukemia. Int J Pharm 586:119576.
- Liao D, Zhang W, Gupta P, et al. (2019). Tetrandrine interaction with ABCB1 reverses multidrug resistance in cancer cells through competition with anti-cancer drugs followed by downregulation of ABCB1 expression. Molecules 24:4383.
- Lin D, Lin W, Gao G, et al. (2020). Purification and characterization of the major protein isolated from Semen Armeniacae Amarum and the properties of its thermally induced nanoparticles. Int J Biol Macromol 159:850–8.
- Lin J, Cai Q, Tang Y, et al. (2018). PEGylated lipid bilayer coated mesoporous silica nanoparticles for co-delivery of paclitaxel and curcumin: design, characterization and its cytotoxic effect. Int J Pharm 536:272–82.
- Lin JT, Ye QB, Yang QJ, et al. (2019). Hierarchical bioresponsive nanocarriers for codelivery of curcumin and doxorubicin. Colloids Surf B Biointerfaces 180:93–101.
- Liu H, Yuan M, Liu Y, et al. (2021a). Self-monitoring and self-delivery of self-assembled fluorescent nanoparticles in cancer therapy. Int J Nanomedicine 16:2487–99.
- Liu J, Cheng H, Han L, et al. (2018). Synergistic combination therapy of lung cancer using paclitaxel- and triptolide-coloaded lipid-polymer hybrid nanoparticles. Drug Des Devel Ther 12:3199–209.
- Liu K, Chen W, Yang T, et al. (2017). Paclitaxel and quercetin nanoparticles co-loaded in microspheres to prolong retention time for pulmonary drug delivery. Int J Nanomedicine 12:8239–55.
- Liu L, Qi XJ, Zhong ZK, et al. (2016a). Nanomedicine-based combination of gambogic acid and retinoic acid chlorochalcone for enhanced anticancer efficacy in osteosarcoma. Biomed Pharmacother 83:79–84.
- Liu M, Fu M, Yang X, et al. (2020a). Paclitaxel and quercetin co-loaded functional mesoporous silica nanoparticles overcoming multidrug resistance in breast cancer. Colloids Surf B Biointerfaces 196:111284.
- Liu Q, Li J, Pu G, et al. (2016b). Co-delivery of baicalein and doxorubicin by hyaluronic acid decorated nanostructured lipid carriers for breast cancer therapy. Drug Deliv 23:1364–8.
- Liu Y, Yang S, Wang K, et al. (2020b). Cellular senescence and cancer: focusing on traditional Chinese medicine and natural products. Cell Prolif 53:e12894.
- Liu Z, Chu W, Sun Q, et al. (2021b). Micelle-contained and PEGylated hybrid liposomes of combined gemcitabine and cisplatin delivery for enhancing antitumor activity. Int J Pharm 602:120619.
- Lockhart JN, Stevens DM, Beezer DB, et al. (2015). Dual drug delivery of tamoxifen and quercetin: regulated metabolism for anticancer treatment with nanosponges. J Control Release 220:751–7.
- Lőrincz A, Mihály J, Wacha A, et al. (2021). Combination of multifunctional ursolic acid with kinase inhibitors for anti-cancer drug carrier vesicles. Mater Sci Eng C Mater Biol Appl 131:112481.
- Lu L, Zhang M, Wang X, et al. (2020a). Baicalein enhances the antitumor efficacy of docetaxel on nonsmall cell lung cancer in a beta-catenin-dependent manner. Phytother Res 34:104–17.
- Lu X, Yang F, Chen D, et al. (2020b). Quercetin reverses docetaxel resistance in prostate cancer via androgen receptor and PI3K/Akt signaling pathways. Int J Biol Sci 16:1121–34.
- Lu Y, Wang J, Liu L, et al. (2017a). Curcumin increases the sensitivity of Paclitaxel-resistant NSCLC cells to paclitaxel through microRNA-30c-mediated MTA1 reduction. Tumour Biol 39:1010428317698353.
- Lu Z, Su J, Li Z, et al. (2017b). Hyaluronic acid-coated, prodrug-based nanostructured lipid carriers for enhanced pancreatic cancer therapy. Drug Dev Ind Pharm 43:160–70.
- Lv L, Liu C, Chen C, et al. (2016a). Quercetin and doxorubicin co-encapsulated biotin receptor-targeting nanoparticles for minimizing drug resistance in breast cancer. Oncotarget 7:32184–99.
- Lv L, Qiu K, Yu X, et al. (2016b). Amphiphilic copolymeric micelles for doxorubicin and curcumin co-delivery to reverse multidrug resistance in breast cancer. J Biomed Nanotechnol 12:973–85.
- Lv X, Zhu Y, Deng Y, et al. (2020). Glycyrrhizin improved autophagy flux via HMGB1-dependent Akt/mTOR signaling pathway to prevent doxorubicin-induced cardiotoxicity. Toxicology 441:152508.
- Ma X, Yang S, Zhang T, et al. (2022). Bioresponsive immune-booster-based prodrug nanogel for cancer immunotherapy. Acta Pharm Sin B 12:451–66.
- Mahira S, Kommineni N, Husain GM, et al. (2019). Cabazitaxel and silibinin co-encapsulated cationic liposomes for CD44 targeted delivery: a new insight into nanomedicine based combinational chemotherapy for prostate cancer. Biomed Pharmacother 110:803–17.
- Mahmoudi R, Hassandokht F, Ardakani MT, et al. (2021). Intercalation of curcumin into liposomal chemotherapeutic agent augments apoptosis in breast cancer cells. J Biomater Appl 35:1005–18.
- Majidinia M, Mirza-Aghazadeh-Attari M, Rahimi M, et al. (2020). Overcoming multidrug resistance in cancer: recent progress in nanotechnology and new horizons. IUBMB Life 72:855–71.
- Maleki H, Hosseini Najafabadi MR, Webster TJ, et al. (2021). Effect of paclitaxel/etoposide co-loaded polymeric nanoparticles on tumor size and survival rate in a rat model of glioblastoma. Int J Pharm 604:120722.
- Martinez-Edo G, Fornaguera C, Borros S, et al. (2020). Glycyrrhetinic acid-functionalized mesoporous silica nanoparticles for the co-delivery of DOX/CPT-PEG for targeting HepG2 cells. Pharmaceutics 12:1048.
- Meng J, Guo F, Xu H, et al. (2016a). Combination therapy using co-encapsulated resveratrol and paclitaxel in liposomes for drug resistance reversal in breast cancer cells in vivo. Sci Rep 6:22390.
- Meng L, Xia X, Yang Y, et al. (2016b). Co-encapsulation of paclitaxel and baicalein in nanoemulsions to overcome multidrug resistance via oxidative stress augmentation and P-glycoprotein inhibition. Int J Pharm 513:8–16.
- Mi FL, Wang LF, Chu PY, et al. (2018). Active tumor-targeted co-delivery of epigallocatechin gallate and doxorubicin in nanoparticles for combination gastric cancer therapy. ACS Biomater Sci Eng 4:2847–59.
- Mohammad IS, He W, Yin L. (2020). Insight on multidrug resistance and nanomedicine approaches to overcome MDR. Crit Rev Ther Drug Carrier Syst 37:473–509.
- Mohanty AK, Mohanta GP. (2015). Micelle-assisted combination therapies for effective glioblastoma treatment: an in-vitro assessment. Anticancer Drugs 26:312–22.
- Muthusamy G, Balupillai A, Ramasamy K, et al. (2016). Ferulic acid reverses ABCB1-mediated paclitaxel resistance in MDR cell lines. Eur J Pharmacol 786:194–203.
- Nguyen NT, Nguyen NNT, Tran NTN, et al. (2018). Synergic activity against MCF-7 breast cancer cell growth of nanocurcumin-encapsulated and cisplatin-complexed nanogels. Molecules 23:3347.
- Oz M, Nurullahoglu Atalik KE, Yerlikaya FH, et al. (2015). Curcumin alleviates cisplatin-induced learning and memory impairments. Neurobiol Learn Mem 123:43–9.
- Ozkaya D, Naziroglu M. (2020). Curcumin diminishes cisplatin-induced apoptosis and mitochondrial oxidative stress through inhibition of TRPM2 channel signaling pathway in mouse optic nerve. J Recept Signal Transduct Res 40:97–108.
- Ozturk Y, Gunaydin C, Yalcin F, et al. (2019). Resveratrol enhances apoptotic and oxidant effects of paclitaxel through TRPM2 channel activation in DBTRG glioblastoma cells. Oxid Med Cell Longev 2019:4619865.
- Pang L, Zhang L, Zhou H, et al. (2022). Reactive oxygen species-responsive nanococktail with self-amplificated drug release for efficient co-delivery of paclitaxel/cucurbitacin B and synergistic treatment of gastric cancer. Front Chem 10:844426.
- Pangeni R, Panthi VK, Yoon IS, et al. (2018). Preparation, characterization, and in vivo evaluation of an oral multiple nanoemulsive system for co-delivery of pemetrexed and quercetin. Pharmaceutics 10:158.
- Peng J, Fumoto S, Miyamoto H, et al. (2017). One-step formation of lipid-polyacrylic acid-calcium carbonate nanoparticles for co-delivery of doxorubicin and curcumin. J Drug Target 25:704–14.
- Phung CD, Le TG, Nguyen VH, et al. (2020). PEGylated-paclitaxel and dihydroartemisinin nanoparticles for simultaneously delivering paclitaxel and dihydroartemisinin to colorectal cancer. Pharm Res 37:129.
- Pillai VB, Kanwal A, Fang YH, et al. (2017). Honokiol, an activator of Sirtuin-3 (SIRT3) preserves mitochondria and protects the heart from doxorubicin-induced cardiomyopathy in mice. Oncotarget 8:34082–98.
- Potocnjak I, Simic L, Vukelic I, et al. (2019). Oleanolic acid attenuates cisplatin-induced nephrotoxicity in mice and chemosensitizes human cervical cancer cells to cisplatin cytotoxicity. Food Chem Toxicol 132:110676.
- Qi M, Zou S, Guo C, et al. (2018). Enhanced in vitro and in vivo anticancer properties by using a nanocarrier for co-delivery of antitumor polypeptide and curcumin. J Biomed Nanotechnol 14:139–49.
- Qi SS, Sun JH, Yu HH, et al. (2017). Co-delivery nanoparticles of anti-cancer drugs for improving chemotherapy efficacy. Drug Deliv 24:1909–26.
- Quagliariello V, Iaffaioli RV, Armenia E, et al. (2017). Hyaluronic acid nanohydrogel loaded with quercetin alone or in combination to a macrolide derivative of rapamycin RAD001 (everolimus) as a new treatment for hormone-responsive human breast cancer. J Cell Physiol 232:2063–74.
- Rawal S, Bora V, Patel B, et al. (2020a). Surface-engineered nanostructured lipid carrier systems for synergistic combination oncotherapy of non-small cell lung cancer. Drug Deliv Transl Res 11:2030–51.
- Rawal S, Patel B, Patel MM. (2020b). Fabrication, optimisation and in vitro evaluation of docetaxel and curcumin co-loaded nanostructured lipid carriers for improved antitumor activity against non-small cell lung carcinoma. J Microencapsul 37:543–56.
- Ren Y, Li X, Han B, et al. (2019). Improved anti-colorectal carcinomatosis effect of tannic acid co-loaded with oxaliplatin in nanoparticles encapsulated in thermosensitive hydrogel. Eur J Pharm Sci 128:279–89.
- Rudnik LAC, Farago PV, Manfron Budel J, et al. (2020). Co-loaded curcumin and methotrexate nanocapsules enhance cytotoxicity against non-small-cell lung cancer cells. Molecules 25:1913.
- Ruttala HB, Ko YT. (2015). Liposomal co-delivery of curcumin and albumin/paclitaxel nanoparticle for enhanced synergistic antitumor efficacy. Colloids Surf B Biointerfaces 128:419–26.
- Sabra SA, Elzoghby AO, Sheweita SA, et al. (2018). Self-assembled amphiphilic zein-lactoferrin micelles for tumor targeted co-delivery of rapamycin and wogonin to breast cancer. Eur J Pharm Biopharm 128:156–69.
- Sabzi A, Rahmani A, Edalati M, et al. (2020). Targeted co-delivery of curcumin and doxorubicin by citric acid functionalized Poly (epsilon-caprolactone) based micelle in MDA-MB-231 cell. Colloids Surf B Biointerfaces 194:111225.
- Sahu BP, Hazarika H, Bharadwaj R, et al. (2016). Curcumin-docetaxel co-loaded nanosuspension for enhanced anti-breast cancer activity. Expert Opin Drug Deliv 13:1065–74.
- Saneja A, Kumar R, Mintoo MJ, et al. (2019). Gemcitabine and betulinic acid co-encapsulated PLGA-PEG polymer nanoparticles for improved efficacy of cancer chemotherapy. Mater Sci Eng C Mater Biol Appl 98:764–71.
- Sang DP, Li RJ, Lan Q. (2014). Quercetin sensitizes human glioblastoma cells to temozolomide in vitro via inhibition of Hsp27. Acta Pharmacol Sin 35:832–8.
- Sarisozen C, Dhokai S, Tsikudo EG, et al. (2016). Nanomedicine based curcumin and doxorubicin combination treatment of glioblastoma with scFv-targeted micelles: In vitro evaluation on 2D and 3D tumor models. Eur J Pharm Biopharm 108:54–67.
- Sesarman A, Tefas L, Sylvester B, et al. (2019). Co-delivery of curcumin and doxorubicin in PEGylated liposomes favored the antineoplastic C26 murine colon carcinoma microenvironment. Drug Deliv Transl Res 9:260–72.
- Shao Y, Luo W, Guo Q, et al. (2019). In vitro and in vivo effect of hyaluronic acid modified, doxorubicin and gallic acid co-delivered lipid-polymeric hybrid nano-system for leukemia therapy. Drug Des Devel Ther 13:2043–55.
- Shen Q, Qiu L. (2017). Reversal of P-glycoprotein-mediated multidrug resistance by doxorubicin and quinine co-loaded liposomes in tumor cells. J Liposome Res 27:293–301.
- Shen S, Xu X, Lin S, et al. (2021). A nanotherapeutic strategy to overcome chemotherapeutic resistance of cancer stem-like cells. Nat Nanotechnol 16:104–13.
- Siegel RL, Miller KD, Fuchs HE, et al. (2021). Cancer Statistics, 2021. CA Cancer J Clin 71:7–33.
- Soetikno V, Sari SDP, Ul Maknun L, et al. (2019). Pre-treatment with curcumin ameliorates cisplatin-induced kidney damage by suppressing kidney inflammation and apoptosis in rats. Drug Res (Stuttg) 69:75–82.
- Sohail M, Guo W, Li Z, et al. (2021). Nanocarrier-based drug delivery system for cancer therapeutics: a review of the last decade. Curr Med Chem 28:3753–72.
- Song Z, Shi Y, Han Q, et al. (2018). Endothelial growth factor receptor-targeted and reactive oxygen species-responsive lung cancer therapy by docetaxel and resveratrol encapsulated lipid-polymer hybrid nanoparticles. Biomed Pharmacother 105:18–26.
- Sun G, Sun K, Sun J. (2021a). Combination prostate cancer therapy: prostate-specific membranes antigen targeted, pH-sensitive nanoparticles loaded with doxorubicin and tanshinone. Drug Deliv 28:1132–40.
- Sun L, Cao J, Chen K, et al. (2019). Betulinic acid inhibits stemness and EMT of pancreatic cancer cells via activation of AMPK signaling. Int J Oncol 54:98–110.
- Sun Y, Ma X, Hu H. (2021b). Marine polysaccharides as a versatile biomass for the construction of nano drug delivery systems. Mar Drugs 19:345.
- Tai W, Mo R, Lu Y, et al. (2014). Folding graft copolymer with pendant drug segments for co-delivery of anticancer drugs. Biomaterials 35:7194–203.
- Tang Y, Liang J, Wu A, et al. (2017). Co-delivery of trichosanthin and albendazole by nano-self-assembly for overcoming tumor multidrug-resistance and metastasis. ACS Appl Mater Interfaces 9:26648–64.
- Tapeinos C, Battaglini M, Ciofani G. (2017). Advances in the design of solid lipid nanoparticles and nanostructured lipid carriers for targeting brain diseases. J Control Release 264:306–32.
- Tefas LR, Sylvester B, Tomuta I, et al. (2017). Development of antiproliferative long-circulating liposomes co-encapsulating doxorubicin and curcumin, through the use of a quality-by-design approach. Drug Des Devel Ther 11:1605–21.
- Thandavarayan RA, Giridharan VV, Arumugam S, et al. (2015). Schisandrin B prevents doxorubicin induced cardiac dysfunction by modulation of DNA damage, oxidative stress and inflammation through inhibition of MAPK/p53 signaling. PLoS One 10:e0119214.
- Vakilinezhad MA, Amini A, Dara T, et al. (2019). Methotrexate and curcumin co-encapsulated PLGA nanoparticles as a potential breast cancer therapeutic system: In vitro and in vivo evaluation. Colloids Surf B Biointerfaces 184:110515.
- Varshosaz J, Jandaghian S, Mirian M, et al. (2021). Co-delivery of rituximab targeted curcumin and imatinib nanostructured lipid carriers in non-Hodgkin lymphoma cells. J Liposome Res 31:64–78.
- Vinod BS, Nair HH, Vijayakurup V, et al. (2015). Resveratrol chemosensitizes HER-2-overexpressing breast cancer cells to docetaxel chemoresistance by inhibiting docetaxel-mediated activation of HER-2-Akt axis. Cell Death Discov 1:15061.
- Wan X, Beaudoin JJ, Vinod N, et al. (2019). Co-delivery of paclitaxel and cisplatin in poly(2-oxazoline) polymeric micelles: implications for drug loading, release, pharmacokinetics and outcome of ovarian and breast cancer treatments. Biomaterials 192:1–14.
- Wang B, Gou M, Zheng X, et al. (2010). Co-delivery honokiol and doxorubicin in MPEG-PLA nanoparticles. J Nanosci Nanotechnol 10:4166–72.
- Wang C, Su L, Wu C, et al. (2016). RGD peptide targeted lipid-coated nanoparticles for combinatorial delivery of sorafenib and quercetin against hepatocellular carcinoma. Drug Dev Ind Pharm 42:1938–44.
- Wang C, Zhu J, Ma J, et al. (2019a). Functionalized Bletilla striata polysaccharide micelles for targeted intracellular delivery of doxorubicin: in vitro and in vivo evaluation. Int J Pharm 567:118436.
- Wang J, Ma W, Tu P. (2015a). Synergistically improved anti-tumor efficacy by co-delivery doxorubicin and curcumin polymeric micelles. Macromol Biosci 15:1252–61.
- Wang MZ, He X, Yu Z, et al. (2020). A nano drug delivery system based on Angelica sinensis polysaccharide for combination of chemotherapy and immunotherapy. Molecules 25:3096.
- Wang QS, Gao LN, Zhu XN, et al. (2019b). Co-delivery of glycyrrhizin and doxorubicin by alginate nanogel particles attenuates the activation of macrophage and enhances the therapeutic efficacy for hepatocellular carcinoma. Theranostics 9:6239–55.
- Wang R, Huang J, Chen J, et al. (2019c). Enhanced anti-colon cancer efficacy of 5-fluorouracil by epigallocatechin-3-gallate co-loaded in wheat germ agglutinin-conjugated nanoparticles. Nanomedicine 21:102068.
- Wang R, Yang M, Li G, et al. (2019d). Paclitaxel-betulinic acid hybrid nanosuspensions for enhanced anti-breast cancer activity. Colloids Surf B Biointerfaces 174:270–9.
- Wang S, Wang A, Shao M, et al. (2017). Schisandrin B reverses doxorubicin resistance through inhibiting P-glycoprotein and promoting proteasome-mediated degradation of survivin. Sci Rep 7:8419.
- Wang W, Xi M, Duan X, et al. (2015b). Delivery of baicalein and paclitaxel using self-assembled nanoparticles: synergistic antitumor effect in vitro and in vivo. Int J Nanomedicine 10:3737–50.
- Wang X, Liu Y, Xu W, et al. (2021). Irinotecan and berberine co-delivery liposomes showed improved efficacy and reduced intestinal toxicity compared with onivyde for pancreatic cancer. Drug Deliv Transl Res 11:2186–97.
- Wu L, Cao K, Ni Z, et al. (2019a). Rhein reverses doxorubicin resistance in SMMC-7721 liver cancer cells by inhibiting energy metabolism and inducing mitochondrial permeability transition pore opening. Biofactors 45:85–96.
- Wu L, Liu X, Cao KX, et al. (2020a). Synergistic antitumor effects of rhein and doxorubicin in hepatocellular carcinoma cells. J Cell Biochem 121:4009–21.
- Wu YZ, Zhang L, Wu ZX, et al. (2019b). Berberine ameliorates doxorubicin-induced cardiotoxicity via a SIRT1/p66Shc-mediated pathway. Oxid Med Cell Longev 2019:2150394.
- Wu Z, Li S, Cai Y, et al. (2020b). Synergistic action of doxorubicin and 7-ethyl-10-hydroxycamptothecin polyphosphorylcholine polymer prodrug. Colloids Surf B Biointerfaces 189:110741.
- Xiao H, Xiong L, Song X, et al. (2017). Angelica sinensis polysaccharides ameliorate stress-induced premature senescence of hematopoietic cell via protecting bone marrow stromal cells from oxidative injuries caused by 5-fluorouracil. Int J Mol Sci 18:2265.
- Xiao Y, Zhang T, Ma X, et al. (2021). Microenvironment-responsive prodrug-induced pyroptosis boosts cancer immunotherapy. Adv Sci (Weinh) 8:e2101840.
- Xiong K, Zhang Y, Wen Q, et al. (2020). Co-delivery of paclitaxel and curcumin by biodegradable polymeric nanoparticles for breast cancer chemotherapy. Int J Pharm 589:119875.
- Xu H, Lu X, Li J, et al. (2017a). Superior antitumor effect of extremely high drug loading self-assembled paclitaxel nanofibers. Int J Pharm 526:217–24.
- Xu J, Geng MY, Hang M. (2019a). Mechanistic advancement in chemotherapeutic agents modulated antitumor immune response. Acta Pharmaceutica Sinica 54:1741–8.
- Xu J, Liu D, Niu H, et al. (2017b). Resveratrol reverses Doxorubicin resistance by inhibiting epithelial-mesenchymal transition (EMT) through modulating PTEN/Akt signaling pathway in gastric cancer. J Exp Clin Cancer Res 36:19.
- Xu M, Li G, Zhang H, et al. (2020). Sequential delivery of dual drugs with nanostructured lipid carriers for improving synergistic tumor treatment effect. Drug Deliv 27:983–95.
- Xu Y, Huang Y, Lu W, et al. (2019b). 4-Carboxyphenylboronic acid-decorated, redox-sensitive rod-shaped nano-micelles fabricated through co-assembling strategy for active targeting and synergistic co-delivery of camptothecin and gemcitabine. Eur J Pharm Biopharm 144:193–206.
- Xu Y, Huang Y, Zhang X, et al. (2018). Carrier-free Janus nano-prodrug based on camptothecin and gemcitabine: reduction-triggered drug release and synergistic in vitro antiproliferative effect in multiple cancer cells. Int J Pharm 550:45–56.
- Xu Y, Liu Y, Liu Q, et al. (2021). Co-delivery of bufalin and nintedanib via albumin sub-microspheres for synergistic cancer therapy. J Control Release 338:705–18.
- Xu Y, Wang C, Ding Y, et al. (2016). Nanoparticles with optimal ratiometric co-delivery of docetaxel with gambogic acid for treatment of multidrug-resistant breast cancer. J Biomed Nanotechnol 12:1774–81.
- Xue P, Liu D, Wang J, et al. (2016). Redox-sensitive citronellol-cabazitaxel conjugate: maintained in vitro cytotoxicity and self-assembled as multifunctional nanomedicine. Bioconjug Chem 27:1360–72.
- Yan J, Wang Y, Jia Y, et al. (2017). Co-delivery of docetaxel and curcumin prodrug via dual-targeted nanoparticles with synergistic antitumor activity against prostate cancer. Biomed Pharmacother 88:374–83.
- Yang M, Li J, Gu P, et al. (2021). The application of nanoparticles in cancer immunotherapy: targeting tumor microenvironment. Bioact Mater 6:1973–87.
- Yang Z, Sun N, Cheng R, et al. (2017a). Hybrid nanoparticles coated with hyaluronic acid lipoid for targeted co-delivery of paclitaxel and curcumin to synergistically eliminate breast cancer stem cells. J Mater Chem B 5:6762–75.
- Yang Z, Sun N, Cheng R, et al. (2017b). pH multistage responsive micellar system with charge-switch and PEG layer detachment for co-delivery of paclitaxel and curcumin to synergistically eliminate breast cancer stem cells. Biomaterials 147:53–67.
- Yao Q, Gutierrez DC, Hoang NH, et al. (2017). Efficient codelivery of paclitaxel and curcumin by novel bottlebrush copolymer-based micelles. Mol Pharm 14:2378–89.
- Yin J, Li Q, Sun LD, et al. (2019). Research advancement in natural anti-cancer product. Zhongguo Zhong Yao Za Zhi 44:19–27.
- Yokoyama M. (2014). Polymeric micelles as drug carriers: their lights and shadows. J Drug Target 22:576–83.
- Yu M, Qi B, Xiaoxiang W, et al. (2017). Baicalein increases cisplatin sensitivity of A549 lung adenocarcinoma cells via PI3K/Akt/NF-kappaB pathway. Biomed Pharmacother 90:677–85.
- Yu X, Sun L, Tan L, et al. (2020). Preparation and characterization of PLGA-PEG-PLGA nanoparticles containing salidroside and tamoxifen for breast cancer therapy. AAPS PharmSciTech 21:85.
- Yuan CH, Horng CT, Lee CF, et al. (2017). Epigallocatechin gallate sensitizes cisplatin-resistant oral cancer CAR cell apoptosis and autophagy through stimulating AKT/STAT3 pathway and suppressing multidrug resistance 1 signaling. Environ Toxicol 32:845–55.
- Yukuyama MN, Kato ETM, Lobenberg R, et al. (2017). Challenges and future prospects of nanoemulsion as a drug delivery system. Curr Pharm Des 23:495–508.
- Zan Y, Dai Z, Liang L, et al. (2019). Co-delivery of plantamajoside and sorafenib by a multi-functional nanoparticle to combat the drug resistance of hepatocellular carcinoma through reprograming the tumor hypoxic microenvironment. Drug Deliv 26:1080–91.
- Zang X, Cheng M, Zhang X, et al. (2021). Quercetin nanoformulations: a promising strategy for tumor therapy. Food Funct 12:6664–81.
- Zeng X, Zhang Y, Xu X, et al. (2022). Construction of pH-sensitive targeted micelle system co-delivery with curcumin and dasatinib and evaluation of anti-liver cancer. Drug Deliv 29:792–806.
- Zeng YY, Zeng YJ, Zhang NN, et al. (2019). The preparation, determination of a flexible complex liposome co-loaded with cabazitaxel and β-elemene, and animal pharmacodynamics on paclitaxel-resistant lung adenocarcinoma. Molecules 24:1697.
- Zhai B, Zhang N, Han X, et al. (2019). Molecular targets of β-elemene, a herbal extract used in traditional Chinese medicine, and its potential role in cancer therapy: a review. Biomed Pharmacother 114:108812.
- Zhan XK, Li JL, Zhang S, et al. (2018). Betulinic acid exerts potent antitumor effects on paclitaxel-resistant human lung carcinoma cells (H460) via G2/M phase cell cycle arrest and induction of mitochondrial apoptosis. Oncol Lett 16:3628–34.
- Zhang D, Xu Q, Wang N, et al. (2018a). A complex micellar system co-delivering curcumin with doxorubicin against cardiotoxicity and tumor growth. Int J Nanomedicine 13:4549–61.
- Zhang F, Jia Y, Zheng X, et al. (2019a). Janus nanocarrier-based co-delivery of doxorubicin and berberine weakens chemotherapy-exacerbated hepatocellular carcinoma recurrence. Acta Biomater 100:352–64.
- Zhang H, Tian Y, Zhu Z, et al. (2016a). Efficient antitumor effect of co-drug-loaded nanoparticles with gelatin hydrogel by local implantation. Sci Rep 6:26546.
- Zhang J, Hu K, Di L, et al. (2021a). Traditional herbal medicine and nanomedicine: converging disciplines to improve therapeutic efficacy and human health. Adv Drug Deliv Rev 178:113964.
- Zhang J, Li J, Shi Z, et al. (2017a). pH-sensitive polymeric nanoparticles for co-delivery of doxorubicin and curcumin to treat cancer via enhanced pro-apoptotic and anti-angiogenic activities. Acta Biomater 58:349–64.
- Zhang J, Wang L, Fai Chan H, et al. (2017b). Co-delivery of paclitaxel and tetrandrine via iRGD peptide conjugated lipid-polymer hybrid nanoparticles overcome multidrug resistance in cancer cells. Sci Rep 7:46057.
- Zhang L, Zhu H, Gu Y, et al. (2019b). Dual drug-loaded PLA nanoparticles bypassing drug resistance for improved leukemia therapy. J Nanopart Res 21:83.
- Zhang R, Lu M, Zhang Z, et al. (2016b). Resveratrol reverses P-glycoprotein-mediated multidrug resistance of U2OS/ADR cells by suppressing the activation of the NF-kappaB and p38 MAPK signaling pathways. Oncol Lett 12:4147–54.
- Zhang R, Zhang Y, Zhang Y, et al. (2020a). Ratiometric delivery of doxorubicin and berberine by liposome enables superior therapeutic index than DoxilⓇ. Asian J Pharm Sci 15:385–96.
- Zhang S, Guo W. (2021). β-elemene enhances the sensitivity of osteosarcoma cells to doxorubicin via downregulation of peroxiredoxin-1. Onco Targets Ther 14:3599–609.
- Zhang T, Xiong H, Ma X, et al. (2021b). Supramolecular tadalafil nanovaccine for cancer immunotherapy by alleviating myeloid-derived suppressor cells and heightening immunogenicity. Small Methods 5:e2100115.
- Zhang X, Huang J, Yu C, et al. (2020b). Quercetin enhanced paclitaxel therapeutic effects towards PC-3 prostate cancer through ER stress induction and ROS production. Onco Targets Ther 13:513–23.
- Zhang X, Li L, Liu Q, et al. (2019c). Co-delivery of rose bengal and doxorubicin nanoparticles for combination photodynamic and chemo-therapy. J Biomed Nanotechnol 15:184–95.
- Zhang X, Pei Z, Chen J, et al. (2016c). Exosomes for immunoregulation and therapeutic intervention in cancer. J Cancer 7:1081–7.
- Zhang XK, Wang QW, Xu YJ, et al. (2021c). Co-delivery of cisplatin and oleanolic acid by silica nanoparticles-enhanced apoptosis and reverse multidrug resistance in lung cancer. Kaohsiung J Med Sci 37:505–12.
- Zhang Y, Xiao C, Li M, et al. (2013). Co-delivery of 10-hydroxycamptothecin with doxorubicin conjugated prodrugs for enhanced anticancer efficacy. Macromol Biosci 13:584–94.
- Zhang Y, Yang C, Wang W, et al. (2016d). Co-delivery of doxorubicin and curcumin by pH-sensitive prodrug nanoparticle for combination therapy of cancer. Sci Rep 6:21225.
- Zhang Z, Xu S, Wang Y, et al. (2018b). Near-infrared triggered co-delivery of doxorubicin and quercetin by using gold nanocages with tetradecanol to maximize anti-tumor effects on MCF-7/ADR cells. J Colloid Interface Sci 509:47–57.
- Zhao MD, Li JQ, Chen FY, et al. (2019). Co-delivery of curcumin and paclitaxel by "core-shell" targeting amphiphilic copolymer to reverse resistance in the treatment of ovarian cancer. Int J Nanomedicine 14:9453–67.
- Zhao X, Chen Q, Li Y, et al. (2015a). Doxorubicin and curcumin co-delivery by lipid nanoparticles for enhanced treatment of diethylnitrosamine-induced hepatocellular carcinoma in mice. Eur J Pharm Biopharm 93:27–36.
- Zhao Y, Cai C, Liu M, et al. (2020a). Drug-binding albumins forming stabilized nanoparticles for co-delivery of paclitaxel and resveratrol: in vitro/in vivo evaluation and binding properties investigation. Int J Biol Macromol 153:873–82.
- Zhao Y, Chen F, Pan Y, et al. (2015b). Nanodrug formed by coassembly of dual anticancer drugs to inhibit cancer cell drug resistance. ACS Appl Mater Interfaces 7:19295–305.
- Zhao Y, Huan ML, Liu M, et al. (2016). Doxorubicin and resveratrol co-delivery nanoparticle to overcome doxorubicin resistance. Sci Rep 6:35267.
- Zhao Y, Wang K, Zheng Y, et al. (2020b). Co-delivery of salinomycin and curcumin for cancer stem cell treatment by inhibition of cell proliferation, cell cycle arrest, and epithelial-mesenchymal transition. Front Chem 8:601649.
- Zheng D, Zhao J, Li Y, et al. (2021a). Self-assembled pH-sensitive nanoparticles based on Ganoderma lucidum polysaccharide–methotrexate conjugates for the co-delivery of anti-tumor drugs. ACS Biomater Sci Eng 7:3764–73.
- Zheng S, Wang J, Ding N, et al. (2021b). Prodrug polymeric micelles integrating cancer-associated fibroblasts deactivation and synergistic chemotherapy for gastric cancer. J Nanobiotechnology 19:381.
- Zhu Y, Wen LM, Li R, et al. (2019). Recent advances of nano-drug delivery system in oral squamous cell carcinoma treatment. Eur Rev Med Pharmacol Sci 23:9445–53.
- Zou L, Wang D, Hu Y, et al. (2017). Drug resistance reversal in ovarian cancer cells of paclitaxel and borneol combination therapy mediated by PEG-PAMAM nanoparticles. Oncotarget 8:60453–68.
- Zucker D, Barenholz Y. (2010). Optimization of vincristine-topotecan combination–paving the way for improved chemotherapy regimens by nanoliposomes. J Control Release 146:326–33.