Abstract
Thermal properties of new extruded products with texturized properties were examined using Differential Scanning Calorimetry (DSC). Corn-texturized protein systems were extrusion-cooked in a twin-screw extruder with varying feed rate (0.7–1.9 g/s), screw speed (150–250 rpm), extrusion temperature (150 to 260°C), and feed moisture content (13 to 19 g/100g wet basis). Four different texturized legume flours, namely bean, chickpea, kidneybean, and lentil were mixed with corn grits. A simple mathematical model was used to correlate the melting transition temperature with the extrusion conditions and moisture content. As expected, phase transition behaviour and thermal properties of the corn-water-legume system depend significantly on product temperature, feed moisture and corn/legume ratio and less on screw speed and feed rate.
INTRODUCTION
The extrusion of food materials is a well-known continuous processing unit operation, which is applied in the food industry to produce a wide variety of expanded food products, such as snacks, breakfast cereals, pet food, and pellet fish feeds.[Citation1,Citation2,Citation3,Citation4,Citation5] During extrusion, starchy or proteinaceous foods with moisture content ranging from 15 to 35% are subjected to high temperature, high pressure, and intensive mechanical shear forces. Under these conditions, the biopolymer-based raw materials are converted in a heated barrel into viscoelastic melts, which are further forced to flow through a die. Due to the pressure drop across the die and the subsequent conversion of high-temperature water to steam, the molten stream at the exit expands dramatically to give the desired expanded/cooked product.
The quality of the final product depends on both the feed material properties and the extrusion processing parameters, including extruder type, screw configuration, temperature and shear profile along the extruder, moisture content, screw speed and feed rate. Some of these processing variables are linked, which increases the complexity of the process. The influence of extrusion processing variables on product quality of starch-based materials has been studied extensively.[Citation6,Citation7,Citation8,Citation9,Citation10,Citation11,Citation12]
Starchy foods represent the major source of carbohydrate in the human diet, comprising 80% of the global average calorie intake.[Citation13,Citation14] Starch is also the main component in extruded products including food, feed, degradable plastics, and packaging foams.[Citation15] It is produced as granules in almost all plant cells as an inert source of energy. Though the size and composition of native starch granules depend on the botanical source, they are mainly composed of a mixture of amylose and amylopectin with small amounts of lipids, proteins, and trace amounts of organic and inorganic substances. Amylose exists in an amorphous state and consists of straight chains of α-glucose units with molecular weight from 105 to 106 g.mol−1, corresponding to a few hundred glucose molecules. Amylopectin, the major component of most starches, is composed of branched chains of glucose. The molecules are very large, with molecular weights varying from 107 to 109 g.mol−1 corresponding to about a million glucose units. It exists only in the crystalline layers, which are subdivided into semi-crystalline and amorphous lamellae.[Citation13] Native corn starch consists of approximately 25% amylose and 75% amylopectin.
During extrusion, starch is subjected to high shear, pressure and temperature, leading to the modification of all structural features. The starch granules are ruptured by shear and heat, and the crystalline regions of the granule are melted.[Citation16,Citation17,Citation18,Citation19] Amylose and amylopectin chains are depolymerised and rearranged before exiting the extruder die. In the presence of water, shorter amylose and amylopectin chains are reassociated by hydrogen bonds after exiting the die.[Citation20] Depending on the processing conditions new structures may appear, such as amylose/lipid complexes in crystalline or amorphous state.
Differential Scanning Calorimetry (DSC) is a powerful thermal analysis technique widely used to investigate thermal properties and phase transition of biopolymers. Endothermal and exothermal changes in a DSC thermograph reveal transitions or reactions occurring during DSC testing, such as glass transition, gelatinisation, and melting. Thus, DSC can directly provide the temperature and enthalpy of transition/reaction, as well as heat capacities.[Citation21] By correlating the melt transition temperature of raw materials with characteristics of the finished products, feed manufacturers can predict how much additional thermal processing is necessary to yield desired specific product characteristics.[Citation15]
The interaction between starch and lipids is a well-known phenomenon in the food industry which is used to improve product quality. Eliasson[Citation22] has investigated the starch-lipid interactions by DSC and indicated the formation of amylose-lipid complexes confirming results of previous studies. However, based on indirect evidence, the formation of an amylopectin-lipid complex has also been suggested. Food legumes are characterised by a relatively large content of proteins and carbohydrates. In general, pulses also contain significant amounts of crude fibre, lipids, minerals, and vitamins.[Citation23] Under certain conditions, heat treatment of food results in physicochemical changes in proteins, starch, and in the other components of legume seeds, affecting their final nutritional properties.[Citation24] Alonso et al.[Citation23] have investigated the effects of extrusion on antinutrients and in vitro digestibility of protein and starch in fava and kidney beans; they indicated that extrusion was more effective in improving protein and starch digestibility when compared with dehulling, soaking, and germination.
Barron et al.[Citation25] investigated the structural modifications of low-hydrated pea starch subjected to high thermomechanical processing, using a preshearing rheometer to simulate extrusion cooking. A variety of methods including optical microscopy, X-Ray Diffraction, and DSC were applied to examine physicochemical transformations. It was indicated that incomplete transformations occur at lower temperatures, whereas the crystalline structure is greatly modified, showing partial melting with a higher sensitivity of the B-type crystalline structure. Melting of starch granules began near the hylum without swelling, and no mechanical effects of shearing were observed on granular or crystalline structures.
In many applications native materials are often chemically modified since their properties are not optimal.[Citation26] Nowadays, market tendencies press the producers toward more natural food components, avoiding chemical treatments as much as possible. Therefore, new ways of improving the properties of natural starches excluding the use of chemical compounds were investigation.[Citation27] One possibility which is investigated in the present study is the mixing of different legume flours with corn starch.
The objective of this article was to examine the influence of extrusion parameters, i.e., temperature, legumes content in the feed, moisture content, screw speed, and feed rate on melting temperature and heat, determined by DSC measurements of extrudates. A mathematical model developed by Krokida and Maroulis[Citation28] was applied to identify the most significant extrusion parameters influencing melting temperature and heat.
MATERIALS AND METHODS
Materials
Commercially available legume seeds (chickpea, beans, kidney beans, and lentils), as well as corn meal were used in this study. Seeds were ground and sieved to grain size lower than 0.5 mm using a Hermetic Peraga grinding mill and then stored at 4°C for extrusion experiments. Before mixing, the moisture content of raw materials was determined. A predefined amount of ground legumes was mixed with corn flour, salt, and deionized water, to obtain a final mixture of specific corn to total dry weight ratio, 0.5% salt, and specific moisture content. The ingredients were blended in a mixer at least 24 h before extrusion and the samples were stored at 25°C in plastic bags in order to equilibrate. Before extrusion, the moisture content of the mixtures obtained was measured and, wherever needed, it was corrected by adding deionized water.
Extrusion Cooking
A laboratory scale food extruder (Prism Eurolab extruder, model KX-16HC) equipped with conical, counter-rotating twin screw was used. Screws of 1 m length and 16 mm diameter rotate at a maximum speed of 500 rpm. The pressure at the die (2 mm in diameter), was measured during extrusion with a pressure transducer. A twin screw volumetric feeder was used for the material feeding into the extruder at a controlled rate. The extruder has 5 temperature control zones. The product temperature during extrusion was adjusted by varying the temperature in the barrel and die. All extrusion variables were displayed on the control panel (barrel and die temperatures, pressure, and screw speed).
The independent extrusion variables considered were varied within the following ranges: feed moisture content from 13 to 19 g/100 g (wet basis), corn powder to total dry weight ratio 10–90%, product temperature from 150 to 260°C, feed rate from 0.7 to 1.9 g/s, and rotation speed from 150 to 250 rpm. Steady state extrusion conditions were reached after 20 min. The steady-state samples were then collected, dried in air, and stored for DSC measurements.
DSC Measurements
DSC measurements were performed with a Perkin-Elmer Differential Scanning Calorimeter (DSC 6, Norwalk, CT, USA) on all produced extrudates to study their main thermal properties, including melting temperature and maximum heat flow at melting temperature. About 10 mg of the dried samples (<2 % moisture) were placed in stainless steel DSC pans and the pans were sealed. The instrument was calibrated with indium. The samples were heated from 20° to 250°C with a heating rate of 10°C/min in a nitrogen atmosphere. The melting temperature (T m ) and the maximum heat flow rate (H m ) were taken as the coordinates of the peak value of the endothermal process in the DSC curves.
MATHEMATICAL MODELING
A simple mathematical model has been proposed to predict thermal properties of extruded materials as a function of water and corn content as well as extrusion process characteristics.[Citation28] The proposed model is summarized on . In order to examine the influence of material and process characteristics on the melting transition temperature (T m ), a power model was used, in which n o is a constant and n F , n T , n r , n X, and n P are the exponents of feed rate, temperature, screw speed, feed moisture content, and legume (proteinaceus food) content, respectively. It is expected that not all of these parameters will influence the thermal properties of extruded products to the same degree and this may be revealed through regression analysis.
Table 1 Mathematical model
The values of the required parameters can be determined by fitting the proposed model to the experimental data. This can be done by minimizing the following residual sum of squares:
where T
m
and are the experimental and the predicted values of melting point, respectively, N is the number of experimental points, and p is the number of estimated parameters. Following a suitable regression analysis procedure, all six parameters (n
o
, n
F
, n
T
, n
r
, n
X and n
P
) can be determined simultaneously. However, it is not likely that all of these parameters affect the residual sum of squares (S) to the same degree. In order to distinguish between the ones that are necessary to accurately predict the thermal properties, the following procedure was adopted: firstly, the minimum change in sum of squares was evaluated for all six parameters; secondly, omitting one parameter at a time, the value of S was evaluated for all combinations of the remaining five parameters. In this way, the parameter chosen to be eliminated was the one whose elimination produced the minimum sum of squares. Continuing the former procedure, the minimum sum of squares was evaluated for 5, 4, 3, 2, and 1 parameters, respectively. Obviously, the best value of minimum sum of squares is the one that involves all parameters. However, comparing the values of sum of squares obtained by reducing the number of parameters, one can locate an optimum that gives an acceptable accuracy.
RESULTS AND DISCUSSION
Typical diagrams of DSC data are presented in , from which a database with melting points and heat data were extracted, for all the examined materials and experimental conditions (). The regression analysis procedure showed that the optimum number of parameters producing a reasonable minimum residual sum of squares is four. The use of fewer parameters increases dramatically the minimum sum of squares (). These four parameters were found to be n o , n T , n X and n P .
Figure 1 DSC thermograms of corn-legumes mixtures (the arrow indicates endothermic heat flow. Corn content: 50%; extrusion temperature: 200°C; screw speed: 200 rpm; moisture content: 16%; feed rate: 1.3 g/s).
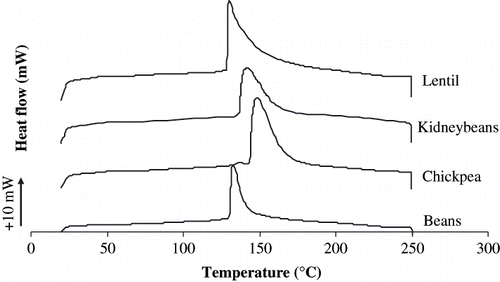
Table 2 Extrusion parameters
The results of fitting the proposed thermal properties predictions into the experimental data are shown in to . The corresponding parameter estimates for all materials are shown on . In , the melting point temperatures as a function of extrusion temperatures for various moisture contents. Solid lines are used to plot the calculated values of melting temperature using the mathematical model and the parameters of . The fitting of the proposed model to the experimental data is considered satisfactory. In , the melting point is presented are presented as a function of corn content in the corn/legume mixture for various feed rates.
Table 3 Results of parameter estimation
The same regression analysis was performed for the maximum heat flow values obtained at the melting temperature, and it was found to be affected by the same extrusion parameters. The corresponding maximum heat flow is presented as a function of extrusion temperature and moisture content (), and corn content in the corn/legume mixture for various feed rates ().
Generally, rotation speed and feed rate do not seem to significantly affect the thermal properties of the extruded products. The melting temperature decreased slightly as the corn content increased for all the feed rates and examined materials. It also decreased by increasing the extrusion temperature and feed moisture content (). These changes are attributed to molecular changes in starch and proteins during extrusion cooking. In the extrusion of large biopolymers, mechanical degradation can occur due to the substantial shear stress when the viscosity of the material is high at low temperatures and/or low moisture content. Under these conditions an increase of screw rotation speed results in increased depolymerization of contained biopolymers and, consequently, in lower melting temperatures. At the higher temperatures applied in this study, well above the corn and legumes melting temperatures, the viscosity of the material is lower, and consequently, mechanical degradation is less pronounced, while the kinetics of thermal degradation become more favorable.[Citation29] For this reason, the effect of screw rotation rate on melting temperature was not significant, and the melting temperature decreased with the increase of process temperature.
The effect of feed rate on both melting temperature of the extrusion products and the respective heat flow was also insignificant. The feed rate affects mechanical stresses in the extruder, which are weak due to the low viscosity of the material at the elevated temperatures applied, and the residence time which seems to be higher than the time required for thermal degradation of the biopolymers at the process conditions. As it is stated by many authors, when the moisture content of the material is limited, the viscosity and, consequently, the mechanical shear forces increase, resulting in a decrease in the components' molecular weight[Citation30] and thus in the melting temperature of the extrusion products. On the other hand, when the moisture content is high, gelatinization reactions may lead to a decrease in the components' average molecular weight and, consequently, in melting temperature; bean, kidneybean, and lentil mixtures of corn follow this trend, whereas high melting temperatures were measured for low moisture content when using a mixture of chickpea and corn flours as feed material.
CONCLUSIONS
The effect of extrusion conditions, i.e., temperature, feed moisture, and corn content, feed rate, and rotation speed, on the thermal properties of extruded corn-legume products was investigated. The melting temperature decreased by increasing corn content and extrusion temperature, due to molecular changes in starch and proteins during extrusion cooking. On the other hand, the increase of feed moisture content resulted in a significant decrease of melting temperature in 3 out of 4 systems examined; the opposite trend was found when using a mixture of chickpea and corn flour as a feed material. Rotation speed and feed rate do not seem to significantly affect the thermal properties of the extruded products, since the viscosity of the material is low at the high temperatures applied and, consequently, mechanical degradation is less pronounced, while the kinetics of thermal degradation becomes more favorable. The maximum heat flow at the melting temperature also decreased with extrusion temperature but increased with increasing moisture content or corn content in the corn-legume mixture.
NOMENCLATURE
S | = |
Sum of squares (°C) | ||||||||||||||||||||
N | = |
Number of experimental points | ||||||||||||||||||||
p | = |
Number of estimated parameters | ||||||||||||||||||||
T m | = |
Melting temperature (experimental value) (°C) | ||||||||||||||||||||
H m | = |
Heat flow (mW) | ||||||||||||||||||||
| = |
Melting temperature (predicted value) (°C) | ||||||||||||||||||||
T | = |
Extrusion temperature (°C) | ||||||||||||||||||||
r | = |
Screw speed (rpm) | ||||||||||||||||||||
X | = |
Feed moisture content (g/100 g wet basis) | ||||||||||||||||||||
F | = |
Feed rate (g/s) | ||||||||||||||||||||
C | = |
Corn content (ration of corn to corn-legume mixture) (g/100 g dry basis)
|
ACKNOWLEDGMENTS
The authors acknowledge the Ministry of Education and the European Commission for co-funding this study in the framework of EPEAEK II—PYTHAGORAS programme.
Notes
Hoseney, R.C.; Mason, W.R.; Lai, C.-S.; Guetzlaff, J. Factors Affecting the Viscosity and Structure of Extrusion-cooked Wheat Starch. In J. L. Kokini, C. Ho, & M.V. Karwe (Eds.), Food Extrusion Science and Technology; Kokini, J.L.; Ho, C.; Karwe, M.V.; Eds.; Marcel Dekker: New York, 1992; 277–305.
REFERENCES
- Harper , J.M. 1989 . “ Food Extruders and Their Application ” . In Extrusion Cooking , Edited by: Harper , J.M. , Mercier , C. and Linko , P. 1 – 15 . Minnesota : American Association of Cereal Chemists, Inc .
- Miller , R.C. 1990 . “ Unit operations and equipment IV. Extrusion and extruders ” . In Breakfast cereals and how they are made , Edited by: Fast , R.B. and Galdwell , E.F. 135 – 193 . St. Paul, MN : American Association of Cereal Chemists .
- Rahman , S. 1995 . Food Properties Handbook , New York : CRC Press .
- Chauhan , G.S. , Sharma , P. and Bains , G.S. 2003 . Effect of Extrusion Cooking on X-Ray Diffraction Characteristics of Rice and Rice-legume Blends . International Journal of Food Properties , 6 ( 1 ) : 127 – 133 .
- Sharma , H.R. , Chauhan , G.S. and Agrawal , K. 2004 . Physicochemical Characteristics of Rice Bran Processed by Dry Heating and Extrusion Cooking . International Journal of Food Properties , 7 ( 3 ) : 603 – 614 .
- Barres , C. , Vergnes , B. , Tayeb , J. and Della Valle , G. 1990 . Transformation of Wheat Flour by Extrusion Cooking: Influence of Screw Configuration and Operating Conditions . Cereal Chemistry , 67 : 427 – 433 .
- Bhattacharya , M. and Hanna , M.A. 1987 . Textural Properties of Extrusion Cooked Corn Starch . Lebensmittel-Wissenschaft und Technologie , 20 : 195 – 201 .
- Kirby , A.R. , Ollet , A.L. , Parker , R. and Smith , A.C. 1988 . An Experimental Study of Screw Configuration Effects in the Twin Screw Extrusion Cooking of Maize Grits . Journal of Food Engineering , 8 : 247 – 272 .
- Mason , W.R. and Hoseney , R.C. 1986 . Factors Affecting the Viscosity of Extrusion Cooked Wheat Starch . Cereal Chemistry , 63 : 436 – 451 .
- Gujral , H.S. and Singh , N. 2002 . Extrusion Behaviour and Product Characteristics of Brown and Milled Rice . International Journal of Food Properties , 5 ( 2 ) : 307 – 316 .
- Guha , M. and Zakiuddin Ali , S. 2002 . Molecular Degradation of Starch During Extrusion Cooking of Rice . International Journal of Food Properties , 5 ( 3 ) : 509 – 521 .
- Adamu , A. and Jin Yu , Z. 2002 . Effect of Chemical Agents on Physical and Rheological Properties of Starch Guar Gum Extrudates . International Journal of Food Properties , 7 ( 3 ) : 261 – 275 .
- Cheyne , A. , Barnes , J. , Gedney , S. and Wilson , D.I. 2005 . Extrusion Behaviour of Cohesive Potato Starch Pastes: II. Microstructure-process Interactions . Journal of Food Engineering , 66 : 13 – 24 .
- Food and Agriculture Organisation of the United Nations . 1999 . Production Yearbook Volume 1988 , FAO Statistics Series No. 148 Vol. 53 , Rome : FAO .
- Blanche , S. and Sun , X. 2004 . Physical Characterisation of Starch Extrudates as a Function of Melting Transitions and Extrusion Conditions . Advances in Polymer Technology , 23 ( 4 ) : 277 – 290 .
- Owusu-Ansah , J. , van der Voort , F.R. and Stanley , D.W. 1983 . Physicochemical Changes in Cornstarch as a Function of Extrusion Variables . Cereal Chemistry , 60 : 319 – 324 .
- Davidson , V.J. , Paton , D. , Diosady , L.L. and Laroque , G. 1984 . Degradation of Wheat Starch in a Single Screw Extruder: Characteristics of Extruded Starch Polymers . Journal of Food Science , 49 : 453 – 458 .
- Davidson , V.J. , Paton , D. , Diosady , L.L. and Rubin , L.J. 1984 . Model for Mechanical Degradation of Wheat Starch in a Single Screw Extruder . Journal of Food Science , 49 : 1154 – 1157 .
- Gomez , M.H. and Aguilera , J.M. 1983 . Changes in the Starch Fraction During Extrusion Cooking of Corn . Journal of Food Science , 48 : 378 – 381 .
- Guan , J. and Hanna , M.A. 2004 . Extruding Foams from Corn Starch Acetate and Native Corn Starch . Biomacromolecules , 5 ( 6 ) : 2329 – 2339 .
- Zhong , Z. and Sun , X.S. 2005 . Thermal Characterisation and Phase Behaviour of Cornstarch Studied by Differential Scanning Calorimetry . Journal of Food Engineering , 69 : 453 – 459 .
- Eliasson , A.C. 1994 . Interactions between Starch and Lipids Studied by DSC . Thermochimica Acta , 246 : 343 – 356 .
- Alonso , R. , Aguirre , A. and Marzo , F. 2000 . Effects of Extrusion and Traditional Processing Methods on Antinutrients and in Vitro Digestibility of Protein and Starch in Faba and Kidney Beans . Food Chemistry , 68 ( 2 ) : 159 – 165 .
- Della Valle , G. , Quillien , L. and Gueguen , J. 1994 . Relationships between Processing Conditions and Starch and Protein Modifications during Extrusion-cooking of Pea Flour . Journal of the Science of Food and Agriculture , 64 : 509 – 517 .
- Barron , C. , Bulleon , A. , Colonna , P. and Della Valle , G. 2000 . Structural Modifications of Low Hydrated Pea Starch Subjected to High Thermomechanical Processing . Carbohydrate Polymers , 43 : 171 – 181 .
- Jacobs , H. and Delcour , J.A. 1998 . Hydrothermal Modifications of Granular Starch, with Retention of the Granular Structure: A Review . J. Agric. Food Chem. , 46 : 2895 – 2905 .
- Ortega-Ojeda , F.E. and Eliasson , A-C. 2001 . Gelatinisation and Retrogradation Behaviour of Some Starch Mixtures . Starch/Stärke , 53 : 520 – 529 .
- Krokida , M.K. and Maroulis , Z.B. 1997 . Effect of Drying Method on Shrinkage and Porosity . Drying Technology , 10 ( 15 ) : 1145 – 1155 .
- Hoseney, R.C.; Mason, W.R.; Lai, C.-S.; Guetzlaff, J. Factors Affecting the Viscosity and Structure of Extrusion-cooked Wheat Starch. In J. L. Kokini, C. Ho, & M.V. Karwe (Eds.), Food Extrusion Science and Technology; Kokini, J.L.; Ho, C.; Karwe, M.V.; Eds.; Marcel Dekker: New York, 1992; 277–305.
- Karathanos , V.T. and Saravacos , G.D. 1992 . “ Water Diffusitivity in the Extrusion Cooking of Starch Materials ” . In Food Extrusion Science and Technology , Edited by: Kokini , J.L. , Ho , C. and Karwe , M.V. 177 – 183 . New York : Marcel Dekker .