Abstract
Rheological properties of single-phase, and emulsions containing modified starch and gum arabic as surface active hydrocolloids, as well as xanthan and tragacanth gums as stabilizers were evaluated under steady and dynamic shear testing conditions using a control stress rheometer. Emulsions were formed by 9% and 14% gum concentrations with oil concentration maintained at 9% thus giving a 1:1 and 1.5:1 surface active agent to oil ratio, respectively. The rates of droplet coalescence and creaming, for a total of 8 emulsions, as a function storage time before and after dilution in a simulated fruit beverage were then investigated. Steady shear (flow curve) were well described by the Carreau model at shear stress ranging from 0.01 to 100 Pa. All prepared water phases indicated a zero-shear viscosity plateau followed by shear thinning behavior with flow behavior index (n) ranging from 0.51 to 0.79 for 14% starch-0.3% xanthan and 14% gum arabic-0.8% tragacanth stabilized emulsions, respectively. The water phase flow property data were well fitted by the Einstein equation and its expansions. The dynamic rheological properties of water phase and emulsions were also evaluated for G′(ω) and G″(ω) from 1 to 50 rad/s. Similar curves were obtained with varying degrees of deviations (G′ from G″) for different emulsions. Starch-xanthan emulsion and associated water phase at 1.5/1 agent to oil ratio demonstrated viscoelastic behavior (G′ ≥ G″) with lower droplet coalescence and creaming rates. On the other hand, gum arabic-xanthan emulsion at 1:1 agent to oil ratio showed the highest rate of droplet coalescence and a greater degree of creaming. It was speculated that the lower stability of gum arabic-xanthan emulsion could be related to the denaturation of proteinaceous part in the gum and loss of emulsification capacity due to lower pH and pasteurization.
INTRODUCTION
A stable beverage emulsion is characterized by long-term stability in the diluted form, or absence of ringing resulting from raised coalesced droplets around the neck of the beverage bottle. Since ban and/or restriction on use of density adjusting weighting agents in 1970 for producing cloud or flavor emulsions, their stability over the desired shelf life has become a common problem in the beverage industry.[Citation1–6] Beverage cloud emulsions are oil-in-water emulsions comprising of vegetable oil and a combination of weighting agents to provide opacity, and increase the density of the oil phase, respectively. The water phase is formed by water and amphiphilic polysaccharides to reduce surface tension and grant steric stabilization, added stabilizer gums prolong the stability and control rheological properties and citric acid is used to adjust the acidity.
Several studies concerning emulsion stability have indicated that the use of a proper polysaccharide in the water phase could prevent the breakdown of the emulsion and it will seldom reach the coalescence stage.[Citation4,Citation7–9] Furthermore, to perform as an effective stabilizer for beverage emulsion, the surface active hydrocolloids must be readily soluble in cold water, and should have a low viscosity in water (to cover the droplet surfaces by a sufficiently high concentration of hydrocolloid), a high emulsifying capacity, and should not thicken or gel on aging.
Gum arabic, which has been known as the most surface active hydrocolloid gum, is highly soluble in cold water and its solubility increases at higher temperatures. Because of its effective emulsifying capacity and the well known film forming ability gum arabic has been widely used in the soft drink industry for emulsifying flavor oils under acidic conditions. It is mainly produced from the species Acacia Senegal—Acacia Seyal produces the rest. The surface activity is due to the branched arabino-galactan blocks attached to a polypeptide backbone. Chanamai and McClements[Citation3] reported that the hydrophobic polypeptide chain anchor the molecules to the droplet surface and the hydrophilic arabino-galactan blocks extend into the solution, providing stability against droplet aggregation by steric stabilization and electrostatic repulsion. Buffo et al.[Citation9] and Dickinson[Citation10] indicated that gum arabic is an indisputable emulsifier that grants functionality not by modifying the rheology of the water phase but by leading the formation of a macromolecular stabilizing layer around the oil droplets. Despite its functionality, gum arabic is a fairly expensive ingredient and there have been many attempts for its replacement by hydrophobically modified starch or other gums.
In this study, we also used a modified starch (Purity gum 2000; National Starch, Bridgewater, NJ) known as octenyl-succinated starch or OSA. It is made by esterification of starch with anhydrous octenyl-succinic acid under alkaline conditions.[Citation11] It consists primarily of amylopectin that has been modified to contain non-polar side-groups. These side-groups anchor the molecule to the droplet surface, while the hydrophilic starch chains stick out into the aqueous phase and protect droplets against aggregation through steric repulsion. The Purity gum 2000 is mildly anionic in aqueous solutions and has a surface activity that is almost as high as gum arabic.[Citation3] Therefore, polysaccharides such as amphiphilic gum arabic or hydrophobically modified starch serve as the surface active and steric stabilizer in the cloud emulsions, while xanthan and tragacanth gums could control the rheological properties of the emulsions.[Citation2–4,Citation12–14] Xanthan gum is a microbial exocellular polysaccharide approved by FDA for use in food. Tragacanth gum, a Persian gum, is a complex mixture of polysaccharides, mostly poly-D-galacturonic acid and bassorin. A part of it is miscible and a part forms a gel of exceptional quality.[Citation15] Both xanthan and Tragacanth gums have been recognized as cold water soluble gums with good stability to salt and acid.[Citation11,Citation16]
Several studies have attempted to correlate the rheological properties and stability of emulsions.[Citation17–19] Tempel and Van Den[Citation20] reported that many hydrocolloids used to stabilize emulsion are shear-thinning, and they indicated that information about the size of the aggregates and the strength of the bonds between flocculated droplets can be determined from steady state flow tests over a large range of shear rates. Xie and Hettiarachchy[Citation21] studied the effect of xanthan gum on emulsifying properties of soy protein isolate. They mentioned that xanthan gum contributed to the emulsion stability by its adsorption at the oil-water interface which resulted in decreasing the surface tension as well as formation of liquid crystalline lamellae in the water phase to trap the oil droplets in the micro-gel matrix. A study by Chen and Dickinson[Citation22] on effect of emulsifiers on the viscoelastic properties of heat-set whey protein emulsion gel revealed that by decreasing the mean droplet size the adsorbed polymer stabilizing layer gives rise to an effectively larger volume fraction and produce a gel of higher elastic modulus. Buffo and Reineccius[Citation23] indicated that an appropriate stability of concentrated beverage emulsion could be achieved by adjusting the viscosity of the system through the hydrocolloid concentration. Dickinson[Citation10] pointed out that emulsions with low volume fraction (5–10%) favor the destabilization by flocculation when the aqueous phase contains a non-gelling hydrocolloid or gelling hydrocolloids at a concentration below the gelation threshold. Paraskevopoulou et al.[Citation13] studied the stability of olive oil-lemon juice emulsion stabilized by xanthan gum as stabilizer and gum arabic and propylene glycol alginate as emulsifiers, and they emphasized the importance of continuous phase rheology in determining the rheological and creaming behavior of emulsions. Batista et al.[Citation24] studied the rheological properties of added lutein and phycocyanin in colored oil-in-water emulsions and highlighted the contribution of each component on the quality of formed emulsions.
The production of high-quality food emulsions, consequently, depends on the knowledge of the contribution of the components both individually and in combination on the properties of the end product.[Citation5] So far most studies on stabilizing beverage emulsions have aimed at using different weighting agents; but those dealing with the shelf life of beverage emulsions without weighting agents are limited. The objective of this study was to investigate the effect of the viscosity modifiers (hydrocolloid gums) and surface active polysaccharides, which have the affinity to anchor to the oil droplet surface (modified starch and arabic gum) on the rheology and stability of beverage emulsions both in the concentrated and dilute (simulated juice drink) forms in the absence of weighting agents.
Emulsion Rheology
For dilute suspensions of rigid spherical particles, the Einstein equation [Eq.(1)] and its expansions have been widely used:[Citation5,Citation6,Citation17,Citation23,Citation25,Citation26,Citation27,Citation28]
where η Em is viscosity of emulsion; η w viscosity of water phase; and φ is the volume fraction. This equation covers suspensions containing continuous phase (water phase) of Newtonian behavior and rigid particles. For the dilute suspensions of fluid spherical particles the modified Einstein Equation is given by McClements.[Citation5]
For concentrated suspensions of non-flocculated particles and in the absence of long-range colloidal interactions the following two equations have been given to define the relative viscosity of concentrated beverage emulsions:
The value of the constants a, b, c, and . . . can be determined either experimentally or theoretically. The values a and b for rigid particles were established as 2.5 and 6.2, respectively.[Citation5,Citation23] In the presence of long-range repulsive interactions (electrostatic, steric) where the droplets are prevented to become close together and collide which results in increasing the effective volume fraction of the droplets – a modified Dougherty and Krieger equation has been suggested:[Citation23]
where the δ is half the distance of closest separation between the droplets and for steric stabilization, which is approximately equal to the thickness of adsorbed layer. Buffo et al.[Citation9] reported that determination of layer thickness based on viscosity is prone to error due to different degree of poly-dispersity and packing density at the oil/water interface among the samples, as well as dissimilar electrolyte contents which can reduce the effective volume fraction. Later, Buffo and Reineccius[Citation23] justified the assumption of layer thickness to be one eighth of the particle radius: δ = r/8 and δ eff = 2.68δ. Therefore,
In term of complex shear modulus (G∗) and in the linear viscoelastic region, the following equation is suggested by:[Citation19,Citation29]
where is the complex shear modulus of water phase and G∗ defined as
where G′ is the storage modulus, G″ is the loss modulus, and j is the complex number. Pal[Citation29] developed another equation for suspensions of rigid particles as
Considering oil particles are rigid
In the present study, the theoretical models of emulsion viscosity for concentrated beverage emulsions consisting of different surface active hydrocolloids and identical volume ratio were compared. The complex shear moduli of emulsions were also compared to EquationEq. (21)(9).
MATERIALS AND METHODS
Materials
Coconut oil was obtained from Univar and Daminco Inc. (Boucherville, Quebec) and modified starch (Purity Gum 2000) from National Starch and Chemical (Bridgewater, NJ). Spray dried arabic and xanthan gums (TIC PRETESED® XANTHAN 200) were provided by Nealanders Inc. (Montreal, Quebec). Tragacanth gum was kindly granted by Hydrotech Inc. (Teheran, Iran). Food grade citric acid and potassium sorbate were used to adjust the acidity and reduce the chance of contamination in all prepared emulsions.
Preparation of Emulsions
Modified starch and gum arabic as surface active emulsifying agents and xanthan and tragacanth as stabilizing agents were dissolved separately in distilled water and stored overnight for completing hydration. After hydration, the pH of water phase was adjusted through addition of 1% weight/weight (w/w, based on total emulsion weight) of citric acid in form of 50% solution. After addition of a constant amount of coconut oil 9% (w/w based on final preparation) into the emulsifier solutions, each sample was stirred for 15 min. The surface active agent concentrations were based on 1:1 and 1.5:1 agent:oil ratio, thus the batches were 9 and 14% agent with 9% oil (all w/w basis final weight). The solution of stabilizer gums were, then, added to the dispersion and followed by a 3 min homogenization at 22000 rpm using Polytron laboratory scale homogenizer (Polytron, PT 10–35, Kinematica, AG Ltd. Switzerland). Further size reduction was obtained by using a laboratory scale high pressure homogenizer (Emulisiflex-C5, Avestin, Ottawa, ON) at 3500 psi and 2 passes.
Particle Size Distribution
The mean particle size and particle size distribution of prepared emulsions were determined using an integrated light scattering technique (ZetaSizer 4, Malvern Instruments Ltd., Malvern, UK). Concentrated beverage emulsions were diluted 1:1000 using DI water to prevent multiple scattering effects[Citation28] and placed in specified glass cuvette for size measurement. Determination of size was done in duplicate at room temperature and right after preparation of each emulsion.
Surface Tension
The Du Nouy ring method was used to determine the surface tension of hydrocolloid gum solutions.[Citation30] A Fisher Surface Tensiomat (Fisher Scientific, Sainte-Foy, Quebec) was employed and the force acting on the ring was measured at room temperature as it was moved upward form 3 mm below the surface of gum-air interface.
Opacity, Specific Gravity, and Volume Fraction
Opacity of diluted emulsions (1:1000) was measured at 660 nm visible range using a spectrophotometer (Cary, 300-Bio, UV-Visible Spectrophotometer, Varian, Australia). Specific gravity of components phases were evaluated at the prepared concentrations using a 25 mL specific gravity bottle [Fisherbrand, Ottawa, ON]. The volume fraction of each emulsion was determined based on following expression:
where Sgw is the specific gravity of water phase, SgEm the specific gravity of emulsion and Sgoil the specific gravity of oil phase.
Rheological Tests
Using a control-stress rheometer (AR2000 Rheometer, TA Instrument, New Castle, DE) equipped with a stainless steel 60 mm cone of 2° and solvent trap. The measurement temperature was constant at 22°C using a circulating bath and a controlled peltier system. Flow and dynamic tests were conducted at the ranges of 0.001–100 Pa and 1–50 rad/sec (using constant stress value of 1 Pa, find from linear viscoelastic region) respectively. Hydrocolloid gums after 24 h hydration and emulsions immediately after being prepared were subjected to rheological measurements.
Physical Stability
Instrumental test
Homogenized emulsion was diluted in acidified sugar solution 2% (w/w), bottled and pasteurized (12 min at 95°C) for stability studies. The pasteurization time was found by installing a semi-rigid thermocouple with the tip located at 1/10 of height of the bottle from the bottom.[Citation31] An aliquot of 6 ml of each pasteurized sample was poured into a flat-bottom cylindrical glass tube (100-mm height, 16-mm internal diameter) and subjected to an optical scanning screening (Quick Scan, Coulter Crop., Miami, FL). The transmission of monochromatic light (λ = 850 nm) from the diluted emulsion was measured as a function of their height in order to quantify the creaming rate. Creaming rate was then calculated from the height of the interfaces between the opaque droplet-rich layer and the transparent droplet-depleted layer as a function of time. Creaming rate was expressed as slope of absolute thickness of layers per unit time.
Observation in bottles
The 2% (w/w) diluted emulsion (simulated beverage) after preparation, bottling and pasteurization for 12 min at 95°C was stored at ambient temperature. The creaming stability was monitored in duplicate with a total of 6 bottles for each prepared emulsion. The samples with presence of whitish rings on the neck of the bottles after a period of one week were graded as +, and ± when creaming was observed after 3 weeks. Simulated beverages containing 2% of emulsions with no sign of creaming after 5 weeks were considered stable with negative sign.
RESULTS AND DISCUSSION
Flow Properties
The selection of concentration for stabilizing agents was based on primary steady shear rheology of xanthan and tragacanth gums at different concentrations. Xanthan gum at 0.3% and Tragacanth gum at 0.8% indicated the comparative consistency coefficients at 886 and 883 mPa, respectively. Xanthan gum, however, had a lower flow behavior index at 0.28 ± 0.02 compared to that of tragacanth gum at 0.53 ± 0.03. Since the measurements were carried out at shear rate that were sufficiently lower than the high shear rate plateau (η∞ → 0), the modified Carreau equation was used to compare the flow properties of different emulsions:[Citation24,32]
where η0 is the limiting viscosity for the first Newtonian region; γ is the shear rate; λ is the time constant calculated from reciprocal of the shear rate at which the zero shear rate component and power-law component of flow curve intersect; and n is the flow behavior index.
The emulsions flow curves for 1:1 and 1.5:1 surface active agent/oil are shown in and , respectively. Both figures represent a shear thinning behavior with a zero-shear rate limiting viscosity (η0) at very low shear rate (γ). The flow curves were identical for the three measurements and a time period of two weeks after preparation. The zero-shear rate limiting viscosity slightly increased for all the emulsions after two weeks, corresponding to the upper limit of the linear viscoelastic range of the emulsion (). Increase in viscosity of emulsions containing tragacanth and xanthan gums as a function of storage time has also been reported by Coia and Stauffer.[Citation16] They assumed that the initial viscosity resulted from the hydration of amorphous region of tragacanth gum containing the crystalline bodies in their solid state. The crystalline bodies that were soluble and more hydrophobic, and could migrate to the interfacial area producing a film around the droplet. The remaining crystalline bodies were unable to migrate the continuous phase and upon standing slowly hydrated producing additional viscosity. It is also possible that increase in zero-shear viscosity partially corresponded to higher degree of flocculation, thus, more stress needed to breakdown the flocs.
Figure 1 Steady-state flow curves for: a) 9% Arabic and 0.8% Tragacanth gums; b) 9% Arabic and 0.3% Xanthan gums; c) 9% modified Starch and 0.8% Tragacanth gums; and d) 9% modified Starch and 0.3% Xanthan stabilized emulsions.
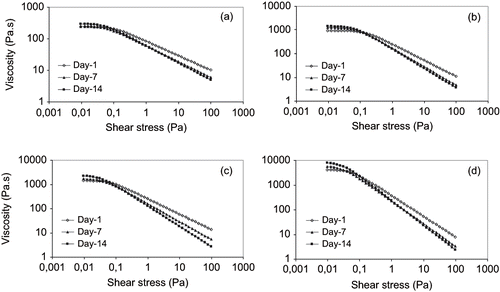
Figure 2 Steady-state flow curve for: (a) 14% Arabic and 0.8% Tragacanth gums; (b) 14% Arabic and 0.3% Xanthan gums; (c) 14% modified Starch and 0.8% Tragacanth gums; and (d) 14% modified Starch and 0.3% Xanthan stabilized emulsions.
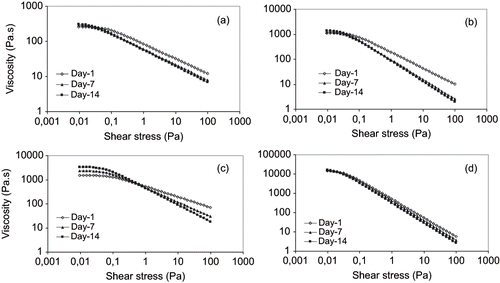
Table 1 Zero-shear viscosity and flow behavior index of emulsions as a function of storage time
The zero-shear viscosity is related to application of shear stresses within the Newtonian plateau producing small deformations which were within the linear viscoelastic range. As a result, the viscosity measured at equilibrium flow corresponded to the Newtonian compliance and as long as the applied shear stress was within the linear viscoelastic range of the emulsion, the measured equilibrium viscosity was constant. Buffo and Reineccius[Citation23] and McClements[Citation5] also indicated that at low shear rates the hydrodynamic forces are not large enough to disrupt the flocs, which then act like particles with fixed size and shape, resulting in a constant viscosity. This increase was more pronounced for emulsions formed by modified starch (purity gum 2000) — tragacanth gum as well as arabic — xanthan gum combinations which could be related to the higher degree of flocculation. As the shear rate is increased and the values of stress exceeded the linear range, the hydrodynamic forces become large enough to deform and disrupt the flocs, which elongate and align with the shear field, and a rapid decline in equilibrium viscosity occurs. The shear thinning index (n) indicated a decrease after two weeks storage time period for all emulsions, the magnitude was different. This decrease was again more articulated for emulsions prepared with modified starch and tragacanth gum and/or arabic and xanthan gum at selected concentrations. The shear thinning region has been reported to be a consequence of a dramatic shear induced structural breakdown, related to the mechanism of oil droplet deflocculation.[Citation5,Citation24] Both findings, therefore, describe the lower stability of these emulsions compared to those prepared with modified starch and xanthan and/or gum arabic and tragacanth gum combinations.
The experimental flow curves consisting of apparent viscosity as a function of shear stress were also compared with Buffo and Reineccius[Citation23] model [Model I, EquationEq. (5)(5)]. This model assumed the reduction of effective volume fraction due to different packing density of gums at the oil/water interface and similar electrolyte content. Since we used the combination of surface active and stabilizer gums, accounting for both hydrodynamic and colloidal interactions, the viscosity was predicted by including additional volume fraction term using extended Einstein equation as:
The flow curves for day 1 and 14 along with model predicted values [EquationEq. (12)(12)] are shown in for stable emulsions and for unstable emulsions. The values of a were found to be 2, 1.8, 1.4, and 0.6 for 14% starch-0.3% xanthan, 14% arabic-0.8% tragacanth, 14% arabic-0.3% xanthan, and 14% starch-0.8% tragacanth, respectively. The values of b were constant at 6.2 for all predictions. As illustrated, Model I over-predicts the viscosity of emulsions, while all flow curves illustrate an excellent fit for predicted model. Stable emulsions after 14 days storage () indicate minor deviations from predicted flow curves. The unstable emulsions () illustrate this deviation along with an increasing zero-shear viscosity. Unstable emulsions, hence, indicated greater extent of flocculation associated with holding the particle together, increasing the resistance to rupture and dramatic decrease of viscosity when the flocs became elongate and aligned with the shear field.
Figure 3 Comparison of experimental steady-state flow curve with Model I [EquationEq. (5)(5)] and that developed in this study [EquationEq. (12)
(12)], for 14% Arabic-0.8% Tragacanth and 14% Starch-0.3% Xanthan stabilized emulsions at a) day 1; b) day 14; c) day 1; and d) day 14, respectively.
![Figure 3 Comparison of experimental steady-state flow curve with Model I [EquationEq. (5)(5)] and that developed in this study [EquationEq. (12)(12)], for 14% Arabic-0.8% Tragacanth and 14% Starch-0.3% Xanthan stabilized emulsions at a) day 1; b) day 14; c) day 1; and d) day 14, respectively.](/cms/asset/6862cb21-d5c0-4af7-8127-3c4b5e91f0ad/ljfp_a_217122_o_f0003g.gif)
Figure 4 Comparison of experimental steady-state flow curve with Model I [EquationEq. (5)(5)] and that developed in this study [EquationEq. (12)
(12)], for 14% Arabic-0.3% Xanthan and 14% Starch-0.8% Tragacanth stabilized emulsions at:a) day 1; b) day 14; c) day 1; and d) day 14, respectively.
![Figure 4 Comparison of experimental steady-state flow curve with Model I [EquationEq. (5)(5)] and that developed in this study [EquationEq. (12)(12)], for 14% Arabic-0.3% Xanthan and 14% Starch-0.8% Tragacanth stabilized emulsions at:a) day 1; b) day 14; c) day 1; and d) day 14, respectively.](/cms/asset/c283caef-c022-4a68-9310-d96bd287cdaf/ljfp_a_217122_o_f0004g.gif)
Dynamic Properties
In order to determine the linear viscoelastic region, the rheological parameters [storage modulus (G′), loss modulus (G″) and complex modulus (G∗)] were first measured by conducting a stress sweep test at a fixed frequency. The stress of 1 Pa was then obtained from linear region and kept constant during course of measurements. The frequency development of the viscoelasticity of emulsions containing an even amount of surface active agents 1:1 or 1.5:1 gum/oil, on day 1 and day 14, are shown in and , respectively. The storage modulus (G′) and loss modulus (G″) for all emulsion systems showed very similar monotonic development at low frequency while emulsions prepared with modified starch and xanthan gum increased to a greater extent at elevated frequency (, and ). For emulsions prepared with gum arabic or starch and tragacanth, there appeared to be a more divergent behavior among the loss moduli with increasing frequency. It is interesting to note that, with the exception of emulsion formed by 14% modified starch and 0.3% xanthan, the loss modulus increased after 14 days of storage, while the storage modulus decreased slightly. This means that viscous component become more prominent after storage and is in agreement with the earlier results for zero-shear viscosity in steady-state flow tests. Comparing both figures, it can also be noted that the emulsions stabilized by tragacanth gum illustrated a lower storage moduli as compared to emulsions stabilized by xanthan gum. The diversity between storage modulus (G′) and loss modulus (G″) for emulsions containing tragacanth gum are also more pronounced ( and ).
Figure 5 Linear viscoelastic properties of emulsions at identical oil phase concentration and a) 9% Arabic + 0.8% Tragacant; b) 9% Arabic + 0.3% Xanthan; c) 9% Starch + 0.8% Tragacanth; and d) 9% Starch + 0.3% Xanthan.
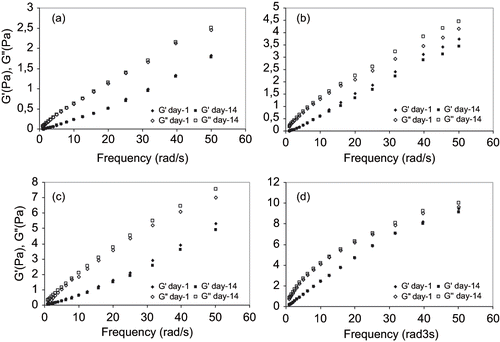
Figure 6 Linear viscoelastic properties of emulsions at identical oil phase concentration and e) 14% Arabic + 0.8% Tragacant; f) 14% Arabic + 0.3% Xanthan; g) 14% Starch + 0.8% Tragacanth; and h) 14% Starch + 0.3% Xanthan in water phases.
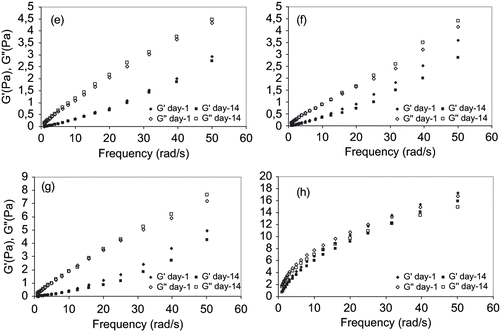
In our primary tests, xanthan gum did not reduce the surface tension of water while tragacanth gum resulted in decreasing the surface tension in a great extent (from 72.5 dyne/cm to 53 dyne/cm). Possibly, the elasticity of the covered oil droplets suspended in water phase dominated the measured viscoelasticity and, therefore, masked the viscoelastic effects of added tragacanth. For emulsions formed by modified starch and xanthan, the mechanical behavior was that of a viscoelastic liquid, where G′ ≥ G″ through the entire frequency range ( and ). Nonetheless, in the case of arabic and tragacanth gums, a weak-gel structure was observed where G″G′ and both moduli increase with frequency under almost the same gradient. All these features are unambiguous evidence that gel-network is being set up in modified starch coconut oil xanthan stabilized emulsions. Consecutively, the corresponding frequency developments of emulsions stabilized with arabic and tragacanth gums showed lower value of the viscoelastic moduli as compared to those of modified starch and xanthan gum stabilized emulsions. It should also be noted that the change in both moduli as influenced by storage time is lower for arabic and tragacanth gum stabilized emulsions ( and ) as compared to arabic and xanthan stabilized emulsions ( and ).
As pointed out earlier, in concentrated beverage emulsions, the colloidal interactions have been reported to be of short-range significance.[Citation9,Citation23] In this situation, the particles begin to interact with each other through a combination of hydrodynamic and colloidal interactions. Hydrodynamic interactions result from the relative motion of neighboring particles and alter the viscosity of the system. In order to account for the effect of these interactions, the additional volume fraction should be added,[Citation5] therefore the Einstein equation can be extended as
where a and b were found to be 4.5 and 6.2, respectively, for 14% starch-0.3% xanthan and 10 and 6.2, respectively for 14% arabic-0.8% tragacanth stabilized emulsions. compare experimental data of 14% starch-0.3% xanthan gum and 14% arabic-0.8%tragacanth gum stabilized emulsions, respectively, with the complex shear modulus, EquationEq. (9)(9), developed by Pal[Citation29] and predicted model (EquationEq. 13
(13)) suggested in this study. EquationEquation (9)
(9) is referred as Model (II) in both figures. These two emulsions indicated good stability after dilution in simulated citrus beverage with no sign of ringing after 5 weeks. The experimental data consisting of complex modulus of emulsions as a function of oscillation frequency are plotted and compared with both Pal (Model II) and predicted models. As illustrated, the Pal model (Model II) under-predicted the complex modulus and the deviation increased with increasing frequency. It should be noted that this model represented a good fit only at low value of dispersed phase volume fraction for suspension of spherical glass beads in purified carboxymethylcellulose (CMC) Pal.[Citation29] In our case, although the volume ratios of all prepared emulsions were lower than 0.1 (0.099 for 14% starch-0.3% xanthan stabilized emulsion and 0.091 for 14% arabic-0.8% tragacanth stabilized emulsion), the covered droplets did not act as glass beads. and also indicate that increase in elasticity of covered droplets could reduce this divergence.
Figure 7 Comparison of complex shear modulus of Starch-Xanthan and Arabic-Tragacanth stabilized emulsions with prediction of models [Model II, EquationEq. (9)(9) and Predicted, EquationEq. (13)
(13)].
![Figure 7 Comparison of complex shear modulus of Starch-Xanthan and Arabic-Tragacanth stabilized emulsions with prediction of models [Model II, EquationEq. (9)(9) and Predicted, EquationEq. (13)(13)].](/cms/asset/bfccaea1-db53-490d-a498-e25b20b2b0f6/ljfp_a_217122_o_f0007g.gif)
The differences in viscoelastic properties of the emulsions referred to in this study were related to the arabic-tragacanthe, starch-tragacanth, arabic-xanthan and starch-xanthan combinations. Hydrophobic interactions between the oil and proteins in tragacanth and arabic gums as well as the modified starch at the interface may account for the relaxation and overall viscoelastic properties. However, they cannot be considered as being the only responsible mechanisms controlling the degree of the aggregation of the emulsion droplets as reported by.[Citation33] Dramatic reduction of emulsification capability of gum arabic has been reported at low pH.[Citation34] Even though xanthan gum did not impart in reduction of surface tension but it was the most effective gum in controlling the rheological properties.
Stability of Emulsions in Concentrated and Diluted Form
The stability of emulsions in diluted form against oil droplet coalescence was determined by following the change with time of the average droplets size and droplet aggregation rate. The rate of coalescence of emulsion droplets (Dc ) mainly followed the first-order kinetics.[Citation13,Citation25,Citation35]
where N0 and Nt are the numbers of droplets per unit volume of emulsion initially and time t, respectively, and Dc is the rate droplets coalescence. In term of average droplets size, the equation is given as:[Citation25]
where D0 and Dt are the mean droplet sizes initially and at time t, respectively. Therefore Dc was determined by plotting 3(ln (Dt/D0 ) versus time (t). The results for emulsion droplet size, rate of coalescence of emulsion droplets, opacity and ringing in bottle for all prepared emulsions are tabulated in . The extent of droplet coalescence is associated with creaming (ringing in bottle). Emulsion stabilized by arabic-xanthan combinations indicated the highest rate of coalescence in both concentrated and diluted forms. Modified starch-tragacanth stabilized emulsions also demonstrated a lower stability compared to arabic-tragacanth and modified starch-xanthan stabilized emulsions. Increase in concentration of gum arabic in arabic-xanthan emulsions did not improve the emulsion stability, and additional concentration of modified starch in starch-tragacanth emulsions had a slight effect on stability improvement, possibly, due to increase in water phase viscosity. Arabic-tragacanth emulsions, despite of low viscosity of their water phases, were also among the most stable emulsions. The highest rate of coalescence was associated with arabic-xanthan emulsions and the lowest rate of coalescence was associated with the modified starch-xanthan emulsions.
Table 2 Comparison of particle size growth, volume ratio, opacity, and stability in simulated beverages for prepared emulsions
In order to find an explanation for all these occurrences, the previously reported data by Garti and Reichman[Citation34] would be useful. They reported that “the gum arabic behaves as a typical surface active protein and anchors strongly to the oil phase via its proteinaceous part of the molecule. When the proteinaceous part is removed, denatured, or deactivated, the gum tends to lose its surface activity and emulsification capacity.” It is also worthy to mention that the rheological testes on arabic gum solution indicated lower viscosity and practically Newtonian behavior at the selected concentrations. The lower stability of arabic-xanthan emulsions may, therefore, be due to the loss of emulsification capacity of arabic gum as the influence of acidity and pasteurization temperature. Since xanthan gum as well did not indicate any surface activity, the only stabilization effect is due to the viscosity and, hence, emulsion will separate rapidly. The results are also in agreement with Chanamai and McClements,[Citation3] who studied the depletion flocculation of beverage emulsions by gum arabic and modified starch. They reported that emulsions containing gum arabic are more susceptible to depletion flocculation compare to emulsions containing modified starch. They also reported that gum arabic has a greater aqueous phase effective volume than modified starch due to higher molecular weight and more open structure. In a study by Parker et al.[Citation36] on stabilization of salad dressing by xanthan gum, the reduction in elastic characteristics has been related to the restructuring of the network and reduction in the number of bonds between connected flocs.
In the case of arabic-tragacanth emulsions, although the emulsions had an identical type of preparation, tragacanth gum is more surface active and is more resistant to acid and heat treatment. Therefore, the greater stability of these emulsions may be due to the residual surface activity contributed by tragacanth gum. Gum arabic stabilized emulsions also added greater opacity to the simulated beverage emulsions compared to modified starch stabilized emulsions. The rate of creaming (aggregation) for emulsion at low and elevated concentrations of arabic and modified starch stabilized emulsions are illustrated in and , respectively. As the rates of aggregations are the highest for arabic-xanthan emulsions, the results are in excellent agreement with previously mentioned coalescence rate. Once again, the modified starch-xanthan emulsion at elevated concentration of modified starch indicated the lowest aggregation rate and, hence, the most stable emulsion in both concentrated and diluted forms.
Figure 8 Creaming velocity profiles for simulated beverages containing 2% of emulsions at 9% Starch (St), 9% Arabic gum (Ar), 0.8% Tragacanth gum (Tr), and 0.3% Xanthan gum (X) concentrations.
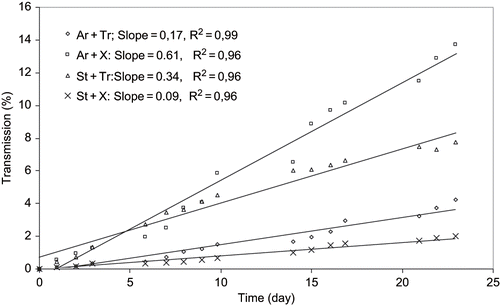
Figure 9 Creaming velocity profiles for simulated beverages containing 2% of emulsions at 14% Starch (St), 14% Arabic gum (Ar), 0.8% Tragacanth gum (Tr), and 0.3% Xanthan gum (X) concentrations.
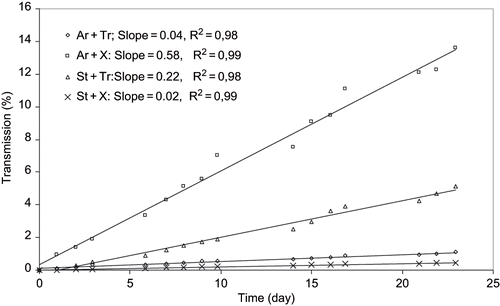
and illustrate shelf stability of pasteurized simulated citrus beverages containing 2% emulsions after 12 weeks storage at room temperature for 1:1 and 1.5:1 surface active hydrocolloids to oil combinations, respectively. As it can be observed that the ringing and aggregation are associated mostly with arabic-xanthan emulsions and slightly with modified starch-tragacanth emulsions at low and elevated surface active gum concentrations. Since the separation of starch-tragacanth stabilized emulsion was also observed in the concentrated form, the instability may be is due to the incompatibility or coacervation of these two hydrocolloids. As Dickinson[Citation10] stated, the thermodynamic incompatibility implies the separation of emulsion into two distinct aqueous phases, one rich in protein and other rich in hydrocolloid. The observations, therefore, are in a good agreement with the results obtained by either rheological assessment or particle properties examinations.
CONCLUSIONS
This study demonstrated that both modified starch-xanthan and arabic-tragacanth gum combinations could be used to form highly stabilized beverage cloud emulsions. In the considered concentration range, the rheological properties of modified starch-xanthan gum combination were able to provide a gel matrix and highly stable emulsion. Arabic-tragacanth stabilized emulsions demonstrated rheological properties with fewer changes in shear thinning behavior after storage. The rheology of all prepared emulsions can be confidently modeled through the Einstein equation or its exponential expansion. The predicted model for complex shear modulus also well fitted with experimental data. With the exception of arabic-xanthan emulsions which demonstrated the lowest stability, the creaming stability and rheology of beverage emulsions were affected by increasing surface active gum concentration. The lower stability of arabic-xanthan was suggested to be due to either loss of emulsification capacity as an influence of pasteurization or more open structure of arabic gum and loss of elastic characteristics of xanthan gum. In the case of arabic-tragacanth emulsions, the greater stability could be observed due to higher surface activity and higher stability to acid and heat by tragacanth gum. From a practical point of view, the results provide useful information for achieving reasonable stability and opacity for concentrated emulsion and fruit beverages.
REFERENCES
- Turbiano , P.C. 1995 . “ The Role of Specialty Food Starches in Flavor Emulsions ” . In Flavor Technology: Physical Chemistry, Modification and Process , Edited by: Ho , C.T. , Tan , C.T. and Tong , C.H. 199 – 209 . Washington, D.C. : American Chemical Society .
- Chee-Teck , T. and Wu Holmes , J. 1988 . Stability of Beverage Flavor Emulsions . Perfumer & Flavorist , 13 : 23 – 41 .
- Chanamai , R. and McClements , D.J. 2001 . Depletion Flocculation of Beverage Emulsions by Gum Arabic and Modified Starch . J. of Food Science , 66 : 457 – 463 .
- Chee-Teck , T. 2004 . “ Beverage Emulsions ” . In Food Emulsions , 4th , Edited by: Friberg , S.E. , Larsson , K. and Sjöblom , J. 485 – 524 . New York : Marcel Dekker .
- McClements , D.J. 2005 . Food Emulsions, Principles, Practice, and Techniques , 2nd , Florida : CRC Press, LLC .
- Taherian , A.R. , Fustier , P. and Ramaswamy , H.S. 2006 . Effect of Added Oil and Modified Starch on Rheological Properties, Droplet Size Distribution, Opacity and Stability of Beverage Cloud Emulsions . J. of Food Eng. , 77 ( 3 ) : 687 – 696 .
- Glicksman , M. 1969 . Gum Technology in the Food Industry , New York : Academic Press .
- Sharma , S.C. 1981 . Gum and Hydrocolloids in Oil-water Emulsions . Food Technology , January : 59 – 67 .
- Buffo , R.A. , Reineccius , G.A. and Oehlert , G.W. 2001 . Factors Affecting the Emulsifying and Rheological Properties of Gum Acacia in Beverage Emulsions . Food Hydrocolloids , 15 : 53 – 66 .
- Dickinson , E. 2003 . Hydrocolloids at Interfaces and the Influence on the Properties of Dispersed System . Review, Food Hydrocolloids , 17 : 25 – 39 .
- Tesch , S. , Gerhards , Ch and Schubert , H. 2002 . Stabilization of Emulsions by OSA Starches . J. of Food Eng. , 54 : 167 – 174 .
- Sahin , H. and Ozdemir , F. 2004 . Effect of Some Hydrocolloids on the Rheological Properties of Different Formulated Ketchups . Food Hydrocolloids , 18 : 1015 – 1022 .
- Paraskevopoulou , A. , Boskou , D. and Kiosseoglou , V. 2005 . Stabilization of Olive Oil—Lemon Juice Emulsion with Polysaccharides . Food Chemistry , 90 : 627 – 634 .
- Ahmed , J. , Ramaswamy , H.S. and Ngadi , M.O. 2005 . Rheological Characteristics of Arabic Gum in Combination with Guar and Xanthan Gum Using Response Surface Methodology: Effect of temperature and concentration . International Journal of Food Properties , 8 ( 2 ) : 179 – 192 .
- Mandala , I. and Palogou , E. 2003 . Effect of Preparation Conditions and Starch/Xanthan Concentration on Gelation Process of Potato Starch Systems International Journal of Food Properties , 6 ( 2 ) : 311 – 318 .
- Coia , K.A. and Stauffer , K.R. 1987 . Shelf life Study of Oil/Water Emulsions Using Various Commercial Hydrocolloids . J. of Food Science , 52 ( 1 ) : 166 – 172 .
- Quemada , D. and Berli , C. 2002 . Energy of Interaction in Colloids and Its Implications in Rheological Modeling . Advances in Colloid and Interface Science , 98 : 51 – 85 .
- Bais , D. , Trevisan , A. , Lapasin , R. , Partal , P. and Gallegos , C. 2005 . Rheological Characterization of Polysaccharide-Surfactant Matrices for Cosmetic O/W Emulsions . J. of Colloid and Interface Science , 290 : 546 – 556 .
- Pal , R. 2000 . Linear Viscoelastic Behaviour of Multiphase Dispersions . J. of Colloid and Interface Science , 232 : 50 – 63 .
- Den , Van and Tempel , M. 1963 . “ Effect of Droplet Flocculation on Emulsion Viscosity ” . In Rheology of Emulsions Edited by: Sherman , Ed . 1 – 14 .
- Xie , Y.R. and Hettiarachchy , N.S. 1997 . Xanthan Gum Effects on Solubility and Emulsification Properties of Soy Protein Isolate . J. of Food Science , 6 ( 62 ) : 1101 – 1104 .
- Chen , J. and Dickinson , E. 1999 . Effect of Monoglycerides and Diglycerol-esters on Viscoelasticity of Heat-set Whey Protein Emulsion Gels . International J. of Food Science and Tech. , 34 : 493 – 501 .
- Buffo , R.A. and Reineccius , G.A. 2002 . Modeling the Rheology of Concentrated Beverage Emulsions . J. Food Eng. , 51 : 267 – 272 .
- Batista , A.P. , Raymundo , A. , Sousa , I. and Empis , J. 2006 . Rheological Characterization of Coloured Oil-in-Water Food Emulsions with Lutein and Phycocyanin Added to the Oil and Aqueous Phases . Food Hydrocolloids , 20 : 44 – 52 .
- Sherman , P. 1983 . “ Rheological Properties of Emulsions ” . In Encyclopedia of Emulsion Technology , Edited by: Becher , P. Vol. 1 , 405 – 437 . New York : Marcel Dekker Inc . 1
- Pal , R. and Rhodes , E. 1989 . Viscosity/Concentration Relationships for Emulsions . J. of Rheology , 33 ( 7 ) : 1021 – 1045 .
- Starov , V.M. and Zhdanov , V.G. 2003 . Viscosity of Emulsions: Influence of Flocculation . J. of Colloid and Interface Science , 258 : 404 – 414 .
- Klinkesorn , U. , Sophanodora , P. , Chinachoti , P. and McClements , D.J. 2004 . Stability and Rheology of Corn Oil-in-Water Emulsions Containing Maltodextrin . Food Res. Int. , 37 : 851 – 859 .
- Pal , R. 2002 . Complex Shear Modulus of Concentrated Suspensions of Solid Spherical Particles . J. of Colloid and Interface Science , 245 : 171 – 177 .
- Huang , X. , Kakuda , Y. and Cui , W. 2001 . Hydrocolloids in Emulsions: Particle Size Distribution and Interfacial Activity . Food Hydrocolloids , 15 : 533 – 542 .
- Lopez , A. 1987 . “ Acomplete Course in Canning and Related Processes ” . In Book II—Packaging. Aseptic Processing Ingredients , Baltimore, MD : The Canning Trade Inc .
- Carreau , P.J. 1972 . Rheological Equations from Molecular Network Theories . Transactions of the Society of Rheology , 16 : 99 – 127 .
- Valdez , M.A. , Acedo-Carrillo , J.I. , Rosas-Durazo , A. , Lizaradi , J. , Rinaudo , M. and Goycoolea , F.M. 2006 . Small-Deformation of Mesquite Gum Stabilized Oil in Water Emulsions . Carbohydrate Polymer , 64 : 205 – 211 .
- Garti , N. and Reichman , D. 1993 . Hydrocolloids as Food Emulsifier and Stabilizer . Food Structures , 12 : 411 – 426 .
- Ye , A. , Hemar , Y. and Singh , H. 2004 . Enhancement of Coalescence by Xanthan Addition to Oil-in-Water Emulsions Formed with Extensively Hydrolyzed Whey Proteins . Food Hydrocolloids , 18 : 737 – 764 .
- Parker , A. , Gunning , P.A. , Ng , K. and Robins , M.M. 1995 . How Does Xanthan Stabilise Salad Dressing? . Food Hydrocolloids , 9 ( 4 ) : 333 – 342 .