Abstract
Four treatments of Cheddar cheese with two levels (high and low) of calcium (Ca) and phosphorus (P), and two levels (high and low) of residual lactose were manufactured. Each treatment was subsequently split prior to the salting step of cheese manufacturing process and salted at two levels (high and low) for a total of eight treatments. After two months of ripening, each treatment of Cheddar cheese was used to manufacture process cheese using a twin-screw Blentech process cheese cooker. NFDM, butter oil, trisodium citrate (emulsifying salt), and water were added along with Cheddar cheese for process cheese formulation. All process cheese food formulations were balanced for moisture (43.5%), fat (25%), and salt (2%), respectively. Dynamic rheological characteristics (G′ and G″) of process cheese were determined at 1.5Hz frequency and 750 Pa stress level by using a Viscoanalyzer during heating and cooling, temperature ranges from 30°C to 70°C then back to 30°C. High Ca and P content, and high S/M (HHH and HLH) cheeses had the significantly higher elastic (G′) and viscous (G″) modulus than other cheeses during heating from 30°C to 70°C, and cooling from 70°C to 30°C. No significant difference was observed among the other process cheeses during heating and cooling. Viscoelastic properties of process cheeses were also determined in terms of transition temperature (where G′ = G″), and tan δ during heating (30°C to 70°C). Cheeses with high Ca and P, high lactose, and high S/M content had higher transition temperature than low Ca and P, low lactose, and low S/M content process cheeses. Low Ca and P and low S/M content cheeses (LLL, LHH, LHL, HLL) exhibited more viscous characteristics than high Ca and P and high S/M content process cheeses (HHL, HLH, LLH, HHH) during heating from 30°C to 70°C. Low Ca and P, low lactose, low S/M content (LLL) process cheese was observed for highest tan δ values (0.39 to 1.43), whereas high Ca and P, high lactose, high S/M content process (HHH) had the least (0.33 to 1.06) during heating. This study demonstrates that different characteristics of natural cheese used in process cheese manufacturing have significant impact on process cheese rheological and viscoelastic properties.
INTRODUCTION
Production of process cheese from natural cheeses has increased drastically in the United States over the last few years. Currently more than 2.24 billion pounds of process cheese is produced annually.[Citation1] Natural cheese is the major ingredient in process cheese manufacturing. About 25% of the natural cheese produced in the United States is used in the manufacture of process cheese.[Citation1] Process cheese is frequently consumed as an ingredient with other food items, and available in several forms, such as slices, blocks, shreds, and sauces.
Dynamic rheology testing is a fundamental method for determining the rheological properties of viscoelastic materials.[Citation2] This test is rapid and involves minimal chemical and physical manipulation.[Citation3] It has been recommended that small amplitude oscillatory shear (SAOS) measurement technique should be used to study the linear viscoelasticity of cheese and other food materials. These tests include stress or strain sweep, frequency sweep, temperature sweep, and time sweep.[Citation4]
G′ (elastic or solid like property) and G″ (viscous or liquid like property) values can be determined at various frequencies and temperatures within a short period of time, which also comforts linear stress-strain behavior, and can be applied in mathematical modeling. Dynamic rheological test methods allow the researchers to better understand the viscoelastic nature of a cheese.[Citation5] These measurements have an advantage especially for quality assurance in the industry, which gives an indication between the protein-protein interactions, and fat globules in cheese.[Citation5]
Storage or elastic modulus (G′), loss or viscous modulus (G″), the phase shift or angle (mechanical loss angle) δ, and the loss tangent (i.e. tan δ) are the main dynamic viscoelastic properties of cheese. G′ and G″ have been reported for variety of cheeses at different temperatures, where G′ measures the energy stored and subsequently released per cycle of deformation, and G″ measures the energy dissipated per cycle of deformation.[Citation6] The ratio of G′ and G″ is defined as:
Loss tangent or tan δ could be potentially used as a predictor for cheese meltability.[Citation7] With the increase in tan δ the materials is reacting to an external stress in a relatively more viscous and less elastic manner.[Citation6,Citation8] The modulus value at tan δ = 1 (45° phase angle) is known as crossover modulus, where the material is equally solid and liquid like characteristics. It had been studied that if tan δ is less than 1 the material is more elastic, and when tan δ is more than 1 the material is more viscous.[Citation6]
According to Joshi et al.,[Citation9] elastic modulus of Mozzarella cheese is always greater than viscous modulus in normal conditions. During heating of cheese softening occurs and it loses it elasticity. Viscous modulus may become greater than elastic modulus when flow occur.[Citation10] A simultaneous decrease in its elastic and viscous moduli also takes place that facilitates flow of the cheese mass.[Citation11,Citation12,Citation13] It has also been concluded that there is critical level of energy stored and dissipated within cheese mass that allows casein network to melt and flow during heating.[Citation10]
Effect of Ca and P content, lactose level, and salt-to-moisture ratio in cheese also play an important role in deciding its rheological properties.[Citation14,Citation15,Citation16,Citation17] Very little research has been carried out and reported in the literatures that correlated to micronutrients levels and rheological properties of process cheese. However, Guinee et al.,[Citation18] reported that Mozzarella cheese containing reduced calcium had low value of elastic modulus and high value of phase angle, indicating a high level of fluidity in that particular cheese. The authors also reported a steep decline in G′ and G″ upon increasing the temperature of cheese from 20°C to 45°C. In other studies on Mozzarella cheese researchers revealed that reduction in calcium percentage in cheese helps to improve the texture by lowering the hardness and increasing the functional and rheological characteristics.[Citation19,Citation20]
The main aim of this study was to investigate the effects of Ca and P content (high/low), residual lactose (high/low), and salt-to-moisture (S/M) ratio (high/low) on dynamic rheological parameters (G′ and G″ spectra) with the changing in temperature (heating and cooling). Test temperature gradient was used from 30°C to 70°C (heating) and back to 30°C (cooling). Transition temperatures along with tan δ (phase angle) were also determined during heating (30°C to 70°C) to understand the complete viscoelastic nature of process cheeses. These findings will help the cheese manufacturers to get necessary information regarding process cheese production on large scale with desired and improved rheological and melt/flow characteristics by controlling calcium and phosphorus, residual lactose, and salt/moisture ratio content in natural cheese.
MATERIALS AND METHODS
Process Cheese Manufacturing
Four treatments of Cheddar cheese with two levels (high—addition of 144 ml CaCl2/1600lbs of milk, and low—no addition) of calcium (Ca) and phosphorus (P), and two levels (high—addition of 2.5% lactose, and low—no addition) of residual lactose were manufactured as described by Upreti.[Citation21] Each treatment was subsequently split prior to the salting step of cheese manufacturing and salted at two levels (high—addition of 3.5% salt, and low—2.25% salt) for a total of eight treatments. Complete experimental design for Cheddar cheese preparation is shown in . The eight treatments included: High Ca and P, High lactose, High S/M (HHH); High Ca and P, High lactose, Low S/M (HHL); High Ca and P, Low lactose, High S/M (HLH); High Ca and P, Low lactose, Low S/M (HLL); Low Ca and P, High lactose, High S/M (LHH); Low Ca and P, High lactose, Low S/M (LHL); Low Ca and P, Low lactose, High S/M (LLH); and Low Ca and P, Low lactose, Low S/M (LLL). At the end of two months of ripening each treatment of Cheddar cheese was used to manufacture process cheese food using a Blentech™ twin-screw (BTS) process cheese cooker blender (Blentech Corporation, Rohnert Park, CA). All process cheese food formulations were balanced for moisture, fat, and salt with the values for moisture (43.5%), fat (25%), and salt (2%), respectively. The prepared eight different process cheeses have been indicated by the same nomenclature that was used for eight different natural Cheddar cheese treatments from where the process cheeses were manufactured (total in 3 replications) as mentioned above.
Among other ingredients non-fat dried milk (∼7%) (low heat) (Dairy America™, Fresno, CA), anhydrous butter oil (∼0.15%) (Mid-America Farms, Springfield, MO), water (∼18%), and trisodium citrate (2.5%) (Archer Daniels Midland Company, Decatur, IL) as emulsifier salt were used. Overall formulation for eight different process cheeses is shown in . All of these ingredients along with natural Cheddar cheese were mixed at 75 rpm for 30 min at room temperature. The temperature of the pre-blend was increased to 80°C in 5 min and held for an additional 5 min. The auger speed was 140 rpm during the heating and the holding period of the manufacturing process. The cooked process cheeses were filled hot in 1 kg boxes and transferred to a cold room (4°C) after 15 min. All the cooked process cheeses were stored at 4°C until further analysis was completed.
Table 1 Average formulations with different ingredients for eight different process cheeses
Chemical Analysis
The moisture content of process cheese was analyzed by using a vacuum oven as described by Bradley and Vanderwarn.[Citation22] Fat content was determined using the Mojonnier method.[Citation23] Salt content was measured using a Corning Chloride Analyzer 926. pH was measured with a Corning pH/ion meter (Model 450, Corning Glass Works, Medfield, MA) method as described by Kapoor and Metzger.[Citation24] Total calcium and total phosphorous of process cheese was determined by the method described by Brooks et al.,[Citation25] and AOAC 991.25,[Citation26] respectively. Lactose content was determined by using HPLC as described by Zeppa et al.[Citation27]
Measurement for Dynamic Rheology—Stress Sweep
Stress sweep experiment for process cheese was carried out by using a rheometer (ATS Rheosystems, Rheologica Instruments Inc., NJ) with parallel plate geometry of 30 mm diameter. Fine sandpaper (400 grits, wet or dry, 3M) was glued to the upper plate of the rheometer to prevent sample slippage. During sample loading, the sample was placed on the lower plate and upper plate was brought in contact over the sample. Exposed sample edge was then coated with vegetable oil (Midwest Country Fare, Des Moines, IA) to minimize drying during measurements. Stress sweep was performed at a frequency of 1.5 Hz, and with a stress ranging from 1 to 3000 Pa at 20°C. Before starting the actual test, preshear of samples were done at 11 s−1 for 20 sec, followed by 25 sec equilibrium time. Maximum stress limit for linear viscoelastic region was obtained as 750 Pa from stress sweep experiment.
Dynamic Rheology Spectra
Dynamic rheological testing was performed using the same rheometer (ATS Rheosystems, Rheologica Instruments Inc., NJ) at 1.5 Hz frequency and constant stress of 750 Pa (linear viscoelastic region). The instrument was fully automated, and necessary data were collected by a personal computer in MS-EXCEL format attached to the instrument. Parallel plate geometry of 30 mm diameter was used for loading the specimens during the experiment.
Process cheese samples were prepared by cutting a thin slice (∼2.0 mm) using a food slicer (Model 1042W, The Rival Co. Kansas City, MO). Cylindrical cheese samples of diameter 28.3 mm were cut with help of a cork borer and a cylindrical mallet from the same slice. The specimens were placed in covered petri plate to minimize moisture loss and kept inside the refrigerator at 4°C–6°C until testing. Before running the actual test the specimen along with petri plate was placed at room temperature for 15 min to bring the cheese temperature to around 25°C.
The samples were then placed on the lower plate and upper plate was brought in contact with the sample. The exposed cheese surface was coated with vegetable oil (Midwest, Country Fare soybean oil) to minimize drying during measurement. Time sweep mode was used along with a temperature ramp of 30°C to 70°C and back to 30°C at a rate of 1°C / min to measure the rheological properties. Before starting of actual test preshear of samples were done at 11 s−1 for 20 sec to homogenously equilibrate the sample. All measurements were done in triplicate, which includes the elastic modulus (G′), viscous modulus (G″), transition temperature, and tangent angle (tan δ). Transition temperature was defined as the lowest temperature during the temperature gradient experiment, where tan δ equaled 1 (G′ = G″).
Statistical Analysis
Observations for all the parameters were done in triplicate, and the data were analyzed using a split-split-plot design with Ca and P content (high/low), lactose (high/low) and salt-to-moisture (S/M) ratio (high/low) as the whole plot factors. PROC GLM procedure of SAS software[Citation28] was used to find LSD means. Duncan's multiple range was also used to separate among the means of three replicates. All statistical data were analyzed and reported with the minimum significant level of P < 0.05.
RESULTS AND DISCUSSION
Chemical Composition
The chemical composition of eight different process cheeses is presented in . It was observed that pH for process cheese sample ranges from 5.65 to 6.16. HLH and HHH process cheeses were observed for maximum pH value of 6.16 and 6.10, respectively, and LHL was observed for least pH value of 5.65. No significant difference (P > 0.05) was found for moisture and fat content among the cheeses. The moisture and fat content range between 42.9% to 43.57%, and 25.18% to 25.65%, respectively. Total Ca and P contents for HHH (0.56% and 0.39%), HHL (0.53% and 0.38%), HLH(0.56% and 0.39%) and HLL (0.56% and 0.39%) were significantly higher (P < 0.05) than those of LHH (0.48% and 0.35%), LHL (0.48% and 0.35%), LLH (0.46% and 0.34%), and LLL (0.46% and 0.33%) process cheeses. Significant difference (P < 0.05) was also observed for lactose content among eight different process cheeses.
Table 2 Composition of process cheese
Dynamic Rheology of Process Cheese—Stress Sweep
The region of linear viscoelasticity (constant G′ and G″ in stress sweep) for process cheese samples is illustrated in and . It was observed that highest shear stress for linear region was about 750 Pa for all process cheese samples. Process cheese with high Ca and P content and S/M ratio (HLH and HHH) showed more G′ and G″ values than that of low Ca and P content and S/M ratio cheeses (HLL, HHL, LLL, LLH, LHL, and LHH), ( and ).
Effect of Experimental Variables (Ca and P content, Residual Lactose, S/M and Temperature) on Storage and Loss Modulus
Process cheese containing high Ca and P and high salt-to-moisture (S/M) ratio (HHH and HLH) had been observed for significantly higher (P > 0.05) elastic modulus (G′) at 30°C compare to other cheeses (, ). The values for HHH and HLH were 28.9 kPa and 29.6 kPa respectively at 30°C, and 2.6 kPa and 3.9 kPa, respectively, at 70°C. It was also observed that with the increase in temperature from 30°C to 70°C, values of elastic modulus decreased and vice versa ( and ) for all process cheeses. A similar trend was observed for viscous modulus (G″) during the heating and cooling process respectively (; and ). The G″ values for HLH and HHH were 10.5 kPa and 10.4 kPa respectively at 30°C, and 3.1 kPa and 2.3 kPa respectively at 70°C, these values were significantly higher (P < 0.05) than those of other cheeses ().
Table 3 Effects of Ca and P content, lactose and S/M on elastic (G′, kPa) modulus on process cheese during heating and cooling
Figure 4 Effects of Ca and P content, lactose and S/M level (high/low) on elastic (G′) modulus of process cheese during heating.
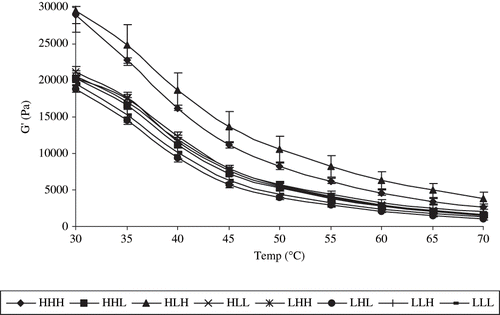
Figure 5 Effects of Ca and P content, lactose and S/M level (high/low) on elastic (G′) modulus of process cheese during cooling.
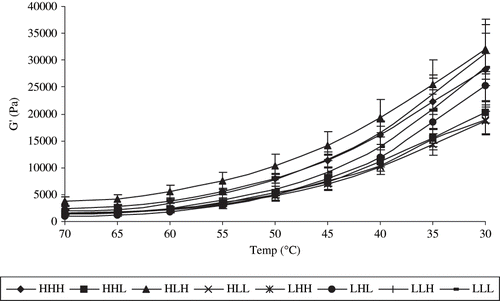
Table 4 Effects of Ca and P content, lactose and S/M on viscous (G″, kPa) modulus on process cheese during heating and cooling
Figure 6 Effects of Ca and P content, lactose and S/M level (high/low) on viscous (G″) modulus of process cheese during heating.
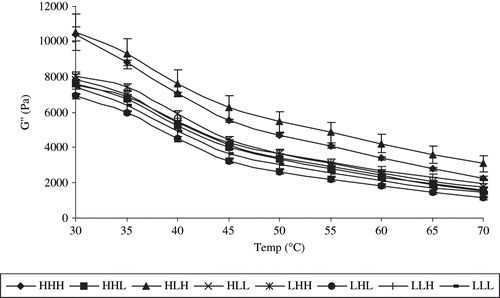
Figure 7 Effects of Ca and P content, lactose and S/M level (high/low) on viscous (G″) modulus of process cheese during cooling.
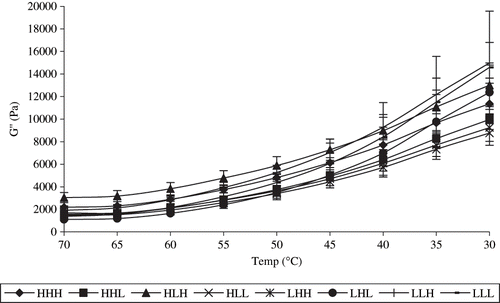
Venugopal and Muthukumarappan[Citation29] observed decrease in elastic (G′) and viscous (G″) moduli for Mozzarella and Cheddar cheese upon heating from 25°C to 60°C, and increase in G′ and G″ during cooling (60°C to 25°C). Guinee et al.,[Citation18] also observed similar trend for determining G′ and G″ values in cheese during heating. In the present study, low Ca and P and high/low lactose content process cheeses did not show any significant difference (P > 0.05) in G′ and G″ values among themselves during heating and cooling process ( and ), respectively.
Viscoelasticity of Process Cheese
The viscoelastic properties of eight different process cheeses were estimated in terms of their transition temperature and tan δ value. Transition temperature is the lowest temperature at which the elastic and viscous moduli are equal (i.e. G′ = G″ at tan δ = 1). At transition temperature process cheese changes in its rheological property, i.e. from elastic like mass to more viscous like mass.[Citation30] The effects of Ca and P content, residual lactose, and S/M ratio on viscoelastic properties of process cheese were described hereafter.
Effect of Ca and P content, Residual Lactose and Salt-to-Moisture (S/M) Ratio on Transition Temperature
Process cheese containing high Ca and P content, (HHH, HHL, and HLH) had significantly (P < 0.05) higher transition temperature than cheese with low Ca and P content, (LHH, LHL, and LLH) (). The temperature ranges from 65.94°C to 68.14°C for high Ca and P content cheeses, and from 64.52°C to 65.41°C for low Ca and P content cheeses. However, no significant difference (P > 0.05) was observed between the high (HLL) and low (LLL) Ca and P content process cheese. Similar findings in transition temperature of around 60°C for process cheese containing different emulsifying salts were also reported.[Citation30,Citation31,Citation32]
High lactose content process cheeses (HHH, HHL, LHL) had significantly higher (P < 0.05) transition temperature compared to low lactose content process cheeses (HLH, HLL, LLL) (). No significant difference was observed between LHH and LLH cheeses.
It was observed that high S/M ratio process cheeses (HHH, HLH, LLH) had significantly higher (P < 0.05) transition temperature than that of low S/M content cheeses (HHL, HLL, LLL) (). This may be due to the fact that high S/M ratio affects the cheese functionality by increasing hardness, which in turn increases the temperature for cheese meltability.[Citation33,Citation34] However, low Ca and P content process cheeses (LHH and LHL) didn't show any significant difference between their transition temperatures ().
Influence of different Treatments on Tan δ of Process Cheese during Heating
Tan δ versus temperature () expressed a clearer indication that whether the process cheese was more elastic or more viscous, and also manifested a quantitative measurement of these properties at different temperature. If tan δ value is greater than 1.0, the process cheese will start melting and flow, since it becomes more viscous. indicates that low Ca and P content and low S/M ratio cheeses (LLL, LHH, LHL, HLL) had more viscous characteristics than high Ca and P content and high S/M ratio process cheeses (HHL, HLH, LLH, HHH) during heating from 30°C to 70°C. This phenomenon also explains that former group of process cheeses had better melting and flow behavior than later group of process cheeses. It was also indicated that LLL had best melt/flow characteristics, whereas HHH had least among all process cheeses. Tan δ values increased from 0.39 to 1.43, and 0.33 to 1.06 for LLL and HHH respectively with the increase in temperature from 30°C to 70°C. Similar kind of studies were performed by Sutheerawattananonda[Citation30] with process cheese manufactured with different emulsifying salts. Process cheese prepared with trisodium citrate exhibited more viscous characteristics than cheese prepared with disodium phosphate.
CONCLUSIONS
Process cheese containing high Ca and P and high S/M ratio (HHH and HLH) had significantly higher elastic (G′) and viscous (G″) moduli compare to other cheeses with low Ca and P and Low S/M ratio during heating and cooling process. However, no significant difference was observed among other process cheeses during heating and cooling respectively. Process cheeses with high Ca and P content (HHH, HHL, HLH), high residual lactose (HHH, HHL, LHL), and high S/M ratio (HHH, HLH, LLH) had higher transition temperature than low Ca and P content (LHH, LHL, LLH), low residual lactose (HLH, HLL, LLL), and low S/M ratio (HHL, HLL, LLL) process cheeses. Low Ca and P content and low S/M ratio cheeses (LLL, LHH, LHL, HLL) exhibited more viscous characteristics than high Ca and P content and high S/M ratio (HHL, HLH, LLH, HHH) process cheeses during heating from 30°C to 70°C. Among eight different process cheeses, LLL exhibited highest viscous and HHH exhibited lowest viscous behavior during heating, which was predicted from its tan δ value.
ACKNOWLEDGMENTS
This research undertaking was supported and funded by Dairy Management Inc. (DMI) and Agricultural Experimental Station (AES), South Dakota State University, Brookings, SD, USA. The authors deeply acknowledge for its successful completion.
REFERENCES
- International Dairy Foods Association . 2005 . Dairy Facts , Washington, DC : Milk Industry Foundation, IDFA .
- Ferry , J.D. 1980 . Viscoelastic Properties of Polymers , 3rd , New York : John Wiley & Sons .
- Rao , M.A. 1992 . “ Classification, Description and Measurement of Viscoelastic Properties of Solid Foods ” . In Viscoelastic Properties of Foods , Edited by: Rao , M.A. and Steffe , J.F. 3 – 49 . New York : Elsevier Applied Science .
- Ak , M.M. and Gunasekaran , S. 2001 . “ Linear Viscoelastic Methods ” . In Nondestructive Food Evaluation: Techniques to Anyalyze Properties and Quality , Edited by: Gunasekaran , S . 287 – 333 . New York : Marcel Dekker, Inc. .
- Rowney , M. , Roupas , P , Hickey , P.W. and Everett , D.W. 1999 . Factors Affecting Functionality of Mozzarella Cheese . Australian. J. Dairy Technol. , 54 : 94 – 102 .
- Gunasekaran , S. and Ak , M.M. 2003 . Cheese Rheology and Texture , Boca Raton, FL : CRC Press LLC .
- Ruegg , M. , Eberhard , P , Popplewell , L.M. and Peleg , M. 1991 . Melting Properties of Cheese , 36 – 43 . Brussels, , Belgium : Int. Dairy Fed . Bull. 268
- van Vleit , T. 1991 . Terminology to be Used in Cheese Rheology , Brussels, , Belgium : Int. Dairy Fed . Bull. 268.
- Joshi , N.S. , Dave , R.I. and Muthukumarappan , K. 2004 . Viscoelastic Properties of Part Skim Mozzarella Cheese: Effect of Calcium, Storage and Test Temperature . International J. Food Prop. , 7 ( 2 ) : 239 – 252 .
- Lucey , J.A. , Johnson , M.E. and Horne , D.S. 2003 . Perspectives on the Basis of Rheology and Texture Properties of Cheese . J. Dairy Sci. , 86 : 2725 – 2743 .
- Taneya , S. , Izutsu , T. and Sone , T. 1979 . “ Dynamic Viscoelasticity of Natural Cheese and Processed Cheese ” . In Food Texture and Rheology , Edited by: Sherman , P. 369 – 383 . London : Academic Press .
- Horne , D.S. , Banks , J.M. , Leaver , J. and Law , A.J.R. . Dynamic Mechanical Spectroscopy of Cheddar Cheese. Cheese Yield and Factors Affecting Its Control . Proceedings IDF Seminar . April 1993 , Cork, Ireland. pp. 507 – 512 . Brussels, , Belgium : International Dairy Federation .
- Guinee , T.P. , Auty , M.A.E. and Mullins , C. 1999 . Observations on the Microstructure and Heat Induced Viscoelasticity of Commercial Cheese . Australian J. Dairy Technol. , 54 : 84 – 89 .
- Strandholm , J.J. , Prochnow , R.R. , Miller , M.S. , Woodford , L.E. and Neunaber , S.M. Method for Controlling Melting Properties of Process Cheese. Kraft Inc . United States Patent, US 4885183 . Filing date 10/19/1998. Publication Date 12/05/1989.
- Aizawa , S. and Yoneda , Y. . Preparation of Melt-resistant Process Cheese . Comm. of XXIII Intrl. Dairy Congress . Vol. II , Oct. 8–12 , pp. 537 Montreal
- McMahon , D.J. , Oberg , C.J. and McManus , W. 1993 . Functionality of Mozzarella cheese . Australian J. Dairy Technol. , 48 : 99 – 104 .
- Lucey , J.A. and Fox , P.F. 1993 . Importance of Calcium and Phosphate in Cheese Manufacture: A Review . J. Dairy Sci. , 76 : 1714 – 1724 .
- Guinee , T.P. , Feeney , E.P. , Auty , M.A.E. and Fox , P.F. 2002 . Effect of pH and Calcium Concentration on Some Textural and Functional Properties of Mozzarella Cheese . J. Dairy Sci. , 85 : 1655 – 1669 .
- Metzger , L.E. , Barbano , D.M. , Kindstedt , P.S. and Guo , M.R. 2001 . Effect of Milk Preacidification on Low Fat Mozzarella Cheese: II Chemical and Functional Properties during Storage . J. Dairy Sci. , 84 : 1348 – 1356 .
- Joshi , N.S. 2003 . Understanding the Role of Calcium on Functional, Rheological and Structural Characteristics of Mozzarella Cheese , Brookings, SD : PhD Thesis, South Dakota State University .
- Upreti , P. 2005 . Understanding the Role of Calcium, Phosphorous, Residual Lactose, and Salt-to-moisture Ratio on Cheddar Cheese Characteristics , St. Paul, MN : Ph.D. Dissertation, University of Minnesota .
- Bradley , R.L. Jr. and Vanderwarn , M.A. 2001 . Determination of Moisture in Cheese and Cheese Products . J. AOAC. , 84 : 570 – 592 .
- Atherton , H.V. and Newlander , J.A. 1977 . “ Tests for Fat: Babcock, Gerber and Mojonnier ” . In Chemistry and Testing of Dairy Products , 4th , 71 – 116 . Westport, CT : AVI Publ. Co., Inc. .
- Kapoor , R. and Metzger , L.E. 2004 . Evaluation of Salt Whey as an Ingredient in Process Cheese . J. Dairy Sci. , 87 : 1143 – 1150 .
- Brooks , I.B. , Luster , G.A. and Easterly , D.G. 1970 . A Procedure for the Rapid Determination of the Major Cations in Milk by Atomic Absorption Spectrophotometry . Atomic Absorption Newsletter , 9 ( 4 ) : 93 – 94 .
- Association of Official Analytical Chemists, International . 1995 . Official Methods of Analysis , 16th , Arlington, VA : AOAC .
- Zeppa , G. , Conterno , L. and Gerbi , V. 2001 . Determination of Organic Acids, Sugars, Diacetyl, and Acetoin in Cheese by High-performance Liquid Chromatography . J. Agric. Food Chem. , 49 : 2722 – 2726 .
- SAS® . 1999 . Users' guide: Statistics , 8.0 , Cary, NC : SAS Inst. Inc. .
- Venugopal , V. and Muthukumarappan , K. 2003 . Rheological Properties of Cheddar Cheese during Heating and Cooling . International J. Food Prop. , 6 : 99 – 114 .
- Sutheerawattananonda , M. 1998 . Physicochemical Properties of Process Cheese; Influence on Meltability , St. Paul, MN : PhD Thesis, University of Minnesota .
- Rayan , A.A. , Kalab , M. and Ernstrom , C.A. 1980 . Microstructure and Rheology of Process Cheese . Scanning Electron Micros. , 3 : 635
- Gupta , S.K. , Karahadian , C. and Lindsay , R.C. 1984 . Effect of Emulsifier Salts on Textural and Flavor Properties of Processed Cheeses . J. Dairy Sci. , 67 : 764
- Schroeder , C.L. , Bodyfelt , F.W. , Wyatt , C.J. and McDaniel , M.R. 1988 . Reduction of Sodium Chloride in Cheddar Cheese: Effect on Sensory, Microbiological, and Chemical Properties . J. Dairy Sci. , 71 : 2010 – 2020 .
- Pastorino , A.J. , Hansen , C.L. and McMahon , D.J. 2003 . Effect of Salt on Structure-function Relationships of Cheese . J. Dairy Sci. , 86 : 60 – 69 .