Abstract
Entrapment of lipid in glass forming matrices in freeze-drying was investigated. Dispersion of lipid in aqueous solutions of three different sugars was formed by addition of protein and using homogenisation process. Two different levels of proteins in the ratios of 9:1 and 8:2 (sugar:protein) were used. Three different homogenisation processes were used to vary the emulsion droplet size of dispersed lipid. A novel ultra high-pressure homogenisation treatment with pressure levels up to 255 MPa (5MPa in second stage) was used to form emulsion before freeze-drying. The non-fat solids formed a glass in freezing which led to entrapment of dispersed oil. Ultra high-pressure homogenisation at pressure levels higher than 155 MPa caused alterations in emulsifying properties of sugar/gelatin systems. Changes in emulsifying properties of encapsulation matrices affected entrapment of dispersed lipid components. Droplet size of the dispersed phase was a significant factor for encapsulation efficiency. Emulsions with smaller droplet diameter gave higher entrapped amounts of lipid than emulsions with larger droplets. The glass forming properties and physical state of freeze-dried matrices were also determined. Knowledge of glass forming properties of an encapsulation matrix is needed for entrapment and predicting the stability and release properties of entrapped components.
INTRODUCTION
Encapsulation of dispersed particles in soluble solids is a common phenomenon in dehydration processes. Encapsulation occurs as a result of formation of a solid amorphous continuous matrix around dispersed components.[Citation1] It is used to protect sensitive components from environmental factors such as water, light and it may also facilitate controlled release of entrapped active compounds.
Various methods for encapsulation of food ingredients are available.[Citation2,Citation3,Citation4] Among them, freeze-drying and spray drying often give a high entrapment of flavours and provide excellent product characteristics.[Citation1] The mechanism behind entrapment of volatiles in freeze and spray drying is similar to each other. A selective diffusion concept was proposed by Thijssen and Rulkens[Citation5] explaining the mechanism of entrapment of dispersed molecules in spray drying. It was proposed that the diffusion coefficient of volatile components decreased at a higher rate than the diffusion coefficient of water during drying and it was also related to size of molecules. Entrapment of dispersed molecules inside micro-regions (consisting of highly concentrated solute) was proposed by Flink and Karel,[Citation6] explaining the entrapment of dispersed components in freeze-drying. This mechanism was based on entrapment of volatile components in amorphous solutes followed by freeze-drying.
Entrapment of an encapsulated compound inside a matrix depends upon various factors and much research has been done to study factors effecting entrapment of dispersed components in dehydration processes. Properties of dispersed molecules, encapsulation matrices,[Citation7,Citation8] and the emulsion,[Citation9,Citation10,Citation11] together with the spray drying process conditions,[Citation12,Citation13,Citation14] and the powder morphology during and after drying[Citation15] have been considered.
Droplet size of the dispersed phase in emulsion is a significant factor affecting entrapment of dispersed compounds. Advantages of preparing fine emulsions prior to spray drying have been reported.[Citation9,Citation10,Citation11] It has been suggested that entrapment of volatiles during encapsulation in spray drying could be enhanced by reducing the mean droplet size of the dispersed phase during emulsification.
Various homogenisation methods can be used to reduce the droplet size in emulsion preparation, namely high speed mixing up to 13,500 rpm/min, high-pressure (up to 50 MPa) homogenisation, microfludization, and ultrasound emulsification. Ultra high-pressure homogenisation up to 350 MPa has received considerable attention since it represents important innovations in texture modifications of emulsions for food products.[Citation16] Studies have been focused on emulsifying and rheological properties of emulsions produced by ultra high-pressure homogenisation.[Citation16,Citation17]Entrapment of the dispersed phase in emulsion dehydration is also affected by the physical state of an encapsulation matrix. Dispersed molecules are entrapped in a continuous solids phase forming during removal of water in dehydration.[Citation1,Citation18] The solid phase is in an amorphous state and tends to undergo time-dependent changes.[Citation18] Amorphous solids may crystallize due to increases in water content or temperature. Crystallization of amorphous solids often leads to release of entrapped compounds. Release of encapsulated methyl linoleate in amorphous lactose-gelatin mixture was caused by lactose crystallization.[Citation19] Similar results were reported by Labrousse, Roos, and Karel.[Citation20] Amorphous solids are stable, however, as solid, glassy materials.[Citation1,Citation21] The glassy state exhibits an extremely high viscosity and any changes occur slowly.[Citation1] Due to limited mobility of molecules in a glassy state, encapsulated compounds in glassy matrices are stable and deteriorative changes are often negligible. Amorphous solids of a number of food components exist in their glassy state at low water activities at ambient temperature.[Citation1]
The objective of the present study was to investigate entrapment of lipid in glassy matrices of sugar-gelatin systems and to study effects of emulsion droplet size on lipid encapsulation in freeze-drying. In the present study, olive oil was used as a model lipid because it is a food lipid, easily available and provided a liquid model material for a dispersed phase in an encapsulation study.
MATERIALS AND METHODS
Preparation of Emulsions
Lactose, maltose, sucrose and gelatin (from bovine skin, type B, bloom value ∼225) from Sigma-Aldrich Chemie Gmbh, Steinheim, Germany) were mixed in two different ratios: 9:1 and 8:2 (sugar:gelatin). Mixtures were dissolved in de-mineralised water at 45°C to 20% total solids concentration. The solutions were cooled to room temperature and olive oil (purchased from a local market) was added in to the solutions to obtain a ratio of 2:1 (total solids:lipid). These mixtures were subjected to three different homogenisation processes; (i) mixing at 13,500 RPM/2 min using ultra-turrax T25 (Janke & Kunkel Ika-Labortechnik, Germany); (ii) two stage high-pressure homogenisation process (21 MPa in first stage and 4 MPa in second stage) using a laboratory homogenizer (Model APV 1000, APV Homogenizers, AS, Albertslund, Denmark); and (iii) two stage ultra high-pressure homogenisation process (55–255 MPa in first stage and 5 MPa in second stage) using an ultra high-pressure homogenizer (Model nG7400H: 320, Stansted Fluid Power Ltd, Stansted, Essex, UK), to obtain emulsions with a range of dispersed phase droplet sizes.
Emulsion Droplet Size Measurements
Droplet size of the freshly prepared emulsions was determined by laser diffraction technique (Malvern Mastersizer S, Malvern Instruments Ltd. Malvern, UK). Calculation of the droplet size distribution was based on a refractive index of 1.46. Distilled water was used as dispersion medium. Sauter mean (D32) was considered as the mean diameter. It is the volume-surface average diameter of emulsion droplets, expressed in micrometers. The volume size distribution was calculated from the intensity of light diffracted at each angle using Mie theory.
Optical Microscopy
A small droplet of homogenised solution was placed between a slide and a cover slip and loaded on an Olympus light (BX-51) microscope. A drop of immersion oil was added on to the cover slip to observe the slide at magnification level of 100X. Images were acquired using an optical camera (Olympus, Japan), attached to the microscope.
Freeze-Drying of Homogenised Emulsions
The homogenised mixtures were frozen in petri dishes at −20°C for 12 h and subsequently at −80°C for 24 h and freeze-dried (32–34 h) using a laboratory freeze-dryer (Lyovac GT2 freeze-dryer; Amsco Finn-Aqua GmH, Hürth, Germany), operating at p < 0.1 mbar (ice T < −45°C). The dried emulsions were stored over P2O5 in vacuum-sealed desiccators at room temperature for at least one week for removal of residual water.[Citation22] For further experiments, the dried emulsions were converted into powder with a pestle and mortar.
Determination of Surface Oil
Oil present on the surface of the freeze-dried materials was determined by hexane washing. One gram of the material was mixed with 100 ml of hexane in conical flasks (250 ml capacity). Conical flasks sealed with glass stopper were shaken using an orbital shaker (Gallenkamp, UK) operating at 200 rpm for 30 min at room temperature. Hexane was separated from the mixture by filtration through ordinary filter paper. Hexane was evaporated by heating and residual oil was quantified by weighing. Analyses were performed in triplicate and average values are reported.
Determination of Encapsulated Lipid
The powdered materials obtained after washing the surface oil with hexane were used to determine the amount of encapsulated lipid. Amount of encapsulated lipid was quantified by the Röse-Gottlieb method.[Citation23] One gram of powdered material was dissolved in 10 ml of de-mineralised water, followed by addition of 1.5 ml of ammonia solution and 10 ml of ethanol. This mixture was mixed with 50 ml of solvent consisting of petroleum ether and diethyl ether (1:1). A few drops of phenolphthalein indicator were also added to distinguish between aqueous and fat phase. Mixture was shaken for 20 minutes manually in a separating funnel. After completion of mixing, solvent was separated from the aqueous phase. This operation was repeated four times. Solvent collected after complete extraction was allowed to evaporate until the extracted lipid achieved a constant weight. Analyses were performed in triplicate and average values are reported.
Encapsulation efficiency was calculated on the basis of following formula:
Determination of Glass Transition Temperature
Glass transition temperature of the freeze-dried matrices (prepared without lipid) was measured using differential scanning calorimetry (DSC, Mettler Toledo 821e, Schwerzenbach, Switzerland; with liquid N2 cooling). Samples of dehydrated materials (4 to 15 mg) were prepared in pre-weighed DSC aluminium pans (40 μl; Mettler Toledo-27331, Switzerland) and stored in open pans for at least one week at room temperature in evacuated desiccators over P2O5. Triplicate samples of each material were analysed. An empty pan was used as a reference. The DSC was calibrated for temperature using n-hexane (melting point, −95.0°C), mercury (melting point, −38.8°C), water (melting point, 0.0°C), gallium (melting point, 29.8°C) and indium (melting point, 156.6°C) and for heat flow using n-hexane (ΔHm, 151.8 J/g), mercury (ΔHm, 11.4 J/g), water (ΔHm, 334.5 J/g) gallium (ΔHm, 80 J/g) and indium (ΔHm, 28.45 J/g). The samples were scanned first over the glass transition region at 5°C/min, then cooled at 10°C/min to below glass transition, and a second heating scan at 5°C/min was run to well above the glass transition range. Anhydrous samples were scanned using pans with punctured lids to allow evaporation of any residual water during the measurement. Glass transition temperature (Tg) was determined using STARe thermal analysis software, version 6.0 (Mettler Toledo, Switzerland).
Scanning Electron Microscopy
The electron micrographs were obtained using a scanning electron microscope (JEOL, JSM-5600, Tokyo, Japan). A small amount of finely ground material was scattered evenly onto the surface of an aluminium stub covered with a 12 mm diameter carbon tab. Excess material was removed using dry compressed air, and the sample was sputter coated with a thin conductive film of gold for approximately 2 min in a sputter coater (S150B Edwards sputter coater, Sussex, UK). The stub containing the coated sample was then placed in the specimen chamber under vacuum. Accelerated electron beam was directed onto the sample and the image was produced. The accelerating voltage used was 20 keV.
X-Ray Diffraction Analysis
X-ray diffraction (XRD) of freeze-dried powdered materials was performed using a Philips X'pert PRO MPD PW3040/60 X-ray diffractometer (P Analytical Ltd, UK) with nickel filtered copper CuKα radiation (λ = 1.542 Å) as the X-ray source. The CuKα diffractometer anode was operated with an anode current of 35 mA and an accelerating voltage of 40 kV. Sample preparation consisted of grinding the material to a powder (< 45 μm) and loading it into PW1814/32 steel (Nickel finish) and PW1817/32 zero background holder (P Analytical Ltd., UK). The zero background holder was made from single crystal silicon with dimensions of 32 mm and 2 mm thickness. Samples were slightly e (75 × 25 mm) and scanned at diffraction angles (2θ) at 5°−40° using a step size of 0.0167° 2θ and a scan speed of 0.0356° 2θ/s. The samples were rotated at 10Hz during analysis. Scans were recorded every 17 min during the measurements.
All experiments were done in triplicate and average values reported.
RESULTS AND DISCUSSION
Emulsion Droplet Size Distribution
The volume percent data of droplet sizes were plotted against the emulsion droplet diameter. Mean diameter (D32) of emulsion droplet in all sugar/gelatin combinations varied with the type of homogenisation process. Highest emulsion droplet sizes were observed when emulsions were mixed with ultra-turrax. Sizes varied from 5.1 to 18.9 μm (). Emulsion droplet size distribution in the emulsions is shown in . Mean droplet size was at minimum (5.1 μm) in the emulsions consisting of sucrose and gelatin in the ratio of 8:2 (sugar:gelatin) and at highest (18.9 μm) in the emulsions consisting of maltose and gelatin in the ratio of 9:1 (sugar:gelatin). It might be due to better emulsifying properties of sucrose/gelatin mixtures than maltose/gelatin mixtures. Emulsions subjected to high-pressure (25 MPa, 4MPa in second stage) homogenisation yielded lower emulsion droplet sizes in comparison to the emulsions subjected to simple shear mixing (). Emulsion droplet sizes were in the range of 0.8 to 1.2 μm. Mean droplet size was at minimum (0.8 μm) in the emulsions consisting of lactose and gelatin in the ratio of 9:1 (sugar:gelatin) and at highest (1.2 μm) in the emulsions consisting of sucrose and gelatin in the ratio of 8:2 (sugar:gelatin). Droplet sizes of the emulsions homogenised at ultra high pressure (55–255 MPa) ranged from 0.3 to 0.7 μm () and decreased with increases in homogenisation pressure. Minimum droplet sizes were obtained at homogenisation pressure levels of 105 MPa and 155 MPa. This may be attributed to the stress required to break down the lipid droplets in uniform fine droplets. Emulsion droplet size generally decreased with increased homogenisation pressure, when sufficient concentration of emulsifier was present to completely cover the entire lipid droplet surfaces formed in homogenisation process.[Citation24] Proteins are surface-active molecules and often used as emulsifiers in oil-in-water emulsions. Proteins adsorb to the surfaces of freshly formed oil droplets during homogenisation of oil-water-protein mixtures, where they facilitate further droplet disruption by lowering the interfacial tension and prevent droplet coalescence by forming protective membranes around the droplets.[Citation25] A sufficient amount of protein should be present in an emulsion to cover all the newly formed droplets in homogenisation process.
Table 1 Effect of different homogenisation processes on emulsion droplet size distribution of sugar/gelatin systems
Figure 1 (a) Effect of mixing at 13,500 rpm/2min and (b) high-pressure homogenisation (25 MPa, 4 MPa in second stage) on emulsion droplet size distribution in sugar/gelatin solutions.
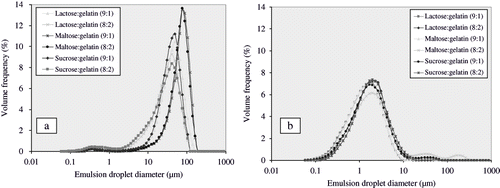
Effect of ultra high-pressure (55–255 MPa) homogenisation on emulsion droplet size distribution is shown in . It was observed that all emulsions showed a broad tail in the emulsion droplet distribution at pressure levels higher than 100 MPa. This may be due to recoalescence of the newly formed fine lipid droplets in the homogenisation process or very shortly afterwards.[Citation24,Citation26] MacClement[Citation26] proposed that the residence time of emulsifying molecules may not be sufficient in the valve of homogeniser to allow their adsorption on the entire lipid droplet surface available before droplet-droplet collision occurs. The high shear forces acting on emulsions in homogenisers might also play a role on the distribution size of the lipid droplets, because the impact forces that act on lipid droplets as the results of a collision might be sufficient to cause the disruption of interfacial membrane formed by emulsifying molecules. However, Floury et al.,[Citation24] suggested that recoalescence of droplets of dispersed phase might be due to loss of emulsifying property of proteins, because of the high stress and rise in temperature exerted by emulsifying molecules in the homogenisation valve.
Effect of Emulsion Droplet Size on Surface Oil Content
Oil present on the surface of freeze-dried matrices is termed as surface oil. The surface oil can be easily oxidised and give off-flavours, on exposure to air. The amount of surface oil is a very important factor for storage stability. Amounts of surface oil present in the freeze-dried matrices are given in . Surface oil varied with the type of homogenisation process. It was at highest (91.6% of initial added amount) in the emulsion consisting of lactose:gelatin (9:1), homogenised using ultra-turrax at 13,500 rpm/2 min. Surface oil was lowest (0.4% of initial added amount) in the emulsion consisting of sucrose:gelatin (8:2) and homogenised at 155 MPa (5 MPa) in second stage, using an ultra high-pressure homogeniser. It seems that an increase in homogenisation pressure led to a decrease in emulsion droplet diameter and gave a low surface oil content. It can be seen in as well that surface oil content decreased with decreases in emulsion droplet size. Surface oil content decreased from 7.7% to 0.4%, when emulsion droplet size decreased from 1.2 to 0.3 μm. Emulsions with smaller droplet sizes decreased surface oil present on the freeze-dried matrices. Surface oil content was also related to the oxidation of oil. It is believed that surface oil is more susceptible to oxidation in comparison to encapsulated lipid.[Citation9,Citation11]
Table 2 Effect of different homogenisation processes on surface oil content of sugar/gelatin systems
Effect of Emulsion Droplet Size on Lipid Entrapment
Entrapment of dispersed molecules in freeze-drying process is affected by emulsion droplet sizes. Smaller emulsion droplet sizes often yield better entrapment levels than those of larger sizes.[Citation27] Entrapment levels of lipid in freeze-drying of emulsions consisting of sucrose, lactose, maltose and gelatin in two different ratios of 9:1 and 8:2 (sugar:gelatin) is listed in . Entrapment level ranged from 8.4% to 99.6% of initially added amount of lipid. It was observed that smaller emulsion droplet sizes provided better entrapment of lipid than larger sizes (). These results were in agreement with the previous findings by Kaushik and Roos.[Citation27]
Table 3 Effect of different homogenisation processes on encapsulation efficiency of sugar/gelatin systems
Effect of Ultra High-Pressure (55–255 MPa, 5MPa in Second Stage) Homogenisation
Effect of ultra high-pressure homogenisation on emulsion droplet size distribution is shown in . It was observed that all emulsions homogenised at the pressure level of 155 MPa, gave lowest amounts of surface oil in freeze-drying ( and ) except the emulsion consisting of lactose and gelatin. Emulsions consisting of lactose and gelatin gave lowest amounts of surface oil, when homogenised at 105 MPa followed by freeze-drying. Further increases in homogenisation pressure led to decreases in emulsion droplet size (), but amount of surface oil in freeze-drying was increased (). This could be related to the degradation of polymer structure due to high shear stress and rise in temperature. This degradation of structure possibly led to coalescence of homogenised lipid droplets in the emulsions, immediately after leaving the homogenisation valve (). The coalescence of the homogenised lipid droplets was evident in the images acquired during the optical microscopy of homogenised emulsions. It can be seen that the coalescence did not take place in the emulsions homogenised at a pressure of 55 MPa (5 MPa in second stage). Emulsions consisting of sucrose-gelatin and maltose-gelatin exhibited coalescence at pressure levels > 150 MPa. However, emulsions consisting of lactose-gelatin showed coalescence of homogenised lipid droplets at homogenisation pressure level > 100 MPa. We assume that the coalescence of homogenised lipid droplets led to higher surface oil content and lower entrapment of lipid in freeze-drying. Since large emulsion droplets often lead to decreased entrapment of dispersed molecules in dehydration processes.[Citation27]
Figure 4 Microscopic images of homogenised emulsions (a) Maltose:gelatin (9:1) homogenised at 55 MPa, (b) Maltose:gelatin (9:1) homogenised at 255 MPa, (c) Sucrose:gelatin (8:2) homogenised at 205 MPa.
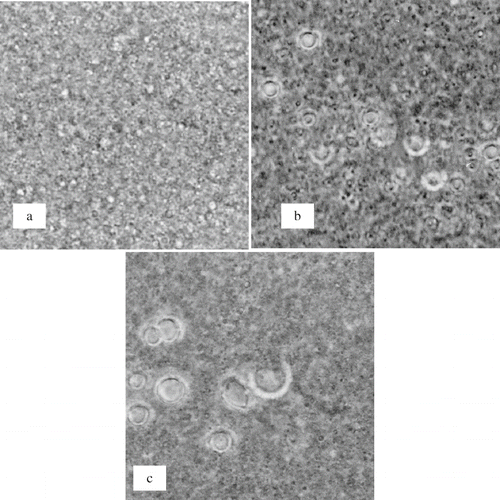
In past, it was reported that high shear stress and rise in temperature in ultra high pressure homogenisation caused degradation of the structure of high molecular weight compounds, such as proteins and gums.[Citation24,Citation28,Citation29] The high shear forces in the valve of ultra high-pressure homogeniser may present enough energy to disrupt covalent bonds of the polymeric structure. These structural changes led to loss of emulsifying and stabilising ability of emulsifiers. Floury et al.[Citation16] studied effects of high-pressure (20–350 MPa) homogenisation on degradation of methylcellulose in emulsions. It was observed that homogenisation at a pressure of 350 MPa, led to reduction of average molecular weight of methylcellulose one fifth of a non-homogenised molecule. Alterations in the molecular weight of a polymer affect its functional attributes, such as emulsifying and stabilising properties. Decrease in the entrapment of lipid in the emulsions homogenised at pressure levels higher than 150 MPa could be related to this phenomenon. Floury et al.[Citation16] reported that the emulsion droplet size of oil in emulsions consisting of methylcellulose as an emulsifier was decreased with increases in homogenisation pressure from 20 to 350 MPa. However, degradation of the polymer structure at pressure levels above 150 MPa resulted in losses of emulsion stabilising properties of methycellulose. This degradation at pressure levels above 150 MPa resulted in the coalescence of smaller emulsion droplets immediately after exiting the homogenisation valve. We assume that the same phenomenon occurred in our materials at pressure levels above 100 MPa. The coalescence of homogenised emulsion droplets at pressure levels above 100 MPa led to decreased entrapment of lipid in freeze-drying.
Physical Characteristics
Glass transition temperatures () of all freeze-dried encapsulation matrices showed that matrices were in an amorphous state. Glass forming properties of a matrix are helpful to predict the release and protection from oxidation of entrapped compounds in these matrices. Entrapped compounds are protected well in the glassy state of a matrix due to very low mobility of compounds attributed to extremely high viscosity of glassy state. Glass transition temperature of all the matrices was well above room temperature (), which suggested that the anhydrous matrices were in glassy state at room temperature. In accordance to the findings of Righetto and Netto,[Citation34] stickiness was also avoided as the materials were below Tg. Glass transition temperatures of lactose-gelatin, maltose-gelatin and sucrose-gelatin mixtures were higher () than glass transition temperature of pure lactose (101°C), maltose (87°C), and sucrose (62°C) reported by Roos.[Citation30] It was possibly due to addition of protein (gelatin) to sugars since addition of high molecular compounds to sugars often causes rise in glass transition temperature.[Citation1] The amorphous state of freeze-dried matrices was also confirmed by x-ray diffraction technique (). These results were in agreement with previous findings.[Citation33] The physical state of an encapsulation matrix is a critical factor affecting entrapment of dispersed compounds in drying processes. Amorphous glasses formed due to removal of water in dehydration processes retain dispersed compounds better that crystallised matrices.[Citation1] Electron micrographs of the freeze-dried matrices were also obtained. It was observed that amorphous encapsulation matrices possessed a glassy structure (). The outer structure of all combinations of sugars and gelatin was smooth and free of dents. The microstructure of a food matrix plays an important role in entrapment of dispersed molecules.[Citation31,Citation32] Presence of cracks and dents on surface of dried particles adversely affects powder flow-ability and reconstitution properties.
Table 4 Glass transition temperatures of freeze-dried sugar/gelatin matrices
CONCLUSIONS
Dispersed lipid was entrapped in the glassy matrix formed in freeze-drying of sugar-gelatin systems. The emulsion droplet size influenced the entrapment of lipid in freeze-drying of sugar-protein emulsions. The results indicated that it was beneficial to obtain small emulsion droplet sizes for high entrapment in freeze-drying. The smaller droplet sizes yielded freeze-dried powders with low surface oil contents and higher entrapment of lipid than systems with larger emulsion droplet sizes. Ultra high-pressure (55–255 MPa) homogenisation could be a useful technique to improve efficiency of an encapsulation process. High levels of entrapment could be achieved by homogenising emulsions up to 155 MPa pressure. The high pressure, high shear and rises in temperature in ultra high-pressure homogenisation could also damage the functional properties like emulsifying and stabilising ability of emulsifiers. Therefore, more research is needed to study the effects of ultra high-pressure homogenisation on the physicochemical properties of emulsions.
ACKNOWLEDGEMENTS
The study was carried out with the financial support of PRTL1 Cycle 3 of the Higher Education Authority of Ireland.
REFERENCES
- Roos , Y.H. 1995 . Phase Transitions in Foods , 1 – 347 . San Diego, CA : Academic Press .
- Dziezak , J.D. 1988 . Microencapsulation and encapsulated ingredients . Food Technology , 42 ( 4 ) : 136 – 151 .
- Shahidi , F. and Han , X.Q. 1993 . Encapsulation of food ingredients . Critical Reviews Food Science and Nutrition , 33 : 501 – 547 .
- Gibbs , B.F. , Kermasha , S. , Alli , I. and Mulligan , N. 1999 . Encapsulation in the food industry: a review . International Journal of Food Science and Nutrition , 50 : 213 – 224 .
- Thijssen , H.A.C. and Rulkens , W.H. 1968 . Retention of aroma in drying food liquids . De Ingenieur , 47 : 45 – 56 .
- Flink , J. and Karel , M. 1970 . Retention of organic volatiles in freeze-dried solutions of carbohydrates . Journal of Agriculture and Food Chemistry , 18 ( 2 ) : 295 – 297 .
- Inglett , G.E. , Gelbman , P. and Reineccius , G.A. . Encapsulation of orange oil: use of oligosaccharides from α-amylase modified starches of maize, rice, cassava and potato . Flavour Encapsulation . ACS symposium series , Edited by: Risch , S.J. and Reineccius , G.A. Vol. 370 , pp. 29 – 36 . Washington, D.C. : American Chemical Society .
- McNamee , B.F. , O'Riordan , E.D. and O'Sullivan , M. 1998 . Emulsification and microencapsulation properties of gum Arabic . Journal of Agriculture and Food Chemistry , 46 : 4551 – 4555 .
- Risch , S.J. and Reineccius , G.A. . Spray-dried orange oil-Effect of emulsion size on flavour retention and shelf life . Flavour Encapsulation . ACS symposium series , Edited by: Risch , S.J. and Reineccius , G.A. Vol. 370 , pp. 67 – 77 . Washington, D.C. : American Chemical Society .
- Mongenot , N. , Charrier , S. and Chalier , P. 2000 . Effect of ultrasound emulsification on cheese aroma encapsulation by carbohydrates . Journal of Agriculture and Food Chemistry , 48 : 861 – 867 .
- Soottitantawat , A. , Yoshii , H. , Furuta , T. , Ohkawara , M. and Linko , P. 2003 . Microencapsulation by spray drying: influence of emulsion size on the retention of volatile compounds . Journal of Food Science , 68 ( 7 ) : 2256 – 2262 .
- Anker , M.H. and Reineccius , G.A. . Encapsulation of orange oil: influence of spray dryer air temperatures on retention and shelf life . Flavour Encapsulation . ACS symposium series , Edited by: Risch , S.J. and Reineccius , G.A. Vol. 370 , pp. 78 – 86 . Washington, D.C. : American Chemical Society .
- Rosenberg , M. , Kopelman , I.J. and Talmon , Y. 1990 . Factors affecting retention in spray drying microencapsulation of volatile materials . Journal of Agriculture and Food Chemistry , 38 : 1288 – 1294 .
- Bhandari , B.R. , Dumoulin , E.D. , Richard , H.M.J. , Noleau , I. and Lebert , A.M. 1992 . Flavour Encapsulation by spray drying: Application to citral and linalyl acetate . Journal of Food Science , 57 ( 1 ) : 217 – 221 .
- Moreau , D.L. and Rosenberg , M. 1996 . Oxidative stability of anhydrous milk fat microencapsulated in whey proteins . Journal of Food Science , 61 : 39 – 43 .
- Floury , J. , Desrumaux , A. , Axelos , M.A.V. and Legrand , J. 2003 . Effect of high pressure homogenisation on methylcellulose as food emulsifier . Journal of Food Engineering , 58 : 227 – 238 .
- Puppo , M.C. , Spernoni , F. , Chapleau , N. , De Lamballerie , M. , Anon , M.C. and Anton , M. 2005 . Effect of high-pressure treatment on emulsifying properties of soybean proteins . Food Hydrocolloids , 19 : 289 – 296 .
- Roos , Y. and Karel , M. 1991 . Applying state diagrams in food processing and development . Food Technology , 45 ( 12 ) : 66 – 71 .
- Shimada , Y. , Roos , Y. and Karel , M. 1991 . Oxidation of methyl linoleate encapsulated in amorphous lactose based food model . Journal of Agriculture and Food Chemistry , 39 ( 4 ) : 637 – 641 .
- Labrousse , S. , Roos , Y. and Karel , M. 1992 . Collapse and crystallization in amorphous matrixes with encapsulated compounds . Science des Aliments , 12 : 757 – 769 .
- Roos , Y. and Karel , M. 1993 . “ Effects of glass transitions on dynamic phenomena in sugar containing food systems ” . In Glassy state in foods , Edited by: Blanshard , J.M.V. and Lillford , P.J. 207 – 222 . Nottingham : Nottingham University Press .
- Roos , Y. and Karel , M. 1992 . Crystallization of amorphous lactose . Journal of Food Science , 57 ( 3 ) : 775 – 777 .
- Richardson , G.H. 1985 . Standard methods for the examination of dairy products , 15th , Washington, D.C. : American Chemical Society .
- Floury , J. , Desrumaux , A. , Axelos , M.A.V. and Lardières , J. 2000 . Effect of high-pressure homogenization on droplet size distributions and rheological properties of model oil-in-water emulsions . Innovative Food Science and Emerging Technologies , 1 : 127 – 134 .
- Walstar , P. 2003 . Physical chemistry of foods , New York : Marcel Dekker .
- Mac Clement , D. J. 1999 . Food emulsions: principles, practice and techniques , Florida : CRC Press LLC .
- Kaushik , V. and Roos , Y.H. 2007 . Limonene encapsulation in freeze-drying of gum Arabic, sucrose and gelatin systems . LWT-Food Science and Technology , 40 ( 8 ) : 1381 – 1391 .
- Lagoueyte , N. and Paquin , P. 1998 . Effects of microfluidisation on functional properties of xanthan gum . Food Hydrocolloids , 12 : 365 – 371 .
- Floury , J. , Desrumaux , A. , Axelos , M.A.V. and Legrand , J. 2002 . Degradation of methylcellulose during ultra-high pressure homogenization . Food Hydrocolloids , 16 : 47 – 53 .
- Roos , Y. 1993 . Melting and glass transition of low molecular weight carbohydrates . Carbohydrate Research , 238 : 39 – 48 .
- Druaux , C. and Voilley , A. 1997 . Effect of food composition and microstructure on volatile flavor release . Trends in Food Science and technology , 8 : 364 – 368 .
- Goubet , I. , Le Quere , J.L. and Voiley , A.J. 1998 . Retention of aroma compounds by carbohydrates: influence of their physicochemical characteristics and of their physical state. A review . Journal of Agriculture and Food Chemistry , 46 : 1981 – 1990 .
- Roe , K.D. and Labuza , T.P. 2005 . Glass transition and crystallization of amorphous trehalose-sucrose mixtures . International Journal of Food Properties , 8 ( 3 ) : 559 – 574 .
- Righetto , A.M. and Netto , F.M. 2005 . Effect of encapsulating materials on water sorption, glass transition and stability of juice from immature acerola . International Journal of Food Properties , 8 ( 2 ) : 337 – 346 .