Abstract
Native barley starch, as well as its blends with corn, wheat, and rice starch at different ratios of 75:25, 50:50, 25:75 were examined in terms of morphology, thermal, pasting, rheological, and retrogradation properties. Amylose content varied between 10.9–41.4% in rice, corn, wheat, and barley while it ranged from 18.02–38.40% in blends of barley starch with rice, corn, and wheat. A rapid visco analyzer showed that barley starch and its blends having low amylose content exhibited higher peak viscosity, breakdown, and setback than the high-amylose-containing starches and their blends. Amylose content was found to be negatively correlated with swelling power while it exhibited nonlinear relationship with solubility index. The transmittance of starch suspension stored at 4°C decreased during storage up to 6 days. Barley starch granules were largest (<110 μm) in size followed by wheat (<30 μm), corn (<25μm) and rice (<20μm) starches. Gelatinization temperatures (To, Tp, Tc) and enthalpies of gelatinization (ΔHgel) of starches from different sources also differed significantly. Corn and rice starches showed higher transition temperatures in general than those from wheat and barley; however, they showed higher ΔHgel values. Barley starch showed a higher tendency towards retrogradation than the cereal starches. Barley starch showed highest peak G′, G″ and lower tan Ð than corn, rice and wheat starches during the heating cycle. This study showed that the magnitude of changes in their properties during blending depends on the amylase content and morphological characteristics.
INTRODUCTION
Starch is stored as discrete semicrystalline granules in higher plants. Starch consists of two main components: mainly linear amylose and highly branched amylopectin. In the area of carbohydrate polymers, starch is an important example that is currently enjoying an increased attention due to its usefulness in different food products. Starch is the major polysaccharide in plants and exists in the form of granules comprising of amylose and amylopectin chains in a relatively ratio of average 20:80.[Citation1] But the amylose content can vary from 17–65% depending on the botanical origin of the starch. The exact location of amylose within the granule interior and the extent and nature of its interaction with amylopectin is still unclear. The physicochemical properties and functional characteristics that are imparted to the starch systems and their uniqueness in various food applications vary with the biological origin.[Citation2] Starch granules vary in shape, size and composition depending on their botanical origin.[Citation3] Extracted starches from cereals are used extensively in food and non-food industries. These starches are physically and chemically modified to meet the properties demanded by industry. Morphological and ultra-structural changes during starch gelatinization have been observed by scanning electron microscopy (SEM) and transmission electron microscopy (TEM) in potato, waxy maize, normal maize, barley, oat, wheat, and cassava starches.[Citation4,Citation5] Morphological and structural changes at various temperature and moisture combinations have been shown to influence rheological behavior and functionality of starch gels.[Citation6,Citation7] Starches from various plant sources, such as wheat, maize, barley and rice, have received extensive attention in relation to structural and physicochemical properties.[Citation8,Citation9] Starch contributes greatly to the texture properties of many foods and is widely used in food and industrial applications as a thickener, colloidal stabilizer, gelling agent, bulking agent, water retention agent, and adhesive. Starches are good texture stabilizer and regulator in food systems,[Citation10] but limitations like low shear stress resistance, thermal resistance, thermal decomposition, and high retrogradation have limited their use in some industrial food applications. These shortcomings can be overcome by chemical and physical modification of starches.[Citation3] This can also be overcome by explore the possibility of blend the different starch sources for change starch properties comparable with modified one.
Native starch blends are increasingly applied in food industry to make starchy food with a desired rheological property, texture, storage stability, or to replace modified starch.[Citation11] In contrast to single starch systems, starch blends generally exhibit unique Visco-elastic or physical properties that cannot be directly interpreted by the final apparent amylose content or mixing ratio. [Citation11,Citation12] This may be because of the differences in swelling and solubility properties between dissimilar starches,[Citation13,Citation14] and the uncertainties involved in intermolecular association.[Citation15,Citation11] The interactions between starch materials that control the texture-related structure of starch composites in food are dependent on the experimental or processing conditions. The modified starches generally exhibited better paste clarity, stability and increased resistance to retrogradation.[Citation16] In chemical starch modification, cross-linking and substitution are used to give thickeners that have the desired rheological properties during storage and shipment.[Citation17] Interest in new value-added products to the industry has resulted in many studies being carried out on the rheological and textural properties of corn and potato starches.[Citation18,Citation19,Citation20,Citation12] The objectives of the present study was to study the morphological, thermal, rheological, pasting and gel strength characteristics of barley starch and blended with corn, rice and wheat starch at different levels that was comparable as that of modified starch properties.
MATERIAL AND METHODS
Barley grains and high-amylose cultivar were procured from Punjab Agriculture University, Punjab-India. Barley grains were conditioned to 14% (db) moisture content. The barley grains were ground to flour in a laboratory centrifugal mill (Retsch GmbH, Germany) and passed through a 60-mesh (British standard-250 microns) sieve to obtain 180–220 micron flour. Barley starch was isolated by the method described.[Citation21] Wheat starch was isolated using the method[Citation22] of Lee et al. 2001. Corn and Rice starches were procured from S. D. Fine Chemicals Ltd. India. Analytical grade sodium hydroxide and hydrochloric acid were procured from Glaxo India Limited, Mumbai (India) and ethanol was obtained from Hayman Ltd., Essex (U.K).
Starch Blends
Starch from different cereal sources like Wheat, Rice, and Corn was blended with that from Barley in the ratios 75:25, 50:50, 25:75. These blends were accessed for the effect on physicochemical, morphological, thermal, rheological, retrogradation, and gel strength properties.
Physicochemical Properties
Amylose content of the starches was determined using the method described.[Citation23] Swelling power and solubility of starches were determined using 2% aqueous suspension of starch by the previous method.[Citation24] Light transmittance (%) of pastes from barley starch blended with other starches was measured by the method earlier described.[Citation25] A 2% aqueous suspension of starch was heated in a boiling water bath for 30 min with constant stirring. The suspension was cooled for one hour at 30°C. The samples were stored for five days at 4°C and % transmittance measured every 24 h at 640 nm against a water blank with a Shimadzu UV-1601 spectrophotometer (Shimadzu Corporation, Kyoto, Japan). Fluidity value was determined by the method described.[Citation26]
Morphological Properties
Scanning electron micrographs of Barley starch blended with other starches were obtained with a scanning electron microscope (Jeol JSM-6100, Jeol Ltd., Tokyo, Japan). Starch samples were suspended in ethanol to obtain a 1% suspension and were sprinkled on double stick tape fixed on an aluminum stub, and the starch was coated with gold-palladium (60:40). An accelerating potential of 5kV was used during micrography.
Thermal Properties
Thermal properties of blended starches were analyzed using DSC-821e (Mettler Toledo, Switzerland) equipped with a thermal analysis data station. Starch (3.5mg, dwb) was weighed into a 40-μl capacity aluminum pan (Mettler, ME-27331) and distilled water was added with the help of Hamilton micro-syringe to achieve a starch-water suspension containing 70% water. Samples were hermetically sealed and allowed to stand for 1 hour at room temperature before heating in DSC. The DSC analyzer was calibrated using indium and an empty aluminum pan was used as reference. Sample pans were heated at a rate of 10°C per min from 20 to 100°C for corn, wheat, rice, and barley starch. Onset temperature (To ); Peak temperature (Tp ); Conclusion temperature (Tc ) and Enthalpy of gelatinization (ΔH gel) were calculated.
Pasting Properties
Pasting properties were analyzed using a Rapid Visco-Analyzer (RVA) (model 3D; Newport Scientific Ltd, Sydney, Australia). Viscograms of starch was monitored using starch–water suspensions (6 and 8%, w/w, dry starch basis, 28.0 g total weight). Each suspension was tested under the same temperature–time conditions: heating from 50 to 95°C at 6°C/min (after an equilibration time of 1 min at 50°C), a holding period at 95°C for 5 min, cooling from 95 to 50°C at 6°C/min and a holding phase at 50°C for 2 min. The constant rotating speed of the paddle was 160 rpm. Pasting parameters were automatically computed and reported.
Rheological Properties
A small amplitude oscillatory rheological measurement was made for starches from barley starch blended with other starches, with a dynamic rheometer (Paar Physica, MCR-100, Anton Paar, Germany) equipped with plate and cone system (4 cm diameter). The gap size was set at 1000 μm. The strain and frequency were set at 2% and 5 Hz, respectively for all determinations. The dynamic rheological properties such as storage modulus (G′), loss modulus (G′′) and loss factor (Tan δ) were determined for starch and its blends. Starch suspensions of 20% (w/w) concentration were loaded on the ram of rheometer and covered with a thin layer of low-density silicone oil (to minimize evaporation losses). The starch samples were heated from 25°C to 85°C at the rate of 3°C/ min and cooled from 85°C to 25°C at the rate of 5°C/ min.
Retrogradation Properties
Starch suspension (2%, w/w) was heated at 85°C for 30 minutes in a temperature controlled water bath, followed by rapid cooling in an ice water bath to room temperature. The starch sample was stored for 24, 48, and 120 hours at 4°C. Syneresis was measured as % amount of water released after centrifugation at 5000 rpm for 15 minutes.
Gel Strength
An aqueous starch suspension (14%) barley starch blended with other starches was heated from 30–90°C and held for 20 min at 90°C, then cooled at 50°C. This cooked paste was used to prepare starch gels for compression testing. Gels were prepared using starches from different sources by slight modification of the earlier method.[Citation27] The cooked paste as described above was poured in a dish and cooled at 30°C for 1 h and stored in refrigerator at 4°C. After every 24 h, a piece of gel (1cm3) was cut and loaded on the base plate of the Texture analyzer (Lloyd Instruments LR 30K, UK). The flat probe (3.5-cm diameter) traveled a distance of 6 mm at a test speed of 25mm/min, for each compression. The maximum force (N) required to compress the sample was recorded.
Statistical Analysis
Data analysis for Duncan multiple comparison test and response optimization were done using Statistica statsoft ver8.0 statistical package.
RESULTS AND DISCUSSION
Physicochemical Properties
Amylose content of native starches was varied between 10.9–41.4% rice, corn, wheat and barley starches respectively, as barley starch blended with rice, corn, and wheat at different ratios the amylose content was varied in between 18.02–38.40%. The amylose content during blended was comes between 10–40% range, was important in this study .
Table 1 Summary of physicochemical properties of barley starch and their blends (n = 3)
The swelling power and solubility of native barley starch was 10.43g/g and 18.40, for wheat was 14.05g/g and 12.72, for corn was 14.185g/g and 11.12 and for rice was 17.74g/g and 9.92, respectively. As barley, starch blended with wheat, corn and rice swelling power increases as amylose content decreases and vice versa with solubility swelling power and solubility index was varied between 11.20–15.12g/g and 10.45–17.73 respectively shown in Table. 1. Both swelling power and solubility of all starches tested increased as the temperature increased. It has been reported that on the molecular level, the Swelling Power and solubility of the starch granule is influenced by many factors, including amylose to amylopectin ratio and contents, molecular mass of each fraction, degree of branching, conformation length of outer branch of amylopectin, and the presence of other components such as lipids and proteins.[Citation28] Waxy starch exhibited the highest degree of swelling, supporting the idea that reduced amylose content relates to greater swelling. Because the swelling behavior of cereals has been related to amylopectin,[Citation29] the high Swelling Power suggested a less rigid granular structure of waxy starch compared with that of non-waxy starches. In fact, it has been reported[Citation6] that waxy starches have a more open structure that allows rapid water penetration, swelling and solubility. Swelling power and Amylose content has negatively correlated with each other. The Fluidity values of native barley, wheat, rice and corn was 95.0, 92.0, 90.0, and 96.0, respectively; the fluidity values of all the starches depends upon their morphological characteristics. As size of the starch granules increases fluidity value also increases means it produce resistance toward floe behaviour Results are shown in .
The Transmittance (%) at 640 nm was measured from the 2% heated starch slurry was obtained up to 6 days stored at 4°C. the results showed that transmittance value was decreased during storage. In case of native barley starch transmittance value decreased from 70.14 to 3.0, for wheat was decreased from 81.6 to 9.3, for corn was decreased from 57.6 to 12.1 and in case of rice, was decreased from 63.11 to 7.3 as barley starch blended with these wheat, corn and rice starches at different levels results showed the same decreased trend as shown in Table. 1. It is well known that when starch suspension is heated, leaching of amylose (linear fraction) occurs, and upon cooling, micro-crystals are formed and turbidity appears.[Citation26] Factors responsible for lower transmittance in starches during storage have been previously identified by many researchers[Citation30,Citation25] and include aggregates made of leached amylose, amylase, and amylopectin chain length, intra or inter molecular bonding, granule swelling, and granule remnants. Starch gels undergo structural transformation in terms of chain aggregation, recrystallization during storage, and these changes are referred to as retrogradation. Short-term development of crystallinity is attributed to molecule organization and crystallization of the amylose fraction [Citation31]
Morphological Properties
Morphological characteristics, such as shape and size of the starch granules, exhibit significant differences. Barley starch granules are smooth–surfaced, oval, and irregular or cuboidal-shaped while corn, rice, and wheat starch granules are angular, pentagonal and angular; and spherical and lenticular–shaped, respectively. Corn, rice and wheat starch granules are less smooth–surfaced than barley starch granules. Barley starch granules are largest (<110 μm) in size followed by wheat (<30 μm), corn (<25 μm) and rice (<20 μm) starches. The variation in the size and shape of starch granules is attributed to the biological origin.[Citation2] The morphology of starch granules depends on the biochemistry of the chloroplast or amyloplast, as well as physiology of the plant[Citation32]. The granular structures of barley, corn, rice and wheat starches show significant variations in size and shape when viewed by SEM. Scanning electron micrographs of the starch granules from various plant sources. The granule size is variable and ranges from 1 to 110 μm. The average granule size ranges from 1–20 μm for small and 20–110 μm for large barley starch granules. The extent of variation in the granular structure of starches from cultivar to cultivar is significantly higher in barley. The average size of individual cornstarch granules ranges from 1–7 μm for small and 15–20 μm for large granules. The rice starch granules range from 3–5 μm in size. Barley starch granules have been observed to be oval and irregular or cuboidal in shape. The starch granules are angular-shaped for corn, and pentagonal and angular-shaped for rice. At maturity, wheat endosperm contains two types of starch granules: large A- and small B-type. A-type granules are disk-like or lenticular in shape with diameters ranging from 10 to 35 μm. On the other hand, B-type starch granules are roughly spherical or polygonal in shape, ranging from 1–10 μm in diameter. It has been observed under a scanning electron microscope, the surfaces of the granules from corn, rice and wheat appear to be less smooth than potato starch granules. Li et al.[Citation33] observed the presence of “pin holes” and equatorial grooves or furrows in large-sized corn starch granules. Fannon[Citation34] has shown the presence of large protuberances (200–500 μm) on the surface of potato starch granules, using atomic force microscopy. The individual granules, in the case of rice starch, develop into compact spherical bundles or clusters, known as compound granules, which fill most of the central space within the endosperm cells. Physicochemical properties, such as percent light transmittance, amylose content, swelling power and water–binding capacity were significantly correlated with the average granule size of the starches separated from different plant sources.[Citation35]
Thermal Properties
shows gelatinization properties determined using DSC. DSC parameters recorded were onset To, Peak Tp Final Tc gelatinization temperatures, gelatinization enthalpy ΔH. Gelatinization temperatures and enthalpies associated with gelatinization endotherms varied between starches. To was 59.79–70.12°C for native barley, wheat, rice and cornstarches and was varied between 58.11–68.56°C for barley starch blended with wheat, rice and corn at different ratios. Tp was 64.06–73.85°C for native barley, wheat, rice, and corn starches and was varied between 61.55–70.92°C for barley starch blended with wheat, rice and corn at different ratios and Tc was 68.42–78.20°C for native barley, wheat, rice, and corn starches and was varied between 67.14 barley, wheat, rice and corn starches and was varied between 67.14–74.33°C for barley starch blended with other, ΔHgel was 10.62–26.3J/g for native starches and 3.32–23.06J/g for blended starches (). Mixed starches showed lower onset and peak temperatures than native starches with the same amylose content. The gelatinized temperature range (Tc-To) of native starch was 8.0−16.7°C, whereas that of blended starch was 5.7–14.28°C. Waxy barley starch showed higher gelatinization temperature and enthalpy than normal barley starch.[Citation36] The enthalpy of gelatinization reflects the loss of molecular order[Citation37] and gelatinization temperature is considered a parameter of crystallite perfection.[Citation29] Because amylopectin plays a major role in starch granule crystallinity, the presence of amylose lower the melting temperature of crystalline regions and the energy for starting gelatinization.[Citation38] More energy is needed to intimate melting in the absence of amylose-rich amorphous regions.[Citation39] This correlation indicated that starch with higher amylose content has more amorphous region and less crystalline, lowering gelatinization temperature and endothermic enthalpy. Native and mixed starches with the same amylose contents as native starches showed clearly different gelatinization onset and peak temperature. The difference in gelatinization properties between native and mixed starches are due to varied homogeneity. Fredriksson[Citation40] reported that a wide temperature range implies a large amount of crystals with varied stability. The lack of homogeneity of ordered inside structures caused a broader gelatinization range.[Citation41]
Table 2 Summary of thermal properties, syneresis (%), and gel strength (N) of barley starch and their blends (n = 3)
Figure 1 Representative thermal endotherms of native Barley, Wheat, Corn, and Rice starches and their blends.
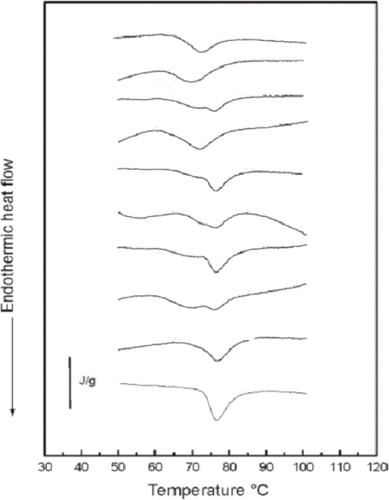
74.33 °C for barley starch blended with other, ΔHgel was 10.62–26.3J/g for native starches and 3.32–23.06J/g for blended starches (). Mixed starches showed lower onset and peak temperatures than native starches with the same amylose content. The gelatinized temperature range (Tc-To) of native starch was 8.0–16.7°C, whereas that of blended starch was 5.7–14.28°C. Waxy barley starch showed higher gelatinization temperature and enthalpy than normal barley starch.[Citation36] The enthalpy of gelatinization reflects the loss of molecular order[Citation37] and gelatinization temperature is considered a parameter of crystallite perfection.[Citation29] Because amylopectin plays a major role in starch granule crystallinity, the presence of amylose lower the melting temperature of crystalline regions and the energy for starting gelatinization.[Citation38] More energy is needed to intimate melting in the absence of amylose-rich amorphous regions.[Citation39] This correlation indicated that starch with higher amylose content has more amorphous region and less crystalline, lowering gelatinization temperature and endothermic enthalpy. Native and mixed starches with the same amylose contents as native starches showed clearly different gelatinization onset and peak temperature. The difference in gelatinization properties between native and mixed starches are due to varied homogeneity. Fredriksson[Citation40] reported that a wide temperature range implies a large amount of crystals with varied stability. The lack of homogeneity of ordered inside structures caused a broader gelatinization range.[Citation41]
Pasting Properties
Pasting viscosity profiles of starches analyzed by using RVA. RVA profiles varied with the type of starch and its blends in different ratios. The peak viscosity indicates the water holding capacity of starch and refers to the maximum viscosity reached during the heating and holding cycle. It can be affected by the molecular structure of amylopectin.[Citation42] Starch water concentration, lipids, residual proteins,[Citation43] granule size,[Citation44] and instrument operating conditions.[Citation45] The average peak viscosities were in the range of 345 to 1240 RVU for native barley shown in , wheat, rice and corn starches and were varied between 226.58–326.83 RVU for barley starch blended with wheat, rice and corn at different ratios. The pasting temperature of native barley starch was 77.75°C, for corn starch was 70.4°C, for rice starch was 75.25°C and for wheat starch was 66.4°C. When barley starch blend with cornstarch the pasting temperature varied between 73.1–75.1 and when blended with rice starch pasting temperature was varied between 70.5–73.8°C and with wheat starch pasting temperature was 70.50–71.85°C. The peak viscosity of native and blended starches was depend upon its amylose content, As amylose content increase from 10.9–41.4% the peak viscosity was also increased from 192.2 to 324.33 RVU for native and blended starches as shown in .
Figure 2 Representative Pasting profiles of native Barley, Wheat, Corn, and Rice starches and their blends.
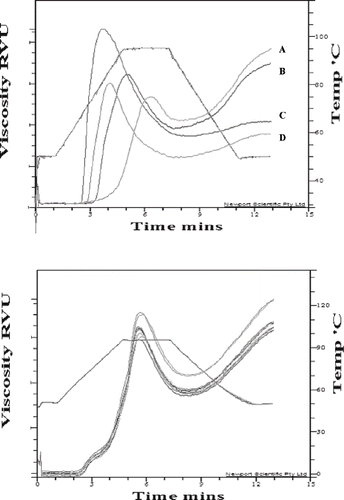
Table 3 Summary of pasting properties of barley starch and their blends (n = 3)
Pasting properties of starch are affected by amylose and lipid contents and by branch chain-length distribution of amylopectin. Amylopectin contributes to swelling of starch granules and pasting, whereas amylose and lipids inhibit the swelling.[Citation29] Furthermore, the amylopectin chain-length and amylose molecular size produce synergistic effects on the viscosity of starch pastes.[Citation46] Thus the effects of the structural features on the pasting properties of starches are rather complex. The RVA results showed that the pasting temperature of all starches were higher than the onset gelatinization temperatures (To) determined by DSC. The differences ranged between ∼3°C of rice and more than 30°C of wheat and barley starches. In general, waxy cereal starches had lower pasting temperatures, higher peak viscosity, and lower setback viscosity than the normal starch counterparts. The increase in viscosity observed during heating of starch in water was mainly attributing to the swollen granules and also to the amount of solubilized carbohydrates with reference to amylase.[Citation47] However, further continuous heating and shearing at a high temperature (95°C) promotes the weakening and susceptibility of the starch granules to shear damage (disruption).[Citation48] In fact, the difference in branch chain length distribution of amylopectin, crystallinity, granular size distribution, and presence of other components likely play an important role in differences in pasting properties among starches. The association between amylose and amylopectin molecules in mixed starches was different from that of individual starches, which induced specific chain interactions (between molecules, starch granules, swollen granules, and granule fragments) during heating, and each starch gelatinized independently of the other, thus providing double-peak viscosities. However, this phenomenon was not observed in mixtures made with high amylose starch and waxy starch nor between non-waxy starch mixtures. It is plausible that the very long branch-chains of amylopectin mimic amylose to form helical complexes with lipids and intertwine with other branch chains to hold the integrity of starch granules during heating and shearing.[Citation49] Sasaki et al.[Citation50] investigate that lower amylase content is associated with higher peak viscosity. Reduced amylose content starch relates to greater swelling. Greater swelling reduces the quantity of free water and is associated with higher pasting viscosity.[Citation51]. Leloup et al.[Citation52] reported that a minimum amylose to amylopectin ratio of 0.43 was needed to maintain the gel network during heating in water. At lower amylose content, the structure of starch gel is easily disrupted by heating. Amylose suppresses swelling and maintains the integrity of swollen starch granules.[Citation6] Because starch swelling is mainly a property of amylopectin[Citation29] waxy starch swells rapidly and swollen granules degrade at lower temperature, indicating that waxy starch rapidly develops viscosity but cannot maintain the stability of paste viscosity. The increase in viscosity during cooling is induced by leaching out amylose rearranging and forming a thin amylose gel layer.[Citation38] This suggests that starch with lower amylose content decreased the amount of leached out amylose, suppressing viscosity during cooling. The breakdown (peak viscosity minus viscosity after holding at 95°C (HPV) is caused by the disintegration of gelatinized starch granule structure during continuous stirring and heating.[Citation43] The differences among individual rice starch samples and their blends in breakdown are related to differences in rigidity of swollen granules.[Citation53,Citation54,Citation55] Moreover, amylose has a marked influence on the breakdown viscosity, which is a measure of the susceptibility of cooked starch granules to disintegration.[Citation56]
Rheological Properties
At the earlier stages of heating, i.e., slightly before 60°C, the increase in G′ was relatively slow and the starch suspension was transformed into a “sol,” and the amylose molecules were dissolved from the swollen starch particles.[Citation57] At a certain temperature above 60°C (>To), G′ of all the heated starch suspensions increased rapidly (as the starch granules kept swelling) to a maximum G′, tan Ð (G″/ G′), G″ max shown in . The initial increase in G′ was attributed to the interplay of the following factors: the progressive swelling of starch granules that finally become a close-packed network[Citation52,Citation12]; the solubilized amylose that was released during the heating process, and the increase in gel volume. In this study, the temperature TG′max for native and blended starches varied from 63.7 to 75.4° C and from 65.6 to 74.1°C for blended starches, respectively (). The rheological properties of starch mixtures varied to a large extent with respect to starch sources (). Svegmark and Hermansson[Citation2] reported that, during the heating cycle, the difference in the G′, G″, and tan Ð should be due to the difference in starch granular structure. Moreover, at G′max and TG′max all starch concentration systems studied during our experiment exhibited G′ values >5000 Pa and tan Ð values <0.2, and these are parameters of starch gels.[Citation7] The gelatinized starch granules strengthened the gel network formed by amylose[Citation58,Citation59] it illustrates the results from selected representative profiles and compares the effects of different ratios in between individual native barley starch and their blends with wheat, corn and rice starches on changes in storage modulus, G′, loss modulus, G″, during heating-cooling cycles. The parameters recorded of all starch suspensions are given in . Moreover, these effects showed similar changing patterns as the temperature increased. It was observed that G′ increased to maxima between 63.7–75.4° C and from 65.6–74.1°C for native and blended respectively and the sol-to-gel transition was reinforced by strong interactions among the swollen starch particles[Citation57] when the leached amylose formed a gel network. Keetls and Van Vliet[Citation60] indicated the decrease in elasticity after reaching G′ max can be attributed to the melting of the remaining crystalline region in the granules (or disintegration of close-packed swollen granules) that result in the granules becoming softer. Furthermore, differences in amylose contents in individual native rice starches and their respective mixtures are probably the main reason for the observed differences in G′ and G″. For starch mixtures, Depending on the proportion of the native barley starch in the mixture, the G′ peak maxima for barley starch blends were generally between those of their individual starch components at both ratios.
Figure 3 Representative Rheological properties of native Barley, Wheat, Corn, and Rice starches and their blends (B = Barley, C = Corn, W = Wheat, R = Rice).
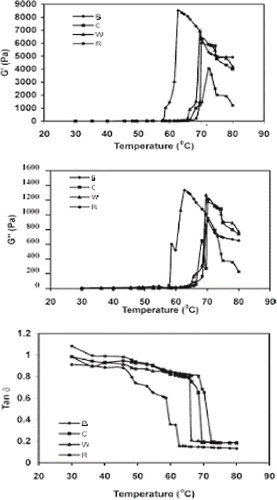
Table 4 Summary of rheological properties of barley starch and their blends (n = 3)
During cooling, the G' values of all native starch systems and their corresponding starch blends decrease during cooling. When heated starch pastes were cooled from 85 to 25°C, a quick development of gel formation took place due to intermolecular association in polymer-rich regions[Citation61,Citation8] and amylose aggregation. In addition, a general decrease in tan Ð was observed. Among individual native starches, barley starch exhibited relatively the highest G′, G″ values and lowest tan Ð, which proved the formation of the most rigid gel structure at both total starch concentrations. The blend made of barley: wheat, 75:25 ratio showed the highest G′ and G″ values. These results show that T G′ max, G″ and tan Ð are likely governed by amylose content. The rheogram that shows the influence of different ratios on change in loss modulus, G″ during cooling proved that the system was not stable and the phenomenon can be probably attributed to a lack of the rigidity and integrity of cooked granule. However, appreciable increases in storage modulii for the related blends of waxy starches occurred at both ratios (). Tan Ð varied significantly among individual native starches and their corresponding starch mixtures. This variation was ascribed to the difference in G′ and G″. Mixtures made of low, intermediate and high amylose content developed generally G' values between those of their individual components. A similar conclusion was drawn earlier in studies on starch mixtures from different botanical sources.[Citation62]
Retrogradation Properties
The retrogradation of gels prepared from native barley, wheat, corn, rice, and their blended starches at different ratios was measured by determining % syneresis during storage at 4°C. The % syneresis value of gelatinized starch gels from the native to blended starches differed significantly. For barley it was increased from 1.18–6.73%, for wheat, it was 2.21–6.54%, for corn, it was 7.10–8.65% and for rice, it was 1.93–8.43%. The same trend was followed when barley starch blended with others at different levels shown in Table. 2.
Native barley, wheat and rice starches showed lower values for % synersis than the native cornstarch that may be attributed to the rigid granular structure as shown in , and presence of lipids in starch[Citation63]. The differences in amylose content of barley, wheat, rice, and cornstarches might have also affected the retrogradation properties. Waxy starch has been reported to retrograde slowly but pea and potato starches with high amylose content.[Citation64,Citation65] Native corn and wheat starch gels showed higher % syneresis values than rice and barley (). As barley, starches blended with corn, wheat and rice at different levels the syneresis values of gels from that increased progressively during storage. Native starches from the corn and wheat starch having large sized granules showed higher % syneresis values while those barley and rice having small sized granules showed lower syneresis values (). The retrogradation properties of starches are indirectly influenced by the structural arrangement of starch chains with in the amorphous and crystalline regions of the ungelatinized granule which in turn, influence the extent of granule breakdown during gelatinization and the interactions that occurs between starch chains during gel storage.[Citation66] The rapid initial rate of retrogradation was related to the loss of networked amylose[Citation65] to the development of amylose aggregates, and the bonding of granule remnants into assemblies by amylose and amylose aggregates.[Citation30] It seems logical to mention that starch retrogradation is governed by a consecutive three-step mechanism that involves nucleation, propagation and maturation. For native starch and mixed starches lower paste clarity was explained by the presence of chain polymers that are resistant to retrogradation. In fact, the network of solubilized waxy starch was retained much of the amylose that leached out of the non-waxy starch, contributing to the lower paste clarity.[Citation67]
Gel Strength
In order to investigate the gel strength the starch gel was prepared with 14% starch suspension of native barley, wheat, corn and rice starches and their blends. The hardness values were measured at 24hrs, 72hrs and 120 hrs after storage at 4°C. The results are shown in . It can be observed that the hardness increased with the storage time and thus with the extent of starch retrogradation. The results showed that native rice starch exhibit the highest hardness (firmness) value followed by corn, barley, and wheat starch. As barley, starch blended with corn, rice, and wheat at different ratios the gel strength values increased during storage up to 120 hrs at 4°C, . Starch with high apparent amylose content gave pastes with high elasticity and greater rigidity. This observation was linked to a greater retrogradation tendency. Thus, the retrogradation rate and the degree of retrogradation orders were Corn > Rice > Barley > Wheat. Barley starch gelation was a much slower process, i.e., the amylopectin recrystallization was slower compared to that of amylose, which re-associates rapidly during cooling similar results were earlier described.[Citation68] Corn and rice starch gel showed highest compression force of 6.72N and 4.67N, respectively while barley and wheat starch gel showed the lower compression force of 3.9N and 3.72N. While in case of barley and wheat blended starches at different level compression force varies between 3.97–4.37N, barley and corn blended starches was varied between 3.92–4.92N and barley and rice blended starches was varied between 3.3–4.8N. The differences in compression force values of gels from different starches may be due to variation in their rheological and morphological properties. Hopkins and Gormley[Citation27] also reported higher compression force values for potato starches having high peak viscosity measured by Visco-amylograph and phosphorus content. The gel compression force values increased with storage duration. During storage, the starch molecules rearrange thus stiffness and rigidity of the granules increases.[Citation69]
CONCLUSION
It is possible to formulate starch blends from unmodified starches that possess at least some of the desired characteristics of modified starches. It appeared that starch molecules from different starches interact to produce the attributes of the blends and that at least some of the interactions occur very early in the heating process, before gelatinization and pasting occur. The amylose content of native barley, corn, wheat and rice starches varied widely. During thermal analysis using DSC, two characteristic endotherms peaks were observed in some cases at different temperature intervals. Measurement of G′ was used to study the development of the network structure of the gel. The rate of development of storage modulus, G′ and loss modulus G″, are concentration dependent. We conclude that TG′ max, G′ and tan Ð are certainly governed by amylose content. Amylose content suppresses starch swelling and appears to play a critical role in determining starch-pasting properties using RVA. Transmittance values of native and blended starch suspensions stored at 4°C generally decreased during storage up to day 6. The results indicated that the retrogradation tendency was the lowest in low amylose and highest in high amylose starch. Amylose content plays the most important role in changing the elasticity of the studied starchy systems after cooling up to 5°C, and during aging. Low amylose blends of 14% were most effective at reducing the rate of gel firmness as shown by the values of hardness when mixed with low and non-waxy starches. Starch mixtures could have a place in the food industry where all natural products are desirable and in the food and other industries, where reduction in the use of chemicals for modification is desirable.
REFERENCES
- Garci'a-Alonso , A. , Jime'nez-Escrig , A. , Mart'in-Carron , N. , Bravo , L. and Saura-Calixto , F. 1999 . Assesment of some parameters involved in the gelatinization and retrogradation of starch . Food Chemistry , 66 : 181 – 187 .
- Svegmark , K. and Hermansson , A. M. 1993 . Microstructure and rheological properties of composites of potato starch granules and amylose: A comparison of observed and predicted structure . Food Structure , 12 : 181 – 193 .
- Fleche , G. 1985 . “ Chemical modification and degradation of starch ” . In Starch Conversion Technology , Edited by: Van Beynum , G. and Roels , J.A. 73 – 100 . New York : Marcel Dekker, Inc .
- Galliard , T. and Bowler , P. 1987 . Starch: Properties and potential , Edited by: Galliard , T. and Morphology and composition of starch . 55 – 78 . Chichester : John Wiley and Sons .
- Liu , H. and Lelievre , J. A . 1992 . differential scanning colorimetery starch of melting transition in aqueous suspensions containing blends of wheat and rice starch . Carbohydrate Polymer , 17 : 145 – 149 .
- Hermansson , A.M. and Svegmark , K. 1996 . Developments in the understanding of starch functionality . Trends in Food Science and Technology , 7 : 345 – 353 .
- Lii , C.Y. , Shao , Y.Y. and Tseng , K.H. 1995 . Gelation mechanism and rheological properties of rice starch. Cereal Chemistry . 72 : 393 – 400 .
- Reddy , I. and Seib , P.A. 2000 . Modified waxy wheat starch compared to modified waxy corn starch . Journal of Cereal Science , 31 : 25 – 39 .
- Biliaderis , C.G. 1982 . Physical characteristics, enzymatic digestibility, and structure of chemically modified smooth pea and waxy maize starches . Journal of Agriculture and Food Chemistry , 30 : 925 – 930 .
- Cousidine , D.M. 1982 . Foods and Food Production Encyclopedia , 142 – 145 . New York : John Wiley Inc .
- Obanni , M. and Be Miller , J.N. 1997 . Properties of some starch blends. Cereal Chemistry , 74 : 431 – 436 .
- Lii , C.Y. , Tsai , M.L. and Tseng , K.H. 1996 . Effect of amylose content on the rheological property of rice starch . Cereal Chemistry , 73 : 415 – 420 .
- Liu , H. and Lelievre , J. 1992 . Differential scanning colorimetric and rheological study of the gelatinization of starch granules embedded in gel matrix . Cereal Chemistry , 69 : 597 – 599 .
- Chen , J.J. , Lai , V.M.F. and Lii , C.-Y. 2003 . Effect of compositional and granular properties on the pasting viscosity of rice starch blends . Starch/Starke , 55 : 203 – 212 .
- Del Rosario , R.P. and Pontiveros , C.R . 1983 . Retrogradation of some starch mixtures . Starch/Starke , 35 : 86 – 92 .
- Agboola , S. O. , Akingbala , J. O. and Oguntimein , G. B. 1991 . Physicochemical and functional properties of low DS cassava starch acetates and citrates . Starch/Starke , 43 : 62 – 66 .
- Moore , C.O. , Tuschhoff , J.V. , Hastings , C.W. and Schanefelt , R.V. 1984 . “ Application of starches in foods ” . In Starch: Chemistry and Technology , 2nd , Edited by: Whistler , R.L. , BeMiller , J.N. and Paschall , E.F. 579 – 592 . Orlando, FL : Academic Press .
- Evans , I.D. and Haisman , D.R. 1979 . Rheology of gelatinized starch suspensions . Journal Texture Studies , 10 : 347 – 370 .
- Kim , S.Y. , Wiesenborn , D.P. , Orr , P.H. and Grant , L.A. 1995 . Screening potato starch for novel properties using differential scanning calorimetry . Journal of Food Science , 60 : 1060 – 1065 .
- Wiesenborn , D.P. , Orr , P.H. , Casper , H.H. and Tacke , B.K. 1994 . Potato starch paste behaviour as related to some physical/chemical properties . Journal of Food Science , 59 : 644 – 648 .
- Vasanthan , T. and Bhatty , R.S. 1995 . Starch purification after pin milling and air classification of waxy, normal and high amylose barley . Cereal Chemistry , 72 ( 4 ) : 379 – 384 .
- Lee , M.R. , Swanson , B.G. and Baik , B.K. 2001 . Influence of amylose content on properties of wheat starch and bread making quality of starch and gluten blend . Cereal Chemistry , 78 ( 6 ) : 701 – 706 .
- Williams , P. C. , Kuzina , F. D. and Hlynka , I. 1970 . A rapid colorimetric procedure for estimating the amylose content of starches and flours . Cereal Chemistry , 47 : 411 – 420 .
- Leach , H.W. , McCowen , L.D. and Schoch , T.J. 1959 . Structure of the starch granule. I. Swelling and solubility patterns of various patterns of various starches . Cereal Chemistry , 36 : 534 – 544 .
- Craig , S. A. S. , Maningat , C.C. , Seib , P.A. and Hoseney , R.C. 1989 . Starch paste clarity of different botanical sources . Cereal Chemistry , 66 : 173 – 182 .
- Woo , Kyungsoo and Seib , Paul, A. 1997 . Cross-linking of wheat starch and hydroxypropylated wheat starch in alkaline slurry with sodium trimetaphosphate . Carbohydrate Polymer , 33 : 263 – 271 .
- Hopkins , S. and Gormley , R. 2000 . Rheological properties of pastes and gels made from starch separated from different potato cultivars . Lebens. Wissen.Technol. , 33 : 388 – 396 .
- Mauro , D. J. 1996 . An up to date of starch . Cereal Food World , 41 : 776 – 778 . 780
- Tester , R.F. and Morrison , W.R. 1990 . Swelling and gelatinization of cereal starches. I. Effects of amylopectin, amylase and lipids . Cereal Chemistry , 67 : 551 – 557 .
- Jacobson , M.R. , Obanni , M. and Bemiller , J.N. 1997 . Retrogradation of starches from different botanical sources . Cereal Chemistry , 74 : 571 – 578 .
- Miles , M.J. , Morris , V.J. , Orford , P.D. and Ring , S.G . 1985 . The roles of amylose and amylopectin in the gelatinization and retrogradation of starch . Carbohydrate Research , 135 : 271 – 281 .
- Badenhuizen , N.P. 1969 . The biogenesis of starch granules in higher plants , New York : Appleton Crofts .
- Li , J.H. , Vasanthan , T. , Rossnalgel , B. and Hoover , R. 2001 . Starch from hull-less barley: II. Thermal, rheological and acid hydrolysis characteristics . Food Chemistry , 74 : 407 – 415 .
- Fannon , J.E. , Hauber , R.J. and BeMiller , J.N. 1992 . Surface pores of starch granules . Cereal Chemistry , 69 : 284 – 288 .
- Singh , J. and Singh , N. 2001 . Studies on the morphological, thermal and rheological properties of starch separated from some Indian potato cultivars . Food Chemistry , 75 : 67 – 77 .
- Gudmundsson , M. and Elliasson , A. C. 1992 . Some physical properties of barley starches from cultivars differing in amylose content . Journal of Cereal Science , : 95 – 105 .
- Cooke , D. and Gidley , M. .J. 1992 . Loss of crystalline and molecular order during starch gelatinization: Origin of the enthalpic transition . Carbohydrate Polymer , 227 : 103 – 112 .
- Flipse , E. , Keetels , C.J.A.M. , Jacobson , E. and Visser , R.G.F. 1996 . Absence of amylose has a distinct influence on the physicochemical properties of starch . Theoretical Applied Genetics , 92 : 121 – 127 .
- Krueger , B.K. , Walker , C.E. , Knutson , C.A. and Inglett , G.E . 1987 . Differential scanning colorimetery of raw and annealing starch isolated from normal and mutant maize genotypes . Cereal Chemistry , 64 : 187 – 190 .
- Fredriksson , H. , Silverio , J. , Andersson , R. , Eliasson , A. C. and Aman , P. 1998 . The influence of amylose and amylopectin characteristics on gelatinization and retrogradation properties of different starches . Carbohydrate Polymer , 35 : 119 – 134 .
- Yuan , R. C. , Thompson , D.B. and Boyer , C. D. 1993 . Fine structure of amylopectin in relation to gelatinization and retrogradation behaviour of maize starches from three wx-containing genotype in two inbred lines . Cereal Chemistry , 70 : 81 – 89 .
- Shibanuma , Y. , Tekeda , Y. and Hizukuri , S. 1996 . Molecular and pasting properties of some wheat starches . Carbohydrate Polymer , 29 : 253 – 261 .
- Whistler , R.L. and BeMiller , J.N . 1997 . Carbohydrate Chemistry for Food Scientist , St. Paul, MN : American Association of Cereal Chemists .
- Fortuna , T. , Januszewska , R. , Juszczak , I. , Kielski , A. and Palasinski , M. 2000 . International Journal of Food Science and Technology . 35 : 285 – 291 .
- Batey , I.L. and Curtin , B.M . 2000 . Effects on pasting viscosity of starch and flour from different operating conditions for the Rapid Visco Analyser . Cereal Chemistry , 77 : 754 – 760 .
- Jane , J. and Chen , J.F. 1992 . Effect of amylose molecular size and amylopectin branch chain length on pasting properties of starch . Cereal Chemistry , 69 : 60 – 65 .
- Yeh , A.I. and Li , J.Y. 1996 . A continuous measurement of swelling of rice starch during heating . Journal of Cereal Science , 2 : 227 – 283 .
- Thiewes , H.J. and Steeneken , P.A.M. 1997 . Comparison of the Brabender viscograph and the Rapid Visco Analyser. I. statistical evaluation of the pasting profile . Starch/Starke , 49 : 85 – 92 .
- Jane , J. , Chen , J.J. , Lee , L.F. , McPherson , A.E. , Wong , K.S. , Radosavljevie , M. and Kasemsuwan , T. 1999 . Effect of amylopectin branch chain length and amylase content on the gelatinization and pasting properties of starch . Cereal Chemistry , 76 ( 5 ) : 629 – 637 .
- Sasaki , T. and Yasui , T. 2000 . Matsuki, Effect of amylose content on gelatinization, retrogradation and pasting properties of starches from waxy and non-waxy wheat and their F1 seeds . J. Cereal Chemistry , 77 ( 1 ) : 58 – 63 .
- Ming , Z. , Morris , C.F. , Batey , I.L. and Wrigley , C.W. 1997 . Sources of variation for starch gelatinization, pasting, and gelation properties in wheat . Cereal Chemistry , 74 : 63 – 71 .
- Leloup , V.M. , Colonna , P. and Buleon , A. 1991 . Influence of amylose-amylopectin ratio on gel properties . Journal of Cereal Science , 13 : 1 – 13 .
- Sandhya Rani , M.R. and Bhattacharya , K.R. 1995 . Rheology of rice flour paste: Effect of variety, concentration, and temperature and time of cooking . Journal of Texture Studies , 20 : 127 – 137 .
- Sandhya Rani , M.R. and Bhattacharya , K.R. 1995 . Rheology of rice flour paste: Relationship of paste breakdown to rice quality, and simplified Brabender viscograph test . Journal of Texture Studies , 26 : 587 – 598 .
- Karim , A.A. , Norziah , M.H. and Seow , C.C. 2000 . Methods for the study of starch retrogradation . Food Chemistry , 71 : 9 – 36 .
- Lee , N.H. , Hettiarachchy , N.S. and Mcnew , R.W. 1995 . Gnanasambandam. R. Physicochemical properties of calcium-fortified rice . Cereal Chemistry , 72 : 352 – 355 .
- Hsu , S. , Lu , S. and Huang , C. 2000 . Viscoelastic changes of rice starch suspensions during gelatinization . Journal of Food Science , 65 : 215 – 220 .
- Eliasson , A.C. 1986 . Viscoelastic behavior during the gelatinization of starch I. Comparison of wheat, maize, potato and waxy-barley starches . Journal of Texture Studies , 17 : 253 – 265 .
- Ring , S. G. 1985 . Some studies on starch gelation . Starch/Starke , 37 : 80 – 83 .
- Keetels , C.J.A.M. , Vliet , T.V. and Walstra , P. 1996 . Food Hydrocolloid , 10 : 343 – 353 .
- Biliaderis , C. G. and Juliano , B.O. 1993 . Thermal and mechenical properties of concentrated rice starch gels of varying composition . Food Chemistry , 48 : 243 – 250 .
- Ortega-Ojeda , F.E. and Eliasson , A.C. 2001 . Gelatinization and retrogradation behavior of some stach mixtures . Starch/Starke , 53 ( 10 ) : 520 – 529 .
- Singh , J. , Singh , N. and Saxena , S.K. 2002 . Effect of fatty acids on the rheological properties of corn and potato starch . Journal of Food Engineering , 52 : 9 – 16 .
- Teo , C.H. and Seow , C.C. 1992 . A pulsed NMR method for the study of starch retrogradation . Starch/Starke , 44 : 288 – 292 .
- Chang , S.M. and Liu , L.C. 1991 . Retrogradation of rice starches studied by differential scanning calorimetery and influence of sugars, NaCl and lipids . Journal of Food Science , 56 : 564 – 566 .
- Perera , C. and Hoover , R. 1999 . Influence of hydroxypropylation on retrogradation properties of native, defatted and heat– moisture treated potato starches . Food Chemistry , 64 : 361 – 375 .
- Hagenimana , A. and Ding , X. 2005 . A comparative study on pasting and hydration properties of native rice starches and their mixtures . Cereal Chemistry , 82 ( 1 ) : 70 – 76 .
- Russell , P.L. and Berry , C.S.P. 1989 . Characterisation of resistant starch from wheat and maize . Journal of Cereal Science , 9 : 1 – 15 .
- Keetls , C.J.A.M. 1994 . “ Van Vliet. Gelation and retrogradation of concentrated starch gels ” . In Gums and stabilizers for food industry , Edited by: Phillips , G.O. , Williams , P.A. and Wedlock , D.J. 271 – 280 . New York : IRL .