Abstract
Addition of glucose oxidase (GOX), peroxidase (POX), and xylanase (XYL) on dough rheological parameters, and bread quality was studied. When these enzymes were used as a blend, it showed a different effect compared to when added alone. Addition of peroxidase or xylanase increased water absorption, while incorporation of GOX did not affect it. Water absorption decreased in flour added with a blend of the three enzymes. High concentrations of GOX or XYL decreased stability, while presence of POX had minimum effect on this parameter. None of the enzymes, neither alone or blended, improved bread volume. Crumb characteristics were only improved by XYL.
INTRODUCTION
Adding chemical oxidants to wheat flour is a common practice to obtain stronger flours, which produce bread of larger volume. In several developing countries, the production of high protein wheat varieties is not sufficient, so millers need to use blends of low and high protein varieties as a method to obtain enough flour to sustain the production of bread. These flours are usually added with oxidants (chemical or biological) to achieve acceptable bread quality. Potassium bromate was heavily used to this purpose until it was banned because of public health concerns. Initially it was substituted with other chemical additives such as azodicarbonamide or ascorbic acid. Nowadays, other alternatives are being used, such as exogenous enzymes, which are regularly added either during the milling process by the miller, during the dough preparation by the baker or even during both processes.
Oxidative or hydrolytic enzymes such as glucose oxidase (GOX), peroxidase (POX), and xylanase (XYL) have been proposed as dough improvers. GOX has been claimed to promote the development of a less extensible and most resistant dough when added to wheat flour,[Citation1,Citation2,Citation3] while the addition of either POX or XYL has been reported to improve dough handling, dough tolerance, crumb structure and loaf volume.[Citation4] The simultaneous addition of more than one enzyme to wheat flour is becoming a common practice, like the use of commercial blends of fungal amylases and proteases; however, the majority of the reported studies regarding the effects of enzymes on rheological properties or bread characteristics has been done with just one kind of enzyme.[Citation1,Citation5,Citation6,Citation7,Citation8,Citation9,Citation10,Citation11,Citation12] Only some works describe the simultaneous effect of two enzymes on dough rheology or bread characteristics. Hilhorst et al.[Citation4] analysed the effect of adding a fixed concentration of xylanase blended with a constant concentration of either glucose oxidase or peroxidase, and reported that the dough containing xylanase plus peroxidase showed the best performance. Primo-Martin et al.[Citation2] studied the effect of three single enzymes: pentosanase (PP), glucose oxidase (GOX), and laccase (LAC), and their combinations, on rheological characteristics of dough and glutenin macropolymer (GMP). They also used fixed concentrations of each enzyme and observed some synergic effects between PP and GOX on gluten properties. Since the addition of glucose oxidase, peroxidase or xylanase has been reported to be positive on dough and bread quality, the simultaneous use of them could produce a complementary beneficial effect. The consequence of the addition of these three enzymes can be verified using dough rheological measurements, mainly alveographic, farinographic, or extensographic ones, as well as using breadmaking tests, in which loaf volume and bread attributes, such as colour, texture and cellular structure (also named grain structure), are evaluated. Farinograms and extensograms can give complementary information about how the enzymes change the dough development, which is reflected in bread quality.
Traditionally, bread-crumb analysis has been conducted visually by scoring the product based on baker's experience, who considers the grain compactness (open or close), the grain uniformity (regular or irregular) as well as the cell shape (round, oval or elongated) and the thickness of the cell wall (thin or thick).[Citation13] Nowadays these parameters can be evaluated by means of advanced instrumental and computational techniques such as X-ray, Ultrasound, Magnetic Resonance Imaging or Digital Image Analysis (DIA). The latter is the less expensive tool to evaluate the form, the structure and the colour of food, including bread crumb.[Citation14,Citation15,Citation16] Digital Image Analysis has already been used in different breadmaking studies. Moore et al.[Citation17] evaluated the effect of different concentrations of transglutaminase on the quality of gluten-free bread, employing DIA to characterise the bread crumb structure. Collar, Bollaín & Angioloni[Citation18] evaluated the impact of microbial transglutaminase on crumb grain pattern of fresh pan breads, by DIA. Other studies have been carried out using DIA to evaluate crumb characteristics, but they were not related to the effect of enzymes.[Citation19, Citation20, Citation21, Citation22] In this work, the effect of adding GOX, POX, and XYL blends, at different concentrations, on wheat flour rheological properties and on bread quality were studied.
MATERIALS AND METHODS
Ingredients
A low-protein commercial wheat flour without additives (9.4% protein (N × 5.7), 0.56% ash, 55.8% farinographic water absorption), which usually requires additives incorporation, was used. All others ingredients: table salt, sugar, skim milk powder, dry instant yeast and vegetable fat were commercial grade. Glucose oxidase (GOX, 50000 U) from Aspergillus niger, horseradish peroxidase type VII (POX, 5000 U) and xylanase (XYL, 1000 U) from Trichoderma viride were all purchased from Sigma Chemical Co. (St. Louis, MO). Commercial xylanase from T. viride is reported to exhibit a higher activity towards water unextractable pentosans (WUP) than that towards water extractable pentosans (WEP).[Citation23] According to the 1996 Sigma Catalogue the enzyme units were defined as follows: A glucose oxidase unit is defined as the quantity of enzyme that will oxidise 1.0 μmol of β-D-glucose to D-gluconolactone and H2O2 per min, at pH 5.1 and 35ºC. A xylanase unit is defined as the quantity of xylanase that will liberate 1 μmol of reducing sugar measured as xylose equivalents, from xylan taken from oat spelts, per minute at pH 4.5 and 30ºC. A peroxidase unit is defined as the quantity of enzyme that will form 1.0 mg (2.37 μmol) of purpurogallin from pyrogallol in 20 s at pH 6.0 and 20ºC.
Flour Preparation
Enzymes were mixed thoroughly with portions of wheat flour using a K45SS Kitchen Aid mixer (Kitchenaid INC, St. Joseph Michigan, USA), to get three stock samples, each of which containing 250 units of GOX, 5 units or POX or 1 units of XYL in 1 g of flour. Calculated amounts of these mixtures were added to the flour to give the desired test enzyme concentrations used during the experiments.
Farinographic Measurements
A farinogram for each formulation was performed in a 300 g Brabender Farinograph (Brabender OHG, Duisburg, Germany) following the procedure recommended by the manufacturer. Selected responses were: water absorption (WA), maximum consistency time (MCT) and stability (STA). MCT is defined as the time when the farinographic curve reaches a maximum and starts to fall, and the dough softening stage begins.[Citation24] MCT can be regarded as a more direct measurement of dough development, since it is easier to visualise in the farinogram and has no ambiguity as in the case of the farinographic “false peak time”. Furthermore, Oliver and Allen[Citation25] published that the optimum bread volume is obtained at the end of the farinogram plateau, which is the point where MCT is measured.
Extensographic Measurements
Extensographic parameters were measured in a Brabender Extensograph (Brabender OHG, Duisburg, Germany) according to the manufacturer's procedure. The parameters measured included: maximum resistance to the extension (Tmax,) and extensibility (L). Tmax is reported in Brabender units (BU), while L is reported in mm. Both extensographic measurements were taken at 45, 90, or 135 min of dough resting as required by the experimental design described below.
Baking Procedure
The optimised straight-dough breadmaking method,[Citation26] at the water level of farinographic absorption, was used to evaluate the effect of different enzyme concentrations on the loaf volume and crumb cell structure. Some modifications were made to the reported method, such as using dry instant yeast that was equivalent in amount to that of the original method (2.5 g compressed yeast), and without adding any kind of additives.
All ingredients, except water, were mixed for 2 min, and then water (30ºC) was poured in the mix. Dough was mixed in a K45SS Kitchen Aid mixer (Kitchenaid INC, St. Joseph Michigan, USA) until the optimum consistency was reached as evaluated by baker's experience. After mixing, the dough was weighed (150 g), rounded by hand and placed into a fermentation chamber for resting. The fermentation process was divided into three fermentation steps and one proving step. After each fermentation stage, the dough was punched and rounded to redistribute the nutrients used by the yeast and to make its temperature more uniform; it was then returned to the fermentation cabinet. After the third stage, the dough was punched, rounded, moulded, placed into the baking pan and left to proof. Fermentation was done in an adapted fermentation cabinet (Afos, Limited, Hull, UK) which provided constant process conditions of 30 ± 2ºC and 75 ± 5% relative humidity. Bread was baked at 210ºC for 20 minutes in a rotary oven (Henry Simon Limited, Cheshire UK). Loaf weight was measured immediately after baking; the loaf volume was determined 120 minutes after that. Three loaves were obtained from each dough sample. Each dough was a single test in the experimental design.
Bread Quality
Volume was determined using the rapeseed displacement method. Crumb characteristics were evaluated by image analysis of crumb structure as described below.
Image Analysis
Three loaves per experiment were used for crumb quality measurements. Two central slices of 20 mm thick were cut transversely from each loaf, using an electric bread knife. A single rectangular field of view (FOV) of 5.2 cm × 4.0 cm that represented approximately one-third of its total sectional area was evaluated at the centre of each slice. Considering that ambient illumination is critical for reproducible imaging, the image acquisition was performed inside a dark room.[Citation27] Images were acquired based on the method described by Bertrand et al.[Citation28] with some modifications: slices were placed before a black background, illuminated by two 75 Watt daylight lamps placed at both sides of the samples, 16.5 cm over the working plane and at an angle of 45° each. A colour video camera (Sony, Digital 8 DRC-TRV-120, Japan) was located vertically above the bread slices at a distance of 5 cm, so the angle between the video axis and lighting source was 45°. The camera settings were: lens aperture at f = 3.7, obturation speed 1/30, zoom in the position for angular wide, exposure in +9, no flash nor automatic focus. The video camera was connected to the i-link interface of a generic PC (AMD Duron 647 MHZ. 128 MB RAM, 80 GB hard disk), equipped with a video card (ATI, All in Wonder Rage-128Pro, USA) and using software for image acquisition (ATI version 6.2, Multimedia Center, USA). The images were stored in a bit map (bmp) colour and graphics format of 24 bits, with a resolution of 640 × 480 pixels and prior to analysis they were converted to a 256 gray scale (0–255) in 8 bit format by means of a SigmaScan Pro Image Analysis 5.0 software (Jandel Scientific Inc. USA). The segmentation process was performed manually by the threshold tool of the SigmaScan software, according to Crowley et al.[Citation22] Three characteristics of the crumb were evaluated: the number of cells per square centimeter (pores/cm2), the overall mean cell area (mm2) and the average cell shape-factor (S). Cell shape-factor estimates the circularity of pores. A perfect circle has a shape factor of 1.0, while a line has a shape-factor approaching zero.[Citation29] The count of cells and the others two parameters were extracted to an electronic spreadsheet by the software. The final data were processed in Microsoft Excel XP.
Experimental Procedure
Three experiments were carried out: one to analyse the effect of the addition of single enzymes at different concentrations on flour farinographic and extensographic properties and bread volume; and the other two to evaluate the effect of adding enzyme blends on farinographic and extensographic parameters, bread volume and crumb quality.
Enzyme Concentrations Tested
The following activity concentrations were selected for the single enzyme experiment: GOX 0, 25 and 50 units; XYL 0, 15 and 30 units, and POX 0, 1.5 and 3.0 units, all per 100 g of flour. These enzyme concentrations were proposed based on previous experiments (data not shown) and literature references. For the blended enzyme experiments, the GOX concentration was limited to 25 units (U) per 100 g of flour, according to the results of the single enzyme experiment. The concentrations of the enzymes used were GOX: 0, 12.5, 25 U; XYL: 0, 15, 30 U; POX: 0, 1.5, 3.0 U, all per 100 g of flour.
Experimental Designs
Two experimental designs were used for the blended enzymes tests. The experimental design for farinographic evaluation and for bread quality was a two-level factorial design with four central point repetitions, and three factors were analysed. A total of twelve samples were run as shown in . The experimental design applied on extensographic characteristics was also a two-level factorial design with four central point repetitions, but four factors were analysed. In this case, a total of twenty experiments were run. Factor and levels used in this experiment are shown in . Selected responses were common rheological (farinographic and extensographic) parameters, and bread quality was measured through volume and cell crumb characteristics. These responses were expressed individually as functions of the independent variables.
Table 1 Response surface experimental design
Table 2 Response surface experimental design for extensigraphic parameters
Data were treated by multiple regression analysis; ANOVA, effect of each variable and regression analysis were reported in order to adjust the variables to a hierarchical mathematical model. The experimental sequences, the data analysis and the response surface plots were obtained from the Design Expert Program software, version 6.0 (Stat-Ease Inc., Minneapolis, USA). The experiments of the designs were all done by duplicates to assure that the data included in the experimental design sheet was representative of the conditions tested. The single enzyme experiment was performed by triplicate.
RESULTS AND DISCUSSION
Effect of Single Enzymes on Farinographic and Extensographic Parameters
shows GOX, POX, and XYL addition effects on the farinographic and extensographic parameters of wheat flour, as well as on bread volume. These tests were carried out using not blended enzymes. Addition of either XYL or POX increased water absorption as compared to the control. Both enzymes reached a value of 57.3%, while GOX did not affect it. The three enzymes induced an increase in the maximum consistency time. GOX had the longest effect (9.2 min) when 50 units were added. Stability varied from 17.7 min (control sample) to 20.1 min (3.0 units POX). XYL did not affect stability in the same way the other enzymes did. When XYL was used at 15 units, stability fell to a minimum (13.4 min), and then increased (17.4 min) when XYL was raised to 30 units.
Table 3 Effect of single enzyme concentration on farinographic and extensographic propertiesFootnote a
All three enzymes increased dough maximum resistance to the extension, proportionally to the concentration used. GOX produced the highest effect at both concentrations, while POX, when added at its lowest concentration, resulted in a slight increment on Tmax as compared to the control. None of the enzymes had a significant effect on extensibility (p 0.05). Bread volume was affected negatively by increasing the level of addition of any of the enzymes as compared to the control (580 cm3), having a minimum response (466 cm3) when flour was added with 50 unit of glucose oxidase. POX at 1.5 units was the only exception to this negative effect since its addition enlarged bread volume to its highest value (595 cm3). Similar results were reported by Indrani et al.,[Citation1] mainly in terms of farinographic characteristics. A noticeable negative effect on bread volume was induced when GOX was used at 50 units. Bread volume is a very important quality parameter, so it was decided for the next set of experiments to limit the maximum level of addition of GOX to 25 units. The concentrations of XYL and POX used were considered appropriate towards breadmaking when using low protein flours, in spite of the slight decrease they caused in bread volume, due to the increase in water absorption and stability which are particularly important in the mixing stage.
Effect of Blended Enzymes on Farinographic Parameters
Water absorption
The combined effect of XYL, POX, and GOX addition on WA is shown in . It can be seen that GOX presence decreases WA values within the range of the tested GOX concentrations, which are shown as a progressive flattening in the response surfaces of and compared to . When enzymes were used as a mixture at their highest concentrations, water absorption decreased almost to the value of the control sample, hindering the positive individual effects of XYL and POX observed in the previous single enzyme experimental results.
Figure 1 Effect of GOX, POX and XYL mixtures on farinographic water absorption. WA: water absorption (%); XYL: xylanase units; POX: peroxidase units; GOX: glucose oxidase units. A) Constant 0.0 units of glucose oxidase. B) Constant 12.5 units of glucose oxidase. C) Constant 25 units of glucose oxidase.
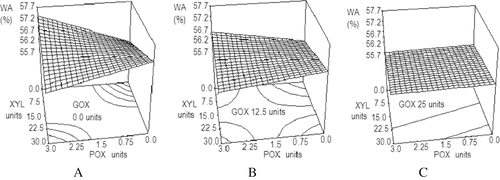
Farinographic water absorption was related to the type of enzyme used and its concentration (P < 0.0001). Statistical analysis showed that data could be fitted to the model in Eq. (1) of , with a determination coefficient R2 of 0.999 and a variation coefficient of 0.02%. No reports have been found on effects of blended enzymes on WA, while effects of adding single enzymes to flour are scarce. Indrani et al.[Citation1] reported that this parameter changes very little with the addition of either XYL or GOX without specifying WA values.
Table 4 Response surface mathematical models of evaluated parameters
Changes in dough consistency may affect the farinographic water absorption measurements. If during mixing dough consistency is larger than the target value of 500 BU, more water is needed to lower consistency to the desired value. Consequently water absorption is increased if consistency is increased too. Petit-Benvegnen et al.[Citation30] reported that the specific viscosity of a supernatant containing soluble arabinoxylans presented an initial increment, and then passed through a maximum to finally decrease as xylanase level was risen, thus modifying dough consistency.
Maximum consistency time
shows the effect of the three enzymes on MCT. As in the case of WA, MCT increased when enzymes were added alone and decreased when they were added as a blend. MCT varied from 1.5 to 8.3 min depending on the added enzymes and the amount used. Successive increments of GOX concentration in the absence of the other enzymes produced small changes on MCT (from 6.8 to 8.3 minutes). When no GOX was added, MCT was almost unaffected by POX or XYL changes (A). Results reported by Indrani et al.[Citation1] indicated that the sole addition of GOX or XYL, increased the dough development time, which agrees with our results.
Figure 2 Effect of GOX, POX, and XYL mixtures on farinographic maximum consistency time. MCT: maximum consistency time (min); XYL: xylanase units; POX: peroxidase units; GOX: glucose oxidase units. A) 0.0 units of glucose oxidase. B) 12.5 units of glucose oxidase. C) 25 units of glucose oxidase.
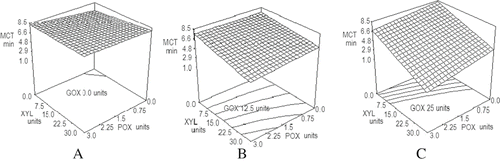
When GOX was increased to 12.5 units, the addition of 30 units of XYL produced a decrease of about one third on MCT, as compared to the response obtained with no XYL and at high POX (3.0 units). However, in the absence of POX, the decrement of MCT was around 10%, as XYL was being increased (). When GOX concentration was 25units, the decrement of MCT caused by the increase of XYL from 0 to 30 units was close to 80% at high POX and 30% in the absence of POX ().
The results shown in may be explained on the basis that XYL action prevents the interference of pentosans with gluten formation, by dissolving WUP to WEP.[Citation31] Moreover, as POX mediates oxidative gelation of WEP using native H2O2,[Citation32] an increase of dough viscosity occurs, thus increasing MCT. Results by Labbat et al.[Citation33] and Michniewicz et.[Citation34] indicate that the presence of WEP rises dough development time. This last parameter is proportional to MCT. Xylanase and glucose oxidase alone and the interaction between both enzymes had the largest effect (P < 0.0001) on MCT, while the effect of POX on the same parameter was smaller (P < 0.0039) but significant. Data were fitted to the model of Eq. (2) in , with a R2 of 0.999 and a variation coefficient of 1.8%.
Dough stability
shows the effect of the three enzymes on STA. It is possible to observe that progressive addition of GOX ( A to C) resulted in a decrease in stability (P = 0.0194) while xylanase, when added alone, raised STA values (P = 0.0296). Peroxidase, on the other hand, did not cause effects on this parameter (P = 0.905), while the interaction between xylanase and peroxidase was significant (P = 0.0460) towards the decrease of STA. This effect was more evident as the concentration of glucose oxidase increased. The response surface model describing the effect of the added enzymes on farinographic stability is presented in Eq. (3) of . The model had a determination coefficient R2 of 0.954 and a variation coefficient of 8.7%. No studies on the addition of more than one enzyme on dough STA were found.
Figure 3 Effect of GOX, POX, and XYL mixtures on farinographic dough stability. STA: dough stability (min); XYL: xylanase units; POX: peroxidase units; GOX: glucose oxidase units. A) 0.0 units of glucose oxidase. B) 12.5 units of glucose oxidase. C) 25 units of glucose oxidase.
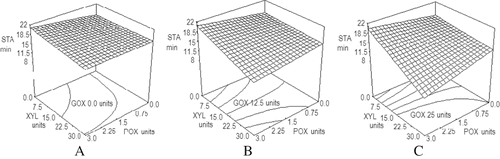
Indrani et al.[Citation1] reported an increase of dough stability with the addition of XYL, a tendency similar to that found in our work. Prabhasankar et al.[Citation12] reported a decrease in STA that we only found when GOX was added along with XYL, both at the highest concentrations used. Regarding the GOX effects on STA, Indrani et al.[Citation1] and Prabhasankar et al.[Citation12] reported an increment on dough stability when a single GOX concentration was added, while we found a slight decrease with increasing concentrations of GOX. This discrepancy may be attributed to the difference on the concentrations of GOX used in those experiments which were lower than the range tested in this work.
Effect of Blended Enzymes and Resting Time on Extensographic Parameters
Maximum resistance to the extension
shows the results of the addition of different concentrations of GOX, POX, and XYL, and resting time on Tmax. This parameter varied from 340 BU to 576 BU. The lowest values were obtained either when adding blends of GOX/XYL (C) or POX/XYL (A), both at their higher concentrations and at the shortest (45 min) resting time. The largest Tmax value was obtained when GOX was not used blended at its largest concentration and dough was rested for 135 minutes (I). Poulsen and H⊘strup,[Citation35] while evaluating the effect of GOX, reported similar values for Tmax, as resting time varied from 45min to 135 min. Indrani et al.[Citation1] and Prabhasankar et al.[Citation12] reported a small increase in Tmax with XYL and a notable increase when GOX was not added blended. Rosell et al.[Citation3] also reported that the addition of glucose oxidase resulted in a dough that was more resistant to extension than their control sample. All the blends tested caused a decrease in this parameter as compared to the control, while a slightly increment was also observed due to resting time. Statistical analysis of Tmax data exhibited that all enzymes and their interactions affected the response (P < 0.05) and so data could be fitted to the model of Eq. (4) of , with a determination coefficient R2 of 0.978 and a variation coefficient of 3.1%.
Extensibility
Extensibility results varied from a minimum of 146 mm to a maximum of 175 mm throughout the range of enzyme concentrations tested and were only affected by GOX and resting time, with probability values of P = 0.025 and P = 0.0127 respectively. An increase in GOX concentration from 0 to 25 units decreased average extensibility values from 158 mm to 151 mm. On the other hand, an increment of resting time from 45 minutes to 135 minutes decreased the average extensibility results from 159 mm to 150 mm. Poulsen and Hostrup[Citation35] reported that extensibility decreased with longer resting times at any level of GOX addition, which is similar to the results reported in this work. They also showed that the addition of increasing levels of GOX did not have a lineal increasing response depending on the concentration used. With respect to XYL, Primo-Martin et al.[Citation36] reported that adding pentosanases to flour did not have significant effect on the extensibility at break, as compared to their control, which is a similar result to the information obtained in this work. Data could not be fitted to a model with an acceptable R2 value so no equation or graph is presented for extensibility.
The rheological results found in this work may be explicable considering that the three added enzymes have H2O2 as a common link. Hydrogen peroxide is produced by glucose oxidase, but it is also the substrate of peroxidase and accessory for xylanase-mediated reactions, like the oxidative gelation. H2O2 has been reported to promote different reactions in a wheat dough, like disulfide bonding between the water soluble protein fraction,[Citation37] dityrosine bonding in gluten protein,[Citation38,Citation39] oxidative gelation of wheat WEP and other enzymatic reactions that could have an effect in both farinographic and extensographic properties. If dityrosine bonds are formed in the repetitive domain of the high molecular weight glutenin subunits (HMWG),[Citation40] they may produce an increase in the resistance to the extension. At the same time they impede the slip between the alpha helical terminal domains of HMWG, so a large change in extensibility is also avoided. Depending on the type and concentration of enzymes added to the formulation, the H2O2 may be used in different pathways, provoking different linkages among wheat flour components and resulting in different effects as compared to those caused when enzymes are added alone. This explanation remains to be tested.
Effect of Different Enzyme Concentrations on Product Quality
Bread quality was evaluated by measuring loaf specific volume and crumb structure. This last bread characteristic was analyzed through DIA, measuring the number of pores per square centimeter, the mean cell area, and the mean shape factor.
Bread volume
shows the effect of adding mixtures of enzymes at different concentrations on bread volume. A decrease in volume was observed when the enzymes were not supplemented as a blend at their maximum concentrations, varying from 580 cm3 for the control sample to 550 cm3 for any of the three enzymes alone. When GOX was not added (), using POX and XYL in increasing concentrations, bread volume was raised, but this only resulted in a similar value to the control sample. On the other hand, when 25 units of GOX were added with no XYL in the blend, the increase in POX concentration slightly improved bread volume (). None of the enzymes improved bread volume over the non-added formulation, with the exception of the blend with high levels of GOX and XYL without POX, which produced a slight volume increase over the control. Published information about this parameter is contradictory. Some authors described a volume improvement due to GOX or XYL [Citation1,Citation18,Citation38] while others reported that there was not a significant change in bread volume due to these enzymes[6,9], which are more alike results to those we obtained. A good breadmaking performance depends on gluten rheological properties. If the enzymes cause the dough to increase its resistance to the extension without improving its extensibility, as shown in , then a poor volume expansion during proving and baking is obtained. The extensographic results observed in the previous experiments are congruent with these bread volume results. Statistical analysis of volume data showed that xylanase, the interaction of xylanase and glucose oxidase and the three enzymes interactions affected the response (P < 0.05). Data could be fitted to the model of Eq. (5) of , with a determination coefficient R2 of 0.968 and a variation coefficient of 0.83%.
Number of pores per square centimeter
The control formulation had 37.6 cell/cm2. Crowley et al.[Citation22] reported that standard bread has 39.7 cells/cm2, while Collar et al.[Citation18] reported a standard value of 39 cells/cm2. Both reported values are similar to our control. The effect of enzymes addition on the number of pores/cm2 varied from 24.8 for maximum XYL-POX-GOX concentrations to 43.0, which corresponded to the maximum levels of addition of XYL and GOX. (). Statistical information showed that only XYL, when added alone, had a significant effect on the response (P < 0.0438), reducing the number of cells as XYL concentration increased (). The statistical analysis for the ternary blend, at its maximum concentration, and the binary blend GOX-POX showed probability values smaller than 0.0821 and 0.0671, respectively, but not smaller than 0.05, which mean a moderate level of significance for these variables. All the others formulations presented much larger probability values. In spite of this, the model in Eq. (6) of had a determination coefficient of 0.909 and a variation coefficient of 9.2%, meaning that the equation has a good fitting to the data. According to Cauvian et al.[Citation41] the number of bubbles in the dough depends on the kind of mixer and on process mixing conditions. The differences in the number of pores caused fundamentally by xylanase imply an effect that is not attributable to the mixer configuration because all the experiments were performed with a single geometry and the same process conditions. When dough is fermented, H2O2 can be produced[Citation32] and if endogenous peroxidase acts on this substrate, the XYL mediated oxidative gelation increases dough viscosity.[Citation6,Citation31] At the same time, during fermentation, resistance to extension increases. These two factors (viscosity and resistance to extension) may avoid gas cell to grow or coalesce at the same extent than the control, resulting in bread with fewer pores.
Mean cell area
The overall mean cell area varied from 0.24 mm2 (XYL alone) to 0.68 mm2 (control). Collar et al.[Citation18] reported an overall mean cell area of 0.66 mm2, while Crowley et al.[Citation22] cited an overall mean cell area varying from 0.7 to 0.84 for their standards. These values are in agreement with our control sample. shows the effect of mean cell area when enzymes added and their concentrations were varied in the blend. The size of pores was almost unaffected by POX concentration changes, while XYL concentration increments caused a decrease on this parameter as its concentration increased. GOX addition had an antagonistic effect to the one caused by XYL, making the cells larger even at high XYL concentrations. This is evident in as a levelling of the response surface on the xylanase axis. In the photos of , the smaller crumb cells due to sole xylanase addition ( B) can be compared to the bigger crumb cells of the xylanase-glucose oxidase pictures ( D), which resemble the crumb of the non-added control sample (). and resemble each other, indicating a slight counteraction of POX higher concentration against the XYL effect on cell size. The formulation added only with XYL had the largest significant effect (P<0.0009) on this parameter. Collar et al.[Citation18] reported that in XYL supplemented breads, smaller cells and thicker cell walls were observed. The other two enzymes and their interactions did not have significant effects (P 0.05), although the blend XYL-GOX had a probability value of P<0.051 which locates this interaction on the limit of significance. Mean cell area data was fitted to the model shown in Eq. (7) of with a determination coefficient of 0.9230 and a variation coefficient of 9.34%.
Figure 7 Effect of varying XYL, POX, and GOX concentration on mean cell area (mm2). XYL: xylanase units; POX: peroxidase units; GOX: glucose oxidase units. A: 0.0 units of GOX units; B: 12.5 units of GOX units; C: 25 units of GOX units.
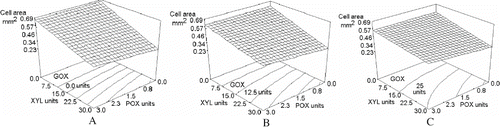
Figure 9 Effect of enzymes on crumb structure. (A) Control blank with large and elongated cells. (B) High xylanase, with smaller, less numerous and less elongated cells. (C) High xylanase and high peroxidase, with still small but a little more elongated pores. (D) High xylanase plus high glucose oxidase, with larger and elongated cells, close to the blank.
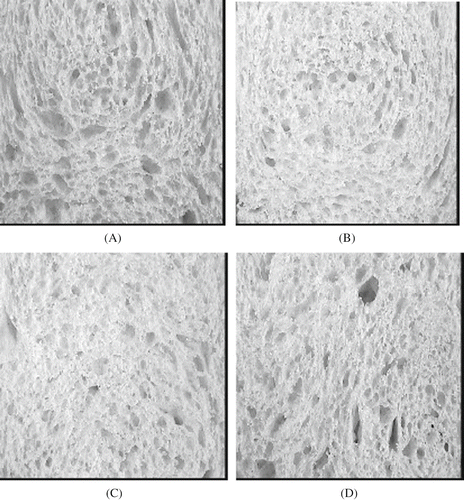
Cell form
Cell circularity was evaluated using the shape factor (S). The closer the shape factor resulted to a value of one, the more circular and less elongated the crumb cells were. The response surface of was mainly affected by xylanase. It resulted almost flat and mostly inclined in the xylanase axis, with a slight inclination on the peroxidase axis and a slope change while varying the glucose oxidase concentrations. Increases in xylanase concentration resulted in larger shape factor values and so in rounder and less elongated cells, which can be observed in the photos of . This effect was slightly affected by POX or GOX concentration changes, and in all cases these two enzymes counteracted the xylanase effect, decreasing the shape factor values achieved by this enzyme alone: GOX increments ( to ) reduced the slope of the surface in the xylanase axis, in absence of POX. With no GOX in the formulation ( A), the increments in POX concentration made the effect of xylanase decreases slightly. These changes in shape factor mean that POX alone or GOX alone do not change significantly the shape of the cells, while xylanase favour the formation of smaller and rounder cells, which can be again elongated if XYL is combined with GOX. Statistical analysis showed that shape factor was significantly affected by xylanase (P < 0.05), and peroxidase (P = 0.0322) as well by the interaction between GOX and XYL (P = 0.0419). Data for shape factor was fitted to the model in Eq. (8) of , with a determination coefficient of 0.9379 and a variation coefficient of 0.82%.
Figure 8 Effect of XYL, POX and GOX concentration on shape factor (S). S: Shape factor; XYL: xylanase units; POX: peroxidase units; GOX: glucose oxidase units. A, D: 0.0 units of GOX; B, E: 12.5 units of GOX; C, F: 25 units of GOX.
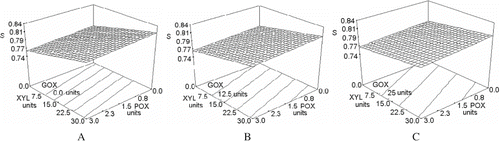
The effect of enzymes in crumb cell characteristics may be related to the change in dough rheology during mixing and fermentation, probably due to changes in viscosity and in the resistance to extension of doughs. When xylanases are in the formula, they promote an increase in dough viscosity to a maximum and if there are not enough oxidant agents, viscosity could start to decrease. On the other hand, when H2O2 and peroxidases are in the formula they promote the oxidative gelation of arabinoxylans, which increase dough viscosity, and the cross linking of flour proteins, changing dough viscoelastic characteristics. During the fermentation, yeast will produce additional H2O2[Citation32] making dough even more elastic. The balance among these properties may be related to the characteristics of crumb structure. The precise mechanism remains to be studied.
CONCLUSION
The effect towards farinographic and extensographic properties of dough prepared with low protein wheat flour, as well as the quality of bread prepared with that dough, resulted more pronounced when Glucose oxidase, Peroxidase or Xylanase were added alone than when used blended. Using the mixture of the three enzymes did not provoke a synergetic addition of their positive single effects. The use of the enzymatic blends hindered the positive effects caused by XYL alone or POX alone on farinographic water absorption, giving a response almost similar to the control value. Those blends reduced the maximum consistency time to a minimum value, achieved when the three enzymes were added at their higher concentrations. Farinographic stability reached its minimum value when glucose oxidase and xylanase were added at their higher concentrations. Single enzymes increased and blended enzymes decreased the extensographic resistance to extension while dough extensibility decreased only in the presence of GOX. Bread volume was not improved by any enzyme combination and it was even reduced with single enzyme addition. Crumb structure was affected with the addition of XYL, reducing the pore number and the pore size. These results may be ascribed to the change in the rheological properties through viscosity and resistance to extension variations, as the result of the interactions among enzyme activities and coupled reactions competing for limiting substrates.
ACKNOWLEDGMENTS
This research was financed by project 20030635 from the Instituto Politécnico Nacional (IPN, Mexico). Miss A. Garrido-Castro and Mr. J.C. Pescador-Piedra wish to thank IPN for a PIFI study grant.
REFERENCES
- Indrani , D. , Prabhasankar , P. , Rajiv , J. and Venkateswara , R. 2003 . Scanning electron microscopy, rheological characteristics and bread-baking performance of wheat-flour dough as affected by enzymes . Journal of Food Science , 68 ( 9 ) : 2804 – 2809 .
- Primo-Martín , C. , Valera , R. and Martínez-Anaya , M.A. 2003 . Effect of pentosanase and oxidases on the characteristics of doughs and the glutenin macropolymer . Journal of Agriculture and Food Chemistry , 51 : 4673 – 4679 .
- Rosell , C.M. , Wang , J. , Aja , S. , Bean , S. and Lookhart , G. 2003 . Wheat flour proteins as affected by transglutaminase and glucose oxidase . Cereal Chemistry , 80 ( 1 ) : 52 – 55 .
- Hilhorst , R. , Dunnewind , B. , Orsel , R. , Stegeman , P. , van Vliet , T. , Gruppen , H. and Schols , H.A.. 1999 . Baking performance, rheology and chemical composition of wheat dough and gluten affected by xylanase and oxidative enzymes . Journal of Food Science , 64 ( 5 ) : 808 – 813 .
- Jelaca , S.L. and Hlynca , I.. 1972 . Effect of wheat flour pentosans in dough, gluten and bread . Cereal Chemistry , 49 : 489 – 495 .
- Vemulapalli , V. , Miller , K. and Hoseney , R.C. 1998 . Glucose oxidase in breadmaking systems . Cereal Chemistry , 75 ( 4 ) : 439 – 442 .
- Wikström , K. and Eliasson , A.C. 1998 . Effects of enzymes and oxidizing agents on shear stress relaxation of wheat flour dough: additions of protease, glucose oxidase, ascorbic acid and potassium bromate . Cereal Chemistry , 75 ( 3 ) : 331 – 337 .
- Miller , K.A. and Hoseney , R.C. 1999 . Effect of oxidation on the dynamic rheological properties of wheat flour-water doughs . Cereal Chemistry , 76 ( 1 ) : 100 – 104 .
- Harada , O. , Lysenko , E.D. and Preston , K.R. 2000 . Effects of commercial hydrolytic enzyme additives on Canadian short process bread properties and processing characteristics . Cereal Chemistry , 77 ( 1 ) : 70 – 76 .
- Dunnewind , B. , van Vliet , T. and Orsel , R. 2002 . Effect of oxidative enzymes on bulk rheological properties of wheat flour doughs . Journal of Cereal Science , 36 : 357 – 366 .
- Hozova , B. , Dodok , L. , Oleksaková , J. and Moravciková , P. 2006 . Choice of cereal raw materials and suitable combinations of additives for sensory quality improvement of pastry . International Journal of Food Properties , 9 ( 4 ) : 897 – 906 .
- Prabhasankar , P. , Indrani , D. , Jyotsna , R. and Venkateswara , R.G.. 2004 . Influence of enzymes on rheological, microstructure and quality characteristics of parotta-an unleavened Indian flat bread . Journal of the Science of Food and Agriculture , 84 ( 15 ) : 2128 – 2134 .
- Pyler , E.J. 1988 . Baking Science and Technology , Sosland : Pu.Co., Merriam, KS .
- Sun , D.W. and Du , C.J. 2004 . Recent developments in the applications of image processing techniques for food quality evaluation . Trends in Food Science and Technology , 15 : 230 – 249 .
- Lagrain , B. , Boeckx , L. , Wilderjans , E. , Delcour , J.A. and Lauriks , W. 2006 . Non-contact ultrasound characterization of bread crumb . Application of the Biot-Allard model, Food Research International , 39 : 1067 – 1075 .
- Ramesh , M.N. 2001 . An application of image analysis for the study of kinetics of hydration of milled rice in hot water . International Journal of Food Properties , 4 ( 2 ) : 271 – 284 .
- Moore , M.M. , Heinbockel , M. , Dockery , P. , Ulmer , H.M. and Arendt , E.K. 2006 . Network formation in gluten-free with the application of transglutaminase . Cereal Chemistry , 83 ( 1 ) : 28 – 36 .
- Collar , C. , Bollaín , C. and Angioloni , A. 2005 . Significance of microbial transglutaminase on the sensory, mechanical and crumb grain pattern of enzyme supplemented fresh pan breads . Journal of Food Engineering , 70 : 479 – 488 .
- Rouillé , J. , Della-Valle , G. , Devaux , M.F. , Marion , D. and Dubreil , L. 2005 . French bread loaf volume variations and digital image analysis of crumb grain changes induced by the minor components of wheat flour . Cereal Chemistry , 82 ( 1 ) : 20 – 27 .
- Kihlberg , I. , Öström , A. , Johansson , L. and Risvik , E. 2006 . Sensory qualities of plain white pan bread: influence of farming system, year of harvest and baking technique . Journal of Cereal Science , 43 : 15 – 30 .
- Zghal , M.C. , Scanlon , M.G. and Sapirstein , H.D. 2001 . Effects of flour strength, baking absorption, and processing conditions on the structure and mechanical properties of bread crumb . Cereal Chemistry , 78 ( 1 ) : 1 – 7 .
- Crowley , P. , Grau , H. and Arendt , E.K. 2000 . Influence of additives and mixing time on crumb grain characteristics of wheat bread . Cereal Chemistry , 77 ( 3 ) : 370 – 375 .
- Moers , K. , Courtin , C.M. , Brijs , K. and Delcour , J.A.. 2003 . “ A screening method for endo-β-1,4-xylanase substrte selectivity ” . In In Recent Advances in Enzymes in Grain Processing, Proceedings of the Third Symposium on Enzymes in Grain Processing. Katholieke Universiteit Leuven, Belgium, Sept 25–27, 2002 , Edited by: Courtain , C.M. , Veraberbeke , W.S. and Delcour , J.A . Belgium : ACCO .
- Calderón-Domínguez , G. , Neyra-Guevara , M. , Farrera-Rebollo , R. , Arana-Errasquín , R. and Escobedo , Mora- . 2003 . Structural and farinographic changes during mixing of a yeast sweet dough . Nahrung , 47 ( 5 ) : 312 – 319 .
- Oliver , J.R. and Allen , H.M. 1992 . The prediction of bread baking performance using the farinograph and extensograph . Journal of Cereal Science , 15 : 79 – 89 .
- American Association of Cereal Chemists . 2000 . Approved Methods of the AACC . St. Paul, MN, USA.
- Mendoza , F. and Aguilera , J.M. 2004 . Application of Image Analysis for Classification of Ripening Bananas . Journal of Food Science , 69 ( 9 ) : E471 – E477 .
- Bertrand , D. , Le Guerneve , C. , Marion , D. , Devaux , M.F. and Robert , P. 1992 . Description of textural appearance of bread crumb by video image analysis . Cereal Chemistry , 69 ( 3 ) : 257 – 261 .
- Aguilera , J.M. 2001 . Tamaño y forma de las partículas. in Métodos para medir propiedades físicas en industrias de alimentos , Edited by: Alvarado , JD and Aguilera , JM . 29 – 48 . Zaragoza, Spain : Editorial Acribia .
- Petit-Benvegnen , MD. , Saulnier , L. and Rouau , X. 1998 . Solubilisation of arabinoxylans from isolated water-unextractable pentosans and wheat flour doughs by cell wall degrading enzymes . Cereal Chemistry , 75 ( 4 ) : 551 – 556 .
- Wang , M. , van Vliet , T. and Hamer , R.J. 2004 . Evidence that pentosans and xylanase affect the re‐agglomeration of the gluten network . Journal of Cereal Science , 39 : 341 – 349 .
- Liao , Y. , Miller , R.A. and Hoseney , R.C. 1998 . Role of hydrogen peroxide produced by baker's yeast on dough rheology . Cereal Chemistry , 75 ( 5 ) : 612 – 616 .
- Labat , E. and Rouau , X. 2002 . Morel M.H.. Effect of flour water extractable pentosans on molecular association in gluten during mixing . Lebensmittel-Wissenschaft und Technologie , 355 : 185 – 189 .
- Michniewicz , J. , Biliaderis , C.G. and Bushuk , W.. 1991 . Effect of added pentosans on some physical and technological characteristics of dough and gluten . Cereal Chemistry , 68 ( 3 ) : 252 – 258 .
- Poulsen , C. and H⊘strup , B. 1998 . Purification and characterization of a hexose oxidase with excellent strengthening effects in bread . Cereal Chemistry , 75 ( 1 ) : 51 – 57 .
- Primo-Martín , C. , Lichtendonk , W.J. , Plijter , J.J. , Wang , M. and Hamer , R.J. . Explaining the synergy between pentosanase and glucose-oxidase . Proceedings of the Third Symposium on Enzymes in Grain Processing. Katholieke Universiteit Leuven . Sept 25–27 2002 , Belgium. Recent Advances in Enzymes in Grain Processing , Edited by: Courtain , C.M. , Veraberbeke , W.S. and Delcour , J.A . Belgium : ACCO .
- Vemulapalli , V. and Hoseney , R.C. 1998 . Glucose Oxidase effect on gluten and water solubles . Cereal Chemistry , 7 ( 6 ) : 859 – 862 .
- Rasiah , I.A. , Sutton , K.H. , Low , F.L. , Lin , H-M. and Gerrard , J.A. 2005 . Crosslinking of wheat dough proteins by glucose oxidase and the resulting effects on bread and croissants . Food Chemistry , 89 ( 3 ) : 325 – 332 .
- Takasaki , S. , Kato , Y. , Murata , M. , Homma , S. and Kawakishi , S. 2005 . Effects of peroxidase and hydrogen peroxide on dityrosine formation and the mixing characteristics of wheat flour dough . Bioscience, Biotechnology- Biochemistry , 69 ( 9 ) : 1686 – 1692 .
- Belton , P.S. 1999 . On the elasticity of wheat gluten . Journal of Cereal Science , 29 : 103 – 107 .
- Cauvain , S.P. , Whitworth , M.B. and Alava , J.M. 1999 . “ The evolution of bubble in bread doughs and its effect on bread structure ” . In Bubbles in Food , Edited by: Campbell , G.M. , Webb , C. , Pandiella , S.S. and Niranjan , K. St. Paul MN : American Association of Cereal Chemists .