Abstract
Headspace volatile flavor compounds of kefir were monitored using headspace solid phase microextraction (HS-SPME) method during fermentation of milk with kefir starter culture. Among all flavor compounds, forty volatile compounds were initially detected using gas chromatography coupled to time-of-flight mass spectrometer (GC-TOFMS). Consequently, eight volatile flavor compounds, namely ethanol, ethyl acetate, ethyl butyrate, 2-butanone, acetone, 3-hydroxy-2-butanone (acetoin), 2,3-butanedione (diacetyl) and acetaldehyde were considered as the representative of the alcohol, ketone, ester and aldehyde compounds in kefir. Moreover, in term of quantitative analysis, more than 97% of total flavor compounds composed of the proposed volatile flavor compounds. The results indicated that the concentration of 2-butanone released into headspace of kefir was found to be stable during fermentation. The release content of other volatile flavor compounds increased throughout the fermentation process. However, the headspace concentration of acetoin significantly (P < 0.05) decreased between pH 5.2 and 4.6.
INTRODUCTION
Kefir is the product of milk fermentation with kefir grains and mother cultures prepared from grains.[Citation1] The industrial manufacture of kefir using grains as the starter culture is very difficult due to the complexity of their microbiological composition.[Citation2,Citation3] Furthermore, the microflora of kefir grains varies widely depending on the origin of grains,[Citation2,Citation4,Citation5] storage and handling conditions[Citation2] and kefir preparation methods.[Citation4] Subsequently, it is difficult to maintain the stability of the microbiological composition of the kefir grains during the production of consecutive batches.[Citation2] Microflora of a kefir grains is in symbiotic equilibrium, but the species and quantitative structure of the various groups of microorganisms significantly changed during kefir production procedure.[Citation3] The most reasonable approach for obtaining a high quality product with consistent characteristics is to use defined strains of starter cultures, which give the desired characteristics compared with the kefir grains.[Citation2] The use of starter cultures facilitates kefir production, leading to produce controlled and standardized kefir.[Citation3,Citation6]
One of the basic functional requirements induced by starter cultures in fermented milks is their ability to produce aroma compounds.[Citation7,Citation8] The amount of volatile compounds is important in acceptability of the products by consumers. The production of high perceived quality fermented milks can be achieved by qualitative and quantitative analyses of volatile flavor compounds.[Citation9,Citation10] There are several techniques that can be used to monitor changes of volatile compounds and among them are conventional gas chromatography (GC) and headspace solid-phase microextraction (HS-SPME) methods. Vilanova et al. [Citation11] observed significant differences among red grape cultivars (Caino Tinto, Caino Longo and Caino Bravo) with respect to the concentrations of aromatic compounds tested with GC. HS-SPME was successfully used to analyze target flavor compounds in orange beverage emulsion. [Citation12]
The LAB and yeast strains tested in this study were selected as they are commercially available and used in different types of fermented milks, e.g. traditional yoghurt, acidophilus milk, koumiss,[Citation13,Citation14] acidophilus yoghurt or ACO-yoghurt[Citation15,Citation16] and Biogurt.[Citation16] Therefore, the present study was conducted to identify and determine the volatile flavor substances formed during milk fermentation with a starter culture candidate for kefir production.
MATERIALS AND METHODS
Chemicals and Materials
New Zealand medium heat-treated skim milk powder used in this study obtained from a local supplier (Promac Enterprises Sdn Bhd, Kuala Lumpur, Malaysia). Standards of 2-butanone (≥ 99.9%), 2,3-butanedione (diacetyl, ≥ 99.4%), acetone (≥ 99.9%), acetaldehyde (≥ 99.5%), ethanol (99%), ethyl acetate (≥ 99.9%), ethyl butyrate (≥ 99.7%) and propyl butyrate (99%) were purchased from Fluka (Buch, Switzerland). 3-hydroxy-2-butanon (acetoin, 99%) standard, NaCl, NaBr, Na2SO4 were purchased from Merck (Darmstadt, Germany).
The SPME manual holder and fibers coated with 100 μm polydimethylsiloxane (PDMS), 65 μm polydimethylsiloxane–divinylbenzene (PDMS–DVB), 50/30 μm divinylbenzene–carboxen–polydimethylsiloxane (DVB–CAR–PDMS), 74 μm Carboxen-polydimethylsiloxane (CAR–PDMS), 10 ml glass vial, butyl septa (20mm diameter) and open center aluminum seal (20 mm diameter) were obtained from Supelco (Bellefonte, PA, USA). The fibers were conditioned as recommended by the manufacturer.
Lactic Acid Bacteria and Yeast Strains
Commercially available pure freeze-dried cultures of Lactobacillus acidophilus LA5, Lactobacillus bulgaricus LB12, Streptococcus thermophilus TH4 and Kluyveromyces marxianus subsp. marxianus LAF4 were obtained from Chr-Hansen (H⊘rsholm, Denmark). Concentrated pure cultures of L. acidophilus LA5, L. delbrueckii subsp. bulgaricus LB12, S. thermophilus TH4 and K. marxianus subsp. marxianus LAF4 were prepared according to Narvhus et al.[Citation17] and Gadaga et al.[Citation18]
Kefir Production and Sampling
The fermentation process performed in a two-liter double-jacketed laboratory fermentor, BIOSTAT B (Sartorius BBI Systems, Melsungen, Germany) was equipped with an inoculation flask, glass pH electrode, temperature probe, sampling port, controller for temperature and two six-bladed rushton turbines impeller. Reconstituted skim milk (12% wt/vol) was prepared using medium heat-treated skim milk powder and poured into the fermentor vessel, calculated to obtain a total volume of 1500 ml considering the volume of the DVS culture needed. The pH electrode was calibrated using standard buffer solutions at pH 4.0 and 7.0 and then screwed to the top lid of the vessel as well as temperature probe and inoculation flask. The system was then sterilized at 115°C for 15 min according to Champagne et al.[Citation19] and cooled down to fermentation temperature (37°C). Appropriate volumes of the individual thawed concentrated DVS cultures were aseptically transferred into the inoculation flask, calculated to reach to standard initial inoculums of about 7 log10 cfu/ml of each strain. After stabilization of the pH which was 6.5 ± 0.05 (mean ± SD), inoculation was performed via inoculation flask at 37°C. The pH immediately after inoculation was 6.4 ± 0.04 (mean ± SD), depending on the initial pH and the volume of the DVS culture needed for each experiment. Fermentation was allowed to continue until pH reached 4.6. Then fermentation was stopped by rapidly cooling of the double jacketed vessel down to 4°C. Immediately after inoculation, a 4 ml sample was taken aseptically using the sampling port. Consecutive samples were also taken during the fermentation process every time the pH dropped by 0.2 units. The time corresponding to each pH of sampling is shown in . These samples were used for analysis of headspace flavor compounds using HS-SPME method.
Table 1 The time (min ± SD, n = 3) corresponding to the pH of sampling
HS-SPME Procedure for Determining the Volatile Flavor Compounds
Headspace solid-phase microextraction (HS-SPME) followed by gas chromatographic (GC) method was used for determination of volatile flavor compounds. Extraction time, absorption temperature, NaCl concentration and sample dilution ratio used in HS‐SPME method were previously optimized using a fractional factorial design followed by response surface methodology. A volume of 4 ml diluted (1 % in deionized water) was pipetted and placed in a 10-ml glass vials containing magnetic stirrer and 1.2 g NaCl. The vial was tightly capped with 20-mm diameter butyl septum, sealed with 20 mm open center headspace aluminum seals and placed in a water bath maintained at 25°C. Samples were allowed to equilibrate for 5 min before analysis. Then the SPME device was inserted into the sealed vial by manually penetrating the septum and the fiber was exposed to the headspace over the sample for 60 min. A magnetic stirrer was used in all experiments. After sampling, the SPME fiber was immediately inserted into the GC injector and the fiber thermally desorbed for 5 min at 270°C.
GC Conditions
The volatile compounds were initially identified using a Hewlett-Packard 6890N GC system (Agilent Technologies, Wilmington, DE) equipped with Time-of-Flight Mass Spectrometer (TOFMS, Pegasus III, Leco Corp., St. Joseph, MI, USA). The volatile compounds were then analyzed by a Hewlett-Packard 6890 GC equipped with a flame ionization detector (FID). Compounds were separated on a HP-Innowax (60 m, 0.25 mm, 0.25 μm) capillary column (J&W Scientific, Folsom, CA, USA). The GC injection port was equipped with a 0.75 mm i.d. liner (Supelco) to minimize peak broadening.
For GC-FID, desorption was performed in the splitless mode for 5 min at 270°C. Oven temperature was programmed at 40°C for 10 min, and then increased at 5°C/min up to 100°C. Then it was ramped to 240°C at 20°C/min and held for 10 min. The carrier gas was helium at a flow rate of 1.4 ml/min. The detector temperature was set at 300°C. In GC-TOFMS, the injector and detector temperatures and oven program were the same as GC-FID. The mass spectra in the electron impact (EI) mode were obtained at 70 eV. The data obtained from GC-TOFMS was processed using the ChromaTOF software version 2.4 (LECO Corporation). The volatile flavor compounds of the sample were identified by analogy of mass spectra and matching mass spectra fragment with the NIST library version 2.0 and additional standards.
Statistical Analysis
All measurements on each sample were carried out in duplicate. Results were reported as the mean ± SD of three independent fermentations and submitted to analysis of variance (ANOVA) using MINITAB statistical software, release 13.20. (MINITAB Inc., Pennsylvania, USA). Mean values were compared using the Tukey's test at P < 0.05.
RESULT AND DISCUSSION
Identification of Volatile Flavor Compounds
Among all flavor substances extracted by HS-SPME, only forty volatile compounds including hydrocarbon, aldehyde, alcohol, ketone, ester, organic acid, sulfur and benzoic compounds were detected by GC-TOFMS. More than 97% of headspace volatile flavor compounds, quantified by GC-FID, were ethanol (39.347%), 2-butanone (31.682%), ethyl acetate (8.951%), ethyl butyrate (5.535%), acetone (3.674%), 3-hydroxy-2-butanone (acetoin, 3.239%), 2,3-butanedione (diacetyl, 2.986%) and acetaldehyde (1.770%). Thus, the corresponding flavor compounds were chosen as the representative of the volatile flavor compounds in the headspace of kefir for semi-quantitative HS-SPME analysis. They have been previously reported to be the main compounds responsible for the flavor in fermented milk products.[Citation7,Citation20] presents LRI (Linear Retention Indices), similarity and FID peak area (%) of the volatile flavor compounds in kefir sample.
Table 2 Linear retention indices, similarity, and FID peak area (%) of the representative volatile flavor compounds in kefir sample
Ketones
Among different chemical groups of target volatile flavor compounds, the ketone compounds—namely 2-butanone, acetone, 3-hydroxy-2-butanone and 2,3-butanedione—were found to be the most prominent carbonyl substance in the sample kefir headspace. -D presents the changes in ketones content of kefir headspace. 2-butanone is a characteristic flavor component of fermented milk. It is derived from the intermediate product of acetyl methyl carbinol by reduction and subsequent dehydration.[Citation21] Among target volatile compounds studied, 2-butanone was the only flavor compound observed in the sample headspace from the beginning of fermentation (). However, fluctuation in the concentration of 2-butanone was observed during fermentation. Nevertheless, the changes in its headspace concentration were found to be not significant (P > 0.05).
Figure 1 Changes in ketones concentration released in headspace during kefir production. (A): 2-butanone; (B): acetone; (C): acetoin; and (D): diacetyl.
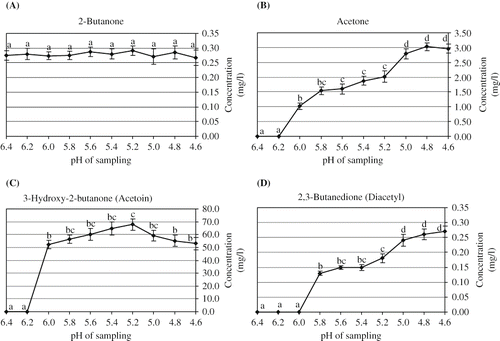
Acetone in the sample headspace was first detected at pH 6.0. Consequently, the headspace concentration of acetone increased during fermentation and reached to the maximum concentration of 3.03 mg/l after 227 min after inoculation. The headspace acetone concentration showed a slight reduction, although not significant (P < 0.05), at the final stage of fermentation and reached to final concentration of 2.96 mg/l (). This observation was in agreement with that of reported by previous researchers.[Citation9]
Acetoin is one of the metabolites generated from citrate metabolism by lactic acid bacteria.[Citation22,Citation23] In addition, during glucose catabolism, diacetyl (2,3-butanedione) serves as electrons acceptor and can be reduced to acetoin.[Citation24,Citation25] Acetoin can also be formed from acetaldehyde through α-acetolactate.[Citation22,Citation26] Diacetyl and acetoin are normally formed at rates dependent upon the rate of citrate metabolism.[Citation10] In this study, a sharp increase in acetoin formation was observed between pH 6.2 and 6.0 and reached to the highest concentration of 67.8 mg/l at pH 5.2 followed by a reduction to a final concentration of 53.1 mg/l (). As clearly shown in , the concentration of acetoin significantly (P < 0.05) decreased between pH 5.2 and 4.6. The proposed finding was also reported by Güzel-Seydim et al.[Citation27] Conversely, Beshkova et al.[Citation9] did not report acetoin formation during kefir production.
Diacetyl is another important compound for determining the specific characteristic of fermented milk flavor.[Citation28] It can be formed via citrate metabolism, which is connected to pyruvate formation. [Citation22,Citation23,Citation26,Citation29] As observed in acetaldehyde formation, diacetyl was also initially detected at pH 6.0. A significant (P < 0.05) increase in headspace concentration of diacetyl during fermentation was observed between pH 6.0–5.8 and 5.2–5.0 (). As also reported by Beshkova et al.[Citation9] diacetyl was detected at the second hour from fermentation onset. In contrast, diacetyl was not detected by Güzel-Seydim et al.[Citation30] during fermentation of kefir. In this study, an amount of 0.270 mg/l of diacetyl was detected in the final product ().
Alcohol
The formation of alcohol in alcoholic fermented milk like kefir is mainly due to the yeast metabolism.[Citation27] In the present work, ethanol and CO2 generated by yeast fermentation are critical compounds for the exotic refreshing flavor and distinctive aroma of kefir.[Citation9] As stated by Güzel-Seydim et al.,[Citation27] the higher ethanol content is associated with the more extensive yeasty flavor. In this research, ethanol exhibited an increment during the fermentation process. The production of ethanol was more intensive when the pH reached to 5.4 until the end of the fermentation reaching to the maximum concentration of 1059 mg/l (). In contrast, a lower amount of ethanol (40 mg/kg) was detected in kefir produced with the previous researchers.[Citation27]
Esters
Ethyl acetate did not detect in the headspace of the first two samples (pH 6.4 and 6.2). The presence of ethyl acetate in headspace was observed when the pH reached to 6.0. The results indicated that the concentration of ethyl acetate increased during fermentation with the maximum concentration of 2.77 mg/l at pH 4.6 (). In contrast, Beshkova et al.[Citation9] observed that the concentration of ethyl acetate declined after 16 hours of fermentation; while it was not detected in kefir produced by the other researchers.[Citation27] The formation of ethyl butyrate during kefir production was not observed previously. However, in the present study, 5.535% of the kefir headspace composed of ethyl butyrate (). It was initially observed that the production of ethyl butyrate was not significantly different (P > 0.05) at pH 5.8 – 5.0. However, the formation of ethyl butyrate started to increase significantly towards the final steps of fermentation ().
Aldehyde
Of all secondary metabolites of LAB, acetaldehyde is of primary interest compound due to its key role in the aroma of fermented milks.[Citation31,Citation32,Citation33,Citation34] In yoghurt, the pathway leading to acetaldehyde formation is generally considered to be through lactose degradation.[Citation35,Citation36] It is formed as a result of pyruvate decarboxylation or through the formation of the intermediate acetyl co-enzyme A by pyruvate formate lyase or pyruvate dehydrogenase. It can also be formed as a result of amino acid catabolism.[Citation36,Citation37] The accumulation of acetaldehyde in the growth medium can occur when the specific activities of enzymes that form acetaldehyde are higher than those (alcohol dehydrogenase) able to convert it to ethanol.[Citation36]
In this study, acetaldehyde in the sample headspace was initially observed at pH 5.8. The results showed that the headspace concentration significantly (P < 0.05) increased until pH 5.4 (). As compared with present study, Beshkova et al.[Citation9] reported higher amount of acetaldehyde formed in the beginning of the fermentation. However, Güzel-Seydim et al.[Citation27] demonstrated that a significant (P < 0.05) increase in acetaldehyde content was observed between 15 to 22 h of fermentation. In this work, the acetaldehyde content reached to the maximum concentration of 13.81 mg/l in the final product. As demonstrated by Beshkova et al.[Citation9] a concentration of 18.3 and 9.5 mg/kg acetaldehyde were found in kefir produced with kefir starter culture and kefir grains, respectively. As comparison, Güzel-Seydim et al.[Citation27] reported the lower concentration level (5 mg/kg) of acetaldehyde in kefir with kefir grains. The amount of acetaldehyde required for the development of a characteristic flavor in fermented milks varied from 10 to 40 mg/kg.[Citation38]
CONCLUSIONS
This study focused on the metabolism of a mixed starter culture and flavor formation during kefir production. Metabolic changes are very important from technological point of view, since the amount of volatile compounds directly influence the product's flavor, its organoleptic quality, and hence, the consumer acceptability. The production of kefir was successful by the proposed kefir starter culture. HS-SPME method was appropriate for semi-quantitation headspace analysis of volatile flavor compounds in kefir. Among the forty volatile flavor compounds, the most abundant flavor compounds quantified by GC-FID were ethanol (39.347%), 2-butanone (31.682%), and ethyl acetate (8.951%). Among different chemical classes of volatile flavor compounds, ketone compounds found to be the most predominant volatile compounds formed by the proposed starter culture in the fermented milk. Considerable amount of acetaldehyde and acetoin formed using the proposed kefir starter culture. Starter culture used for the sample preparation showed an appropriate profile of volatile flavor compounds, which are necessary for a typical flavor of fermented milk. Further studies will address changes in the main volatile flavor substances during refrigerated storage in combination with sensory tests. Thus, this result would be applicable to develop a lactic acid-alcoholic fermented milk.
ACKNOWLEDGMENTS
The authors gratefully acknowledge Ms. Tneh Yen Kiaw from Chr-Hansen (Kuala Lumpur, Malaysia) for donation of bacteria and yeast cultures.
REFERENCES
- Irigoyen , A. , Arana , I. , Castiella , M. , Torre , P. and Ibãnez , F.C. 2005 . Microbiological, physicochemical, and sensory characteristics of kefir during storage . Food Chemistry , 90 : 613 – 620 .
- Fontán , M.C.G , Martínez , S. , Franco , I. and Carballo , J. 2006 . Microbiological and chemical changes during the manufacture of kefir made from cows’ milk, using a commercial starter culture . International Dairy Journal , 16 : 762 – 767 .
- Simova , E. , Beshkova , D. , Angelov , A. , Ts , Hristozova , Frengova , G. and Spasov , Z. 2002 . Lactic acid bacteria and yeasts in kefir grains and kefir made from them . Journal of Industrial Microbiology and Biotechnology , 28 : 1 – 6 .
- Witthuhn , R.C. , Schoeman , T. and Britz , T.J. 2005 . Characterisation of the microbial population at different stages of kefir production and kefir grain mass cultivation . International Dairy Journal , 15 : 383 – 389 .
- Güzel-Seydim , Z. , Wyffels , J.T. , Seydim , A.C. and Greene , A.K. 2005 . Turkish kefir and kefir grains: microbial enumeration and electron microscopic observation . International Journal of Dairy Technology , 58 : 25 – 29 .
- Beshkova , D.M. , Simova , E.D. , Simov , Z.I. , Frengova , G.I. and Spasov , Z.N. 2002 . Pure cultures for making kefir . Food Microbiology , 19 : 537 – 544 .
- Kneifel , W.M. , Ulberth , F. , Erhard , F. and Jaros , D. 1992 . Aroma profiles and sensory properties of yogurt and yogurt-related products. I. Screening of commercially available starter cultures . Milchwissenschaft , 47 : 362 – 365 .
- Helland , M.H. , Wicklund , T. and Narvhus , J.A. 2004 . Growth and metabolism of selected strains of probiotic bacteria in milk- and water-based cereal puddings . International Dairy Journal , 14 : 957 – 965 .
- Beshkova , D. , Simova , E. , Frengova , G. , Simov , Z. and Zh.P , Dimitrov . 2003 . Production of volatile aroma compounds by kefir starter cultures . International Dairy Journal , 13 : 529 – 535 .
- Østlie , H.M. , Helland , M.H. and Narvhus , J.A. 2003 . Growth and metabolism of selected strains of probiotic bacteria in milk . International Journal of Food Microbiology , 87 : 17 – 27 .
- Vilanova , M. , Cortes , S. , Santiago , J.L. , Martinez , C and Fernandez , E. 2007 . Aromatic compounds in wines produced during fermentation: effect of three red cultivars . International Journal of Food Properties , 10 ( 4 ) : 867 – 875 .
- Mirhosseini , H. , Salmah , Y. , Nazimah , S.A.H. and Tan , C.P. 2007 . Solid-phase microextraction for headspace analysis of key volatile compounds in orange beverage emulsion . Food Chemistry , 105 : 1659 – 1670 .
- CODEX Alimentarius Commission. CODEX standard for fermented milks. Codex Stan 243-2003 http://www.codexalimentarius.net/download/standards/400/CXS_243e.pdf (Accessed: 14 May 2009 ).
- 1992 . International Dairy Federation , Belgium : General standard of identity for fermented milks. IDF Standard 163. IDF: Brussels .
- Scheinbach , S. 1998 . Probiotics: Functionality and commercial status . Biotechnology Advances , 16 : 581 – 608 .
- Gomes , A.M.P. and Malcata , F.X. 1999 . Bifidobacterium spp. and Lactobacillus acidophilus: biological, biochemical, technological and therapeutical properties relevant for use as probiotics . Trends in Food Science and Technology , 10 : 139 – 157 .
- Narvhus , J.A. , Østeraas , K. , Mutukumira , T. and Abrahamsen , R.K. 1998 . Production of fermented milk using a malty compound-producing strain of Lactococcus lactis subsp. Lactis biovar. diacetylactis, isolated from Zimbabwean naturally fermented milk . International Journal of Food Microbiology , 41 : 73 – 80 .
- Gadaga , T.H. , Mutukumira , A.N. and Narvhus , J.A. 2001 . The growth and interaction of yeasts and lactic acid bacteria isolated from Zimbabwean naturally fermented milk in UHT milk . International Journal of Food Microbiology , 68 : 21 – 32 .
- Champagne , C.P. , Gardner , N.J. , Soulignac , L. and Innocent , J.P. 2000 . The production of freeze-dried immobilized cultures of Streptococcus thermophilus and their acidification properties in milk . Journal of Applied Microbiology , 88 : 124 – 131 .
- Ott , A. , Fay , L.B. and Chaintreau , A. 1997 . Determination and origin of the aroma impact compounds of yogurt flavor . Journal of Agricultural and Food Chemistry , 45 : 850 – 858 .
- Keen , A.R. , Walker , N.J. and Pederby , M.F. 1974 . The formation of 2-butanone and 2-butanol in Cheddar cheese . Journal of Dairy Research , 41 : 249 – 257 .
- Hugenholtz , J. 1993 . Citrate metabolism in lactic acid bacteria . FEMS Microbiology Reviews , 12 : 165 – 178 .
- Cocaign-Bousquet , M. , Garrigues , C. , Loubiere , P. and Lindley , N.D. 1996 . Physiology of pyruvate metabolism in Lactococcus lactis . Antonie van Leeuwenhoek , 70 : 253 – 267 .
- Hugenholtz , J. and Starrenburg , J.C. 1992 . Diacetyl production by different strains of Lactococcus lactis subsp. lactis var. diacetylactis and Leuconostoc ssp . Applied Microbiology and Biotechnology , 38 : 17 – 22 .
- Snoep , J.L. , Teixeira de Mattos , M.J. , Starrenburg , M.J.C. and Hugenholtz , J. 1992 . Isolation, characterization, and physiological role of the pyruvate dehydrogenase complex and α-acetolactate synthase of Lactococcus lactis subsp. lactis biovar. diacetylactis . Journal of Bacteriology , 174 : 4838 – 4841 .
- Singh , T.K. , Drake , M.A. and Cadwallader , K.R. 2003 . Flavor of Cheddar cheese: A chemical and sensory perspective . Comprehensive Reviews in Food Science and Food Safety , 2 : 139 – 162 .
- Güzel-Seydim , Z.B. , Seydim , A.C. , Greene , A.K. and Bodine , A.B. 2000 . Determination of organic acids and volatile flavor substances in kefir during fermentation . Journal of Food Composition and Analysis , 13 : 35 – 43 .
- Oberman , H. and Libudzisz , Z. 1998 . “ Fermented milks ” . In Microbiology of Fermented Foods , Edited by: Wood , B.J.B . 309 – 350 . London : Blackie Academic and Professional .
- Caplice , E. and Fitzgerald , G.F. 1999 . Food Fermentations: role of microorganisms in food production and preservation . International Journal of Food Microbiology , 50 : 131 – 149 .
- Güzel-Seydim , Z.B. , Seydim , A.C. and Greene , A.K. 2000 . Organic acids and volatile flavor components evolved during refrigerated storage of kefir . Journal of Dairy Science , 83 : 275 – 277 .
- Bottazzi , V. , Battistotti , B. and Montescani , G. 1973 . Influence of single and associated strains of L. bulgaricus and S. thermophilus as well as milk treatments on the production of acetaldehyde in yogurt . Lait , 53 : 295 – 308 .
- Imhof , R. and Bosset , J.O. 1994 . Relationships between microorganisms and formation of aroma compounds in fermented dairy products . Zeitschrift Lebensmitel Unters Forschung , 198 : 267 – 276 .
- Urbach , G. 1995 . Contribution of lactic acid bacteria to flavor compound formation in dairy products . International Dairy Journal , 5 : 877 – 903 .
- Ott , A. , Hugi , A. , Baumgartner , M. and Chaintreau , A. 2000 . Sensory investigation of yogurt flavor perception: Mutual influence of volatiles and acidity . Journal of Agriculture and Food Chemistry , 48 : 441 – 450 .
- Marshall , V.M. 1987 . Lactic acid bacteria: Starters for flavour . FEMS Microbiology Reviews , 46 : 327 – 336 .
- Gonzalez , S. , Morata de Ambrosini , V. , Manca de Nadra , M. , Pesce de Ruiz Holgado , A. and Oliver , G. 1994 . Acetaldehyde production by strains used as probiotics in fermented milk . Journal of Food Protection , 57 : 436 – 490 .
- Raya , R.R. , Manca de Nadra , M.C. , Pesce de Ruiz Holgado , A. and Oliver , G. 1986 . Acetaldehyde metabolism in lactic acid bacteria . Milchwissenschaft , 41 : 397 – 399 .
- Tamime , A.Y. and Robinson , R.K. 1999 . “ Biochemistry of fermentation ” . In Yoghurt: Science and Technology , Edited by: Tamime , A.Y. and Robinson , R.K. 433 – 485 . Oxford : Pergamon Press .