Abstract
The effect of mixing time on two Argentinean commercial flours (FI and FII) was studied. Both flours showed a similar electrophoresis profile but different content of free sulfhydryls. Rheology of dough obtained from flours (DI and DII) revealed that DI presented a more elastic matrix. FI required more than 8 min for an optimum development of gluten, while DII was already fully developed (development time: 7.3 min). Up to 16 min of mixing, DI matrix maintained the sheet like structure while DII showed a more filamentous one, characteristic of a weak and over kneaded dough. This weakness of gluten network obtained from FII was accompanied by a depolymerization process.
INTRODUCTION
The uniqueness properties of wheat flour dough required for breadmaking are derived from the type and quantity of gluten proteins. Gluten proteins, containing mainly gliadin and glutenin, play an important role in bread making. It is generally accepted that disulphide bonds (SS) formed by oxidation of sulphydryl groups (SH) are important in stabilizing the folded conformation of gliadin (monomeric proteins) and in stabilizing the polymer structure of glutenin.[Citation1–4]
Glutenins are composed of high molecular weight (HMW-Glu) and low molecular weight (LMW-Glu) subunits. Many authors have focused their studies on the different HMW subunits, because of the influence these proteins appear to have, on the rheological properties of dough.[Citation5–7] It is well accepted that the viscoelastic properties of dough, which govern breadmaking quality, result not only from the interaction between glutenin polymers but also from the interaction of glutenins with the monomeric gliadin proteins. It is well known[Citation8] that the ratio of gliadins to glutenin proteins is an important factor that influences breadmaking quality, and some authors have claimed that gliadins also have a direct effect on this parameter.[Citation9–12] Van Lonkhuijsen et al.[Citation12] founded a strong relation between loaf volume of bread and the content of certain types of gliadins in wheat varieties with constant composition of certain HMW glutenin-A subunit (−, 7, 2 + 12).
The balance between gliadins and glutenins is related to important rheological properties such as viscosity and elasticity. Viscosity is generally associated with the monomer proteins gliadins, while elasticity of dough derives from the glutenin component, which comprises polydisperse polymers of disulfide-bonded polypeptides.[Citation13] In addition, specific viscoelastic properties of wheat flour dough are conferred by these gluten proteins.[Citation14]
To evaluate flour quality, the mills and bakery industries employ common rheological techniques such as alveographic, farinographic, and mixographic measurements. Recording dough mixers, such as the Farinograph and Mixograph, have been developed for specific use in the cereal industry. Different parameters of flours such as development time, stability and dough softening can be recorded during mixing by measuring the resistance of the dough to mixing, by these techniques; while in the Alveograph, dough is subjected to biaxial extension.[Citation15,Citation16] These techniques usually can differentiate flours of different characteristics and are useful in practical applications, but they have the disadvantage, however, of being mostly empirical in nature.[Citation17]
Rheological techniques are well suited for the characterization of viscoelastic materials such as wheat flour dough[Citation17] and wheat gluten.[Citation18] Dynamic measurements have been employed extensively for studying fundamental rheological testing of wheat flour dough.[Citation19,Citation20] Several authors studied the relationship between dough rheological properties and microstructure characteristics of wheat gluten.[Citation21–24] Zheng et al.[Citation25] found changes in dough rheology, specifically shear and extensional properties, occurring during different stages of the mixing process. Changes in dough rheology due to mixing are strongly related to depolymerization process. Skerrit et al.[Citation26] studied the depolymerisation of the glutenin macropolymer (GMP) during dough mixing. After extensive mixing, molecular weight of GMP decreases and glutenin polymers are disrupted and released from dough. The partially depolymerization of glutenin, with the production of molecules of low molecular mass, produces a low consistency and high stickier dough.[Citation21]
Quality of commercial Argentinean wheat flours is an important factor for different bakery purposes. These flours are usually a blend of almost two wheat varieties and they use to present high quality, as measured by the tenacity (P) to extensibility (L) ratio (P/L > 1), comparable to those produced in other countries.[Citation27,Citation28] In this work, two commercial wheat flours, used to formulate industrial pie dough but having different extensional characteristics, were chosen. One of them was better suitable for this industrial application, although they presented similar composition and equal alveographic properties. Therefore, the objectives of this study were: (a) to analyze the influence of mixing time on the rheological characteristics of each commercial Argentinean wheat flour and (b) to study the relationship between rheological and structure properties of dough.
MATERIALS AND METHODS
Wheat Flour Samples
Two commercial wheat flours derived from different Argentinean mills (type 0000 - Argentinean Codex Alimentarius, 1992) were used in the experiments: FI (Molino Campodónico Ltda., Argentina) and FII (Cargill SACI, Argentina). Flours were chosen because of their similar composition characteristics but different rheological properties. Moisture, ash, protein, and gluten contents were determined according to AACC Approved Methods 44-15A, 8-03, 46-10 and 38-12A, respectively.[Citation29]
Mixing Properties of Flours
Flour mixing properties were analyzed by farinographic and mixographic assays. Water absorption (%), development time (min), stability (min), and degree of softening (Brabender Units, BU) were measured in a 300 g Brabender farinograph (Brabender OHG, Duisburg, Germany).[Citation30] Parameters such as peak time (min), peak height (%Tq), peak width (%), and area (%Tqxmin) were measured in a 10 g Mixograph (National Manufacturing Division, TMCO, Lincoln, Nebraska, USA) using AACC Method 54–40.[Citation31]
Alveograph Measurements
Extensibility properties of dough were measured using the Chopin alveograph test according to IRAM methods.[Citation30] The mixing time corresponding to the alveographic technique is 8 min. Two higher mixing times (16 and 24 min) were also analyzed. Alveographic parameters such as dough strength (W), dough tenacity (P), dough extensibility (L) and tenacity-to-extensibility ratio (P/L) were calculated from the alveograms.
Preparation of Gluten and Dough
Gluten from FI (GI) and FII (GII) flours was prepared according to the 15864-IRAM method.[Citation30] Commercial gluten (Molinos Juan Semino, S.A.) was used as a standard sample.
Dough from FI (DI) and FII (DII) was prepared in a 10 g-Brabender Farinograph at 30°C at different mixing times (8, 16, and 24 min). The amount of distilled water added to the flour was adjusted to provide dough with a maximum consistency of 500 BU.
Scanning Electron Microscopy (SEM)
Dough samples (DI and DII) obtained from Chopin Alveograph and 10 g-Brabender Farinograph, were immersed in 2.5% glutaraldehyde and then washed with phosphate buffer 0.5 M before dehydration process. Samples were dehydrated in a grade acetone series: 25, 50, and 75% and three times with 100%. Dough samples were totally dried using the intermediate CO2 fluid at its critical point. This treatment was performed with the objective of preserving structure during the electron incidence of dough. Samples were then coated with gold in a sputter coater (Pelco, Redding, CA, USA). They were observed at 5 kV voltages in a JEOL JSM 35 CF microscope (Tokyo, Japan) scanning electron microscope.
Instrumental Texture Profile Analysis (TPA)
Dough samples (DI and DII), prepared in the 10 g-Brabender Farinograph, were studied using Texture Profile Analysis (TPA). Cylindrical samples (10 pieces) of diameter 2 cm and height 1 cm were obtained from dough. Samples were compressed at 20°C to 75% of their original height. A plate-plate sensor system with a stainless probe SMSP/75 at a constant rate of 0.5 mm/s was used. Texture of dough was assayed by a uniaxial compression test of two cycles (TPA)[Citation32] using a TA-XT2i Texture Analyzer (Vienna Court, Surrey, England). Parameters such as hardness, adhesiveness, cohesiveness and consistency were analyzed. Hardness is the maximum force obtained during the first compression cycle. Adhesiveness is the negative area obtained during the first cycle. Cohesiveness was obtained as the ratio between the positive areas of the second cycle and the first cycle. Consistency is the sum of the positive areas of the first and the second cycles.
Dynamic Rheological Testing
Dynamic measurements were carried out at 30°C in a Haake RS600 rheometer (Haake Mess-Technik, GmbH u. Co., Karlsruhe, Germany). A parallel-plate sensor was used, with a 1-mm gap between plates. After dough samples, DI and DII, were placed in the lower plate, a thin film of petroleum jelly was spread on the sample contour and the plate edges to prevent dehydration. Frequency scans at a constant deformation within the linear viscoelasticity range (4.5% deformation) were performed. Storage modulus (G') and tan δ (G”/G') at 1 Hz frequency were analyzed.
Free Sulfhydryls (SHF) Determination
Free sulfhydryls (SHF) (μmoles/g of flour, db) were determined in flours and dough through the colorimetric test in solid phase modified by Chan and Wasserman.[Citation33] Dough was prepared mixing wheat flour (10 g) with distilled water at different times (8, 16, and 24 min) in a 10 g-Brabender Farinograph at 30°C. Samples of 30-mg flour or 120-mg dough were suspended in 1.0 mL of reaction buffer (RB) containing urea 8 M, EDTA 3 mM, SDS 1%, Tris-HCl 0.2 M and 5,5'-dithio-bis (2-nitrobenzoic acid) (DTNB) 10 mM-pH 8.0, and constantly stirred for 1 hr under nitrogen atmosphere. After centrifugation for 10 min at 13600 × g at 10°C, 0.1 mL aliquots were mixed with 0.9 mL of dilution buffer (DB) (reaction buffer without DTNB). Absorbance was measured at 412 nm in a Beckman spectrophotometer (DU 650 Model, Beckman instruments Inc., Berkeley, California, USA).
SDS-PAGE
Protein extraction from flours (FI, FII), gluten (GI, GII) and dough (DI, DII) was performed with a buffer containing Tris-HCl 0.125 M, SDS 1% v/v, urea 6 M, β-mercaptoethanol 5% p/v (pH 6.8) for 2.5 h at room temperature (1:10, flour base). Samples were heated in boiling water for 10 min to enhance disulphide bond reduction. Protein extract was diluted with an equal volume of a pH 6.8 buffer (0.125M Tris-HC1, 0.1% SDS, 40% v/v glycerol, 0.05% bromophenol blue). Slab gels formed by a 10% continuous gel and a 4% stacking gel in the top were used. In each lane, an equal protein quantity of each extract was loaded. Electrophoresis was performed in a Mini Protean II at a constant voltage of 60 V (stacking gel) and 120 V (continuous gel) with a Power-Pack 300 (Bio-Rad, Richmond, CA, USA). Low molecular weight standards (LMW) of 94 (phosphorylase b), 67 (albumin), 43 (ovalbumin), 30 (carbonic anhydrase), 20.1 (trypsin inhibitor), and 14.4 (α-lactalbumin) kD were used (Pharmacia calibration kit).
Statistical Analysis
All analyses were performed in duplicate. Comparison of means corresponding to results from physicochemical and rheological characterization of flours was performed by applying the Student's t-test (p < 0.05).
RESULTS AND DISCUSSION
Physicochemical and Rheological Characterization of Flours
Selected composition parameters of flours are shown in . Moisture and protein content of flours was not significantly different (p < 0.05). However, ash and gluten content of FII were significantly higher than values obtained for FI. Moreover, FI presented a higher content of free sulfhydryls (SHF: 2.43 ± 0.09 μmoles SH/g flour, db) than flour FII (SHF: 1.42 ± 0.09 μmoles SH/g flour, db). Starch damage values were calculated from the linear correlation between this parameter and the alveographic P/L, with data obtained for Argentinean flours by Moiraghi et al.[Citation34] and Ponzio et al.[Citation35] A linear correlation with an r2 = 0.8726 was obtained (P/L = 0.338 SD% − 1.7497). Starch damage content of FI (10.5%) was not significantly different of that obtained for FII (10.0%).
Table 1 Selected composition parameters of wheat flours FI and FII
Data corresponding to rheological characterization of flours are shown in . Although no significant differences (p < 0.05) were obtained between water absorption, flour FI showed longer farinographic development time and produced more stable dough with less softening degree than flour FII. Mixograms reflected a comparable behavior than farinograms: they presented a similar form with a pronounced peak at the peak time (data not shown). Times of maximum torque, band width at this time and mixogram area, are related with flour strength. The highest parameters will be obtained with the more strong flours. It was necessary to mix flour FI for longer times (peak time) than FII to develop the dough. Differences in the resistance to mixing between both flours could be attributed to the distinct gliadin to glutenin ratio, and the amount of high molecular mass glutenin polymers soluble in SDS.[Citation31] No significant differences in alveographic parameters (8 min of mixing) were obtained between both flours, while farinograms and mixograms were clearly different.
Table 2 Common rheological characterization of wheat flours: mixograph, farinograph and alveograph parameters
Extensional Properties of Dough
shows the effect of mixing time in alveographic parameters of FI and FII dough. No differences in alveographic parameters (P, L, W) between flours were observed for the equal and common mixing time established in the alveographic technique: 8 min (; ). However, when applying high mixing times differences were observed between flours. Tenacity in FI increased, while in FII decreased at mixing times higher than 8 min (). Extensibility decreased with the increase in mixing time (), while the highest P/L ratio was obtained for FI dough (). A different alveographic work behavior was observed for both type of dough (). FI presented the highest values of mixing work at times higher than 8 min, especially at 16 min. FI required more than 8 minutes to achieve the optimum gluten network. This behavior was also detected with farinographic assays: FI needed more than 16 min to reach the optimum gluten network, while 8 min were enough for FII. Therefore, under the conventional conditions established for alveographic dough preparation, FI resulted underdeveloped. On the other hand, FII was already fully developed at 8 min and higher mixing times led to a weaker, over kneaded dough. These findings were confirmed by the microstructure analysis. Structure of dough prepared in Chopin Alveograph (SEM) is shown in . Gluten matrix exhibits a sheet-like structure wrapping starch granules. Paredes-Lopez and Bushuk[Citation36] reported that gluten in developed dough had a continuous membrane-like structure.
Figure 1 Changes in alveographic parameters of wheat flour dough with mixing time. (a) Tenacity, P; (b) extensibility, L; (c) P/L ratio; (d) work for mixing process. Flours: (•) FI, (▪) FII. Bars indicate standard deviations. Not evident bars are overlapped with symbols.
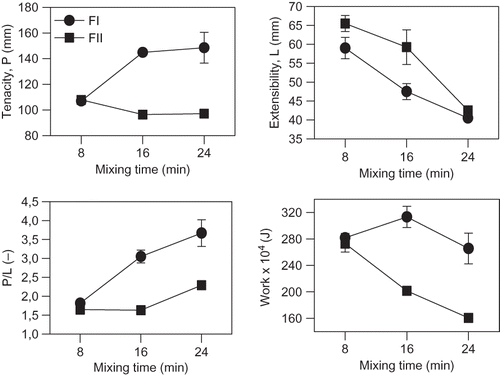
Figure 2 SEM photographs of doughs prepared in Chopin Alveograph. Magnification: 2200 x. Dough prepared with FI and FII flours. Mixing times: 8, 16, and 24 min.
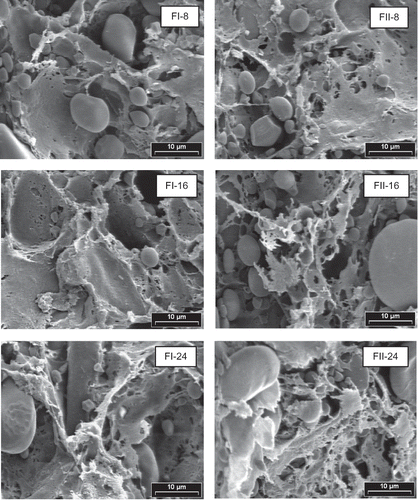
At mixing time of 8 min, network was still being formed in FI, while in FII showed an incipient degree of structural disruption (). When mixing time increased (16 min), matrix of FI dough was not appreciably modified compared to dough kneaded 8 min, in contrast, structure of FII evidenced a more pronounced disruption than at 8 min. Up to 16 min of mixing time, matrix of FI maintained the sheet like structure while FII developed a more filamentous one. With mixing times of 24 min, dough was over kneaded showing a more disrupted gluten structure in both cases.
Texture Properties of Dough
shows texture parameters of DI and DII dough obtained from the TPA analysis. TPA parameters simulate different actions of mastication process. Hardness and consistency are related to resistance of food to first and total mastication, respectively. Adhesiveness is the force that it must be done so as to remove food from palate. Cohesiveness is related to interaction forces that maintain food particles assembled. These parameters of raw dough can be correlated with bread quality. DI presented, at 8 min of mixing similar hardness and consistency than DII; while at 16 min of mixing, DI developed the hardest and consistent dough. At 24 min, both kind of dough decreased their hardness and consistency. DII dough, at 8 min of mixing, was more adhesive than DI dough. At 16 min of mixing, DI increased the adhesiveness, while at higher mixing times (24 min) the adhesiveness decreased in both type of dough. For both DI and DII, different behavior of cohesiveness with time was also observed. Cohesiveness of DI dough was lower than for DII and decreased after 16 min of mixing; while DII did not present changes in this parameter with mixing time. The cohesiveness, from the rheological point of view, is the ability of a material to stick to itself. Therefore, FI formed at 16 min hardest dough but with less ability of maintaining dough particles connected.
Figure 3 Texture profile analysis (TPA) of wheat flour dough: (•) DI, (▪) DII. (a) hardness; (b) adhesive force; (c) adhesiveness; (d) elasticity; (e) cohesiveness; (f) consistency. Dough: (•) DI, (▪) DII. Bars indicate standard deviations.
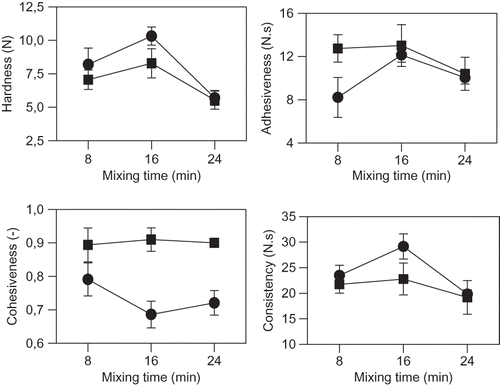
Structure of dough prepared in Brabender Farinograph as analyzed by SEM is shown in . For both flours, FI and FII, the gluten network was not fully developed at 8 min. After 16 min of mixing, both FI and FII dough showed a more developed structure though FI exhibits a more homogeneous network. The greater development of gluten network is reflected in dough hardness () where values at 16 min mixing time are higher than at 8 min. At 24 min of mixing, when both FI and FII dough were over mixed, a higher degree of rupture of the protein matrix was observed, along with a drastic decrease in dough hardness. The microstructure basis for the dough breakdown by mixing was analyzed by Skerrit et al.[Citation26] These authors reported that the amount of GMP and the average apparent molecular weight of GMP decreased during extensive mixing. Glutenin polymers and oligomers were released but not free glutenin subunits. During dough breakdown, the composition of GMP was altered, leading to changes in the molecular weight distribution and composition of the disulfide-bonded GMP.
Figure 4 SEM photographs of doughs prepared in Brabender Farinograph. Magnification: 2600 x. Dough prepared with FI and FII flours. Mixing times: 8, 16, and 24 min.
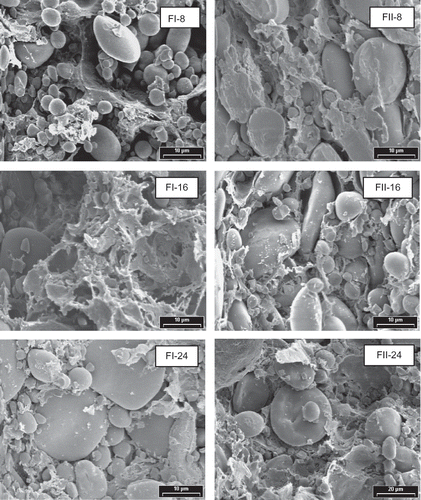
The nature of gluten matrix of dough obtained with farinograph resulted different from that obtained with the Alveograph procedure. The type of gluten matrix formed in dough depends on the mechanical work input and other experimental conditions provided during each technical procedure. Moreover, in Alveograph technique, dough is formed by mixing flour with 2% NaCl solution, thus rendering less sticky and more homogeneous dough than those obtained in the farinograph.
Viscoelasticity of Dough
Changes in elastic modulus (G') and loss tangent (tan δ = G”/G') with mixing time, are shown in . No differences were observed in both parameters between flours at 8 min of mixing. The increase in mixing time produced in FI dough an increase in G'; while for FII dough, the elastic modulus did not change with mixing time. The viscoelastic response (tan δ), was different in both type of dough. Low values of tan δ suggest more elastic samples.[Citation31] Elasticity of FI dough increased, while in FII dough did not change with the increase in mixing time. The low stability to mixing process experienced by FII dough at high deformations was not detected in the linear viscoelasticity range. The increase in G' with mixing time in strong flour like FI, was also observed by Kuktaite et al.[Citation37] The weakening of the material structure observed at high deformations, like in texture measurements where the rupture of matrix takes place, was not observed in these assays performed within the linear viscoelasticity region. Dynamic measurements are carried out at conditions very different from those experienced by the dough during processing or baking expansion.[Citation38] However these assays could be usefully related to the microstructure of dough after mixing.
Gluten and Dough Microstructure
Structure and rheological properties of dough are closely related to the content of free sulfhydryls (SHF) of dough proteins and the formation of disulfide bonds during flour mixing.[Citation39–41] shows the variation of SHF content of dough proteins with mixing time. Considering SHF values of flours (2.43 and 1.42 μmols SH/g flour, db, for FI and FII, respectively-) a drastic decrease in the content of SHF between flours and dough was observed after 8 min of mixing: SHF content in FI decreased 70%, while in FII decreased 50%. No differences in SHF values between FI and FII dough were observed. A great decrease in SHF values, especially after 16 min of mixing, was observed for both kind of dough. The lower SHF content of dough in comparison to flours indicates that mixing process enhance interchange SH/S-S reactions. Due to a greater SHF reduction, FI presented higher SH/S-S interchange than FII. A high number of S-S bonds formed during mixing would lead to the formation of a highly entanglement gluten matrix. On the other hand, overmixing can produce the cleavage of some disulfide bonds with the corresponding increase of free sulfhydryls. Skerrit et al.[Citation26] observed the cleavage of disulfide bonds in HMW glutenin during overmixing and dough breakdown.
In the present work, by the variation of content of free sulfhydryl with mixing time, it could be inferred that the behavior observed in TPA assays on over-mixed dough (24 min) should be related not to changes in the amount of S-S bonds but to the modification of their distribution. These S-S bonds could be linking different low molecular mass glutenin subunits released from GMP as a consequence of over-mixing. For FI dough, the increase in S-S bonds with mixing up to 16 min mixing is reflected in the increase in G' and the consequent decrease in tan δ observed in rheometric assays indicating an increase in dough elasticity. On the other hand, the S-S formation had no consequence on dough viscoelasticity of FII.
Gluten samples GI and GII presented similar SHF contents: 3.92 ± 0.41 and 3.68 ± 0.16 μmols SH/g gluten db. Nevertheless, these values were higher than those obtained from commercial vital gluten, 1.50 ± 0.24 μmols/g gluten db. Differences between native and commercial samples could be attributed to the thermal treatment utilized in commercial gluten preparation, which promotes protein aggregation and the consequently a decrease in free sulfhydryl content.
Different gluten structure was obtained from both flours (). GII presented a more opened structure than GI. In addition, FI presented a glutenin to gliadin ratio higher than FII.[Citation31] Different glutenin to gliadin ratio of flour seems to influence the nature of gluten matrix and consequently, rheological properties of dough. Moreover, this distinct protein proportion and/or the quantity of SDS-soluble HMW glutenin polymers will influence the response of gluten proteins to mixing time. It is probable that GI and GII gluten matrices were stabilized by different kind of glutenin polymers. An evidence of this assessment is that in denaturing and dissociating conditions, a 98 kD polypeptide could be extracted from gluten GII (). This polypeptide was not observed in the case of GI gluten. As the electrophoresis profile of both flours, FI and FII, was the same, this observation could indicate that this polypeptide was stabilizing the insoluble macro polymer in GI gluten. Nevertheless, this polypeptide was detected in both flour dough, DI and DII, after 16 min of mixing (), suggesting a contribution of other molecules to dough matrix stabilization. Gluten stabilized by different kind of protein, develop a distinct gluten network that leads to dough with distinct rheological properties.
CONCLUSIONS
Mixing at different times affects both rheological and structural characteristics of dough. Excessively long mixing times lead to softer, less consistent and stickier dough than optimum mixed ones. However, the effect of over-mixing is highly related to the type of flour. Commercial flours used in this work, having a similar electrophoresis profile, exhibited different tolerance to over-mixing. In spite of the similar content of SHF of both dough, one of them (FI) rendered a more elastic matrix, evidenced by rheometric assays. Rheometric assay is useful to characterize the viscoelastic matrix, but it does not necessarily predict the performance of flour under higher strains. When dough was submitted to compression; breakdown was detected, phenomenon related to matrix disruption as evidenced by SEM. Even though FI and FII had the same protein subunits, different type of macropolymers could be involved in the stabilization of each gluten network. Therefore, dough originated from different flours, were distinctly affected by mixing time due to breaking of disulfide bonds during depolymerization.
ACKNOWLEDGEMENTS
The authors would like to thank Consejo Nacional de Investigaciones Científicas y Técnicas (CONICET), Molinos Rio de la Plata S.A. and SECYT (PICTR 2003 N° 286) for the financial assistance received to carry out this research. Authors want to thank Ms. Viviana Sorrivas (CCT-CONICET Bahía Blanca-UAT) for the technical support in SEM analysis.
REFERENCES
- Schofield , J.D. 1986 . “ Flour proteins: structure and functionality in baked products ” . In Chemistry and Physics of Baking , Edited by: Blanshard , J.M.V. , Frazier , P.J. and Galliard , T. 14 – 19 . London : Royal Society of Chemistry .
- Shewry , P.R. and Tatham , A.S. 1997 . Disulphide bonds in wheat gluten proteins . Journal of Cereal Science , 25 : 207 – 227 .
- Belton , P.S. 1999 . On the elasticity of wheat gluten . Journal of Cereal Science , 29 : 103 – 107 .
- Graveland , A. , Bosveld , P. and Scheepstra , A. 1985 . A model for the molecular structure of the glutenin from wheat flour . Journal of Cereal Science , 3 : 1 – 16 .
- Payne , P.I. 1987 . Genetics of wheat storage proteins and the effect of allelic variation and bread-making quality . Annual Review of Plant Physiology , 38 : 141 – 153 .
- Shewry , P.R. , Pompineau , Y. , Lafiandra , D. and Belton , P. 2001 . Wheat glutenin subunits and dough elasticity: Findings of the EUROWHEAT project . Trends in Food Science and Technology , 11 : 433 – 441 .
- Peña , E. , Bernardo , A. , Soler , C. and Jouve , N. 2005 . Relationship between common wheat (Triticum aestivum L.) . gluten proteins and dough rheological properties. Euphytica , 143 : 169 – 177 .
- Mac Ritchie , F. 1987 . Evaluation of contributions from wheat protein fractions to dough mixing and breadmaking . Journal of Cereal Science , 6 : 259 – 268 .
- Branlard , G. and Dardevet , M. 1985 . Diversity of grain proteins and bread wheat quality . I. Correlation between gliadin bands and flour quality characteristics. Journal of Cereal Science , 3 : 329 – 343 .
- Huebner , F.R. and Bietz , J.A. 1986 . Assessment of the potential breadmaking quality of hard wheats by reversed-phase high-performance liquid chromatography of gliadins . Journal of Cereal Science , 4 : 379 – 388 .
- Huebner , F.R. 1989 . Assessment of the potential breadmaking quality of hard wheats by reversed-phase high-performance liquid chromatography of gliadins—Year two . Cereal Chemistry , 66 : 333 – 337 .
- Van Lonkhuijsen , H.J. , Hamer , R.J. and Schreuder , C. 1992 . Influence of specific gliadins on the breadmaking quality of wheat . Cereal Chemistry , 69 : 174 – 177 .
- Sapirstein , H.D. and Fu , B.X. 1998 . Intercultivar variation in the quality of monomeric proteins, soluble and insoluble glutenin, and residue protein in wheat flour and relationships to breadmaking quality . Cereal Chemistry , 75 : 500 – 507 .
- Janssen , A.M. , van Vliet , T. and Vereijken , J.M. 1996 . Rheological behavior of wheat glutens at small and large deformations. Comparison of two glutens differing in bread making Potential . Journal of Cereal Science , 23 : 19 – 31 .
- Lindborg , K.M. , Trägårdh , C. , Eliasson , A.Ch. and Dejmek , P. 1997 . Time-resolved shear viscosity of wheat flour doughs. Effects of mixing, shear rate, and resting on the viscosity of doughs of different flours . Cereal Chemistry , 74 : 49 – 55 .
- Martinant , J.P. , Nicolas , Y. , Bouguennec , A. , Popineau , Y. , Saulnier , L. and Branlard , G. 1998 . Relationships between mixograph parameters and indices of wheat grain quality . Journal of Cereal Science , 27 : 179 – 189 .
- Janssen , A.M. , van Vliet , T. and Vereijken , J.M. 1996 . Fundamental and empirical rheological behaviour of wheat flour doughs and composition with bread making performance . Journal of Cereal Science , 23 : 43 – 54 .
- Kuktaite , R. , Larsson , H. and Johansson , E. 2007 . “ The influence of dough mixing time on wheat protein composition and gluten quality for four commercial flour mixtures ” . In Wheat Production in Stressed Environments , Edited by: Buck , H.T. , Nisi , J.E. and Salomón , N. 543 – 548 . Netherlands : Springer .
- Faubion , J.M. , Dreese , P.C. and Diehl , K.C. 1985 . “ Dynamic rheological testing of wheat flour doughs ” . In Rheology of Wheat Products , Edited by: Faridi , H . 91 – 116 . St. Paul, MN : American Association of Cereal Chemists .
- Safari-Ardi , M. and Phan-Thein , N. 1998 . Stress relaxation and oscillatory tests to distinguish between dough prepared from wheat flours of different varietal origin . Cereal Chemistry , 75 : 80 – 84 .
- Létang , C. , Piau , M. and Verdier , C. 1999 . Characterization of wheat flour-water doughs. Part I: Rheometry and microstructure . Journal of Food Engineering , 41 : 121 – 132 .
- Schluentz , E.J. , Steffe , J.F. and Ng , P.K.W. 2000 . Rheology and microstructure of wheat dough developed with controlled deformation . Journal of Texture Studies , 31 : 41 – 54 .
- Lee , L. , Ng , P.K.W. , Whallon , J.H. and Steffe , J.F. 2001 . Relationship between rheological properties and microstructural characteristics of nondeveloped, partially developed, and developed doughs . Cereal Chemistry , 78 : 447 – 452 .
- Anjum , F.M. , Khan , M.R. , Din , A. , Saeed , M. , Pasha , I. and Arshad , A.U. 2007 . Wheat gluten: high molecular weight glutenin subunits-structure, genetics, and relation to dough elasticity . Journal of Food Science , 72 : R56 – R63 .
- Zheng , H. , Morgenstern , M.P. , Campanella , O.H. and Larsen , N.G. 2000 . Rheological properties of dough during mechanical dough development . Journal of Cereal Science , 32 : 293 – 306 .
- Skerrit , J.H. , Hac , L. and Bekes , F. 1999 . Depolymerization of the glutenin macropolymer during mixing: I. Changes in levels, molecular weight distribution, and overall composition . Cereal Chemistry , 76 : 395 – 401 .
- García , M.O. 2004 . Comercio de trigo pan. Características de la oferta y la demanda mundial , Argentina : Programa Nacional de Calidad de Trigo .
- Celentano , N. and Montero , K. 2004 . Clasificación de trigo argentino para su comercialización , Argentina : Msc. Thesis. Universidad del CEMA .
- AACC International . 2000 . “ Approved Methods of the American Association of Cereal Chemists ” . In Methods 44-15A, 8-03, 46-10, and 38-12A , 10th , St. Paul, MN : AACC International .
- IRAM . 2007 . “ Methods: 15855, 15857, 15864 ” . Argentina : Instituto Argentino de Racionalización de Materiales .
- Puppo , M.C. , Calvelo , A. and Añón , M.C. 2005 . Physicochemical and rheological characterization of wheat flour dough . Cereal Chemistry , 82 : 173 – 181 .
- Steffe , J.F. 1996 . Rheological methods in food process engineering , 418 East Lansing, MI : Freeman Press .
- Chan , K. and Wasserman , B.P. 1993 . Direct colorimetric assay of free thiol groups and disulfide bonds in suspensions of solubilized and particulate cereal proteins . Cereal Chemistry , 70 : 22 – 26 .
- Moiraghi , M. , Ribotta , P.D. , Aguirre , A. , Pérez , G.T. and León , A.E. 2005 . Análisis de la aptitud de trigos pan para la elaboración de galletitas y bizcochuelos . Agriscientia , 22 : 47 – 54 .
- Ponzio , N.R. , Puppo , M.C. and Ferrero , C. 2008 . Mixtures of two Argentinean wheat cultivars of different quality: a study on breadmaking performance . Cereal Chemistry , 85 : 579 – 585 .
- Parades-Lopez , O. and Bushuk , W. 1982 . Development and ‘undevelopment’ of wheat dough by mixing: microscopic structure and its relations to bread-making quality . Cereal Chemistry , 60 : 24 – 27 .
- Kuktaite , R. , Larsson , H. , Marttila , S. and Johansson , E. 2005 . Effect of mixing time on gluten recovered by ultracentrifugation studied by microscopy and rheological measurements . Cereal Chemistry , 82 : 375 – 384 .
- Dobraszczyka , B.J. and Morgenstern , M.P. 2003 . Rheology and the breadmaking process . Journal of Cereal Science , 38 : 229 – 245 .
- Andrews , D.C. , Caldwell , R.A. and Quail , K.J. 1995 . Shulfhydryl analysis. I. Determination of free shulfhydryls in wheat flour doughs . Cereal Chemistry , 7 : 326 – 329 .
- Andrews , D.C. , Caldwell , R.A. and Quail , K.J. 1995 . Shulfhydryl analysis. II. Free shulfhydryls content of heated doughs from two wheat cultivars and effect of potassium bromate . Cereal Chemistry , 72 : 330 – 333 .
- Anderson , A.K. and Ng , P.K.W. 2000 . Changes in disulfide and sulfhydryl contents and electrophoretic patterns of extruded wheat flour proteins . Cereal Chemistry , 77 : 354 – 359 .