Abstract
Several experiments were conducted at different storage temperatures for generating respiration data using close system method for respiration. A respiration rate model, based on enzyme kinetics and the Arrhenius equation was proposed for predicting the respiration rates of Guava as a function of O2 and CO2 concentrations and storage temperature. Temperature was found to influence the model parameters. In this model, the dependence of respiration rate on O2 and CO2 was found to follow the uncompetitive inhibition. The enzyme kinetic model parameters, calculated from the respiration rate at different O2 and CO2 concentration were used to fit the Arrhenius equation against different storage temperature. The activation energy and respiration pre-exponential factor were used to predict the model parameters of enzyme kinetics at any storage temperature between 0–30°C. The developed models were tested for its validity at 12°C and it was found to be in good agreement (the mean relative deviation moduli between the predicted and experimental respiration rates were found to be 8.95% and 8.02% for O2 consumption and CO2 evolution, respectively) with the experimentally estimated respiration rates.
INTRODUCTION
Guava (Psidium guajava L.) is an important tropical fruits and is native to the tropical America stretching from Mexico to Peru.[Citation1,Citation2] Guava claims superiority over other fruits by virtue of its commercial and nutritional values. It is considered as common man's fruits and is rightly called as the apple of the tropics.[Citation1] Although the guava plant was domesticated more than 2000 years ago, it was not until 1526 when the first commercial cultivation of guava was reported in the Caribbean islands. Later it was spread by explorers into the Philippines and India. From there it quickly spread to most of the tropical and subtropical regions of the world.Citation[3] It has been adopted in India so well that it appears to be an Indian fruit.[Citation2] The world production of Guava is estimated to be 5.0 MT. India leads the world in Guava production with annual production of 1.80 MT with the production area of 0.15 Mha.Citation[4] Guava is the fourth most widely grown fruit crop in India. The popular varieties of guava grown in India are Allahabad Safeda, Lucknoe-49, Baraipur, Nagpur Seedless, Dharwar, etc.[Citation4] In India, Bihar is the leading state in guava production with 0.30 MT followed by Andhra Pradesh, and Uttar Pradesh. The other states in India, where guava is widely grown are Gujarat, Karnataka, Punjab, and Tamil Nadu.
Guava is an important fruit crop of India and has gained considerable importance because of its high nutritive value, availability at moderate price, a pleasant aroma, good flavor, delicious taste, and remunerative nature of crops. Guava is one of the most common fruit liked by both the rich and the poor.Citation[5] Guava fruits consist of about 20% peel, 50% fleshy portion, and 30% seed core. The physicochemical characteristics of fruits change significantly with maturity.[Citation6,Citation7] Guava fruit usually contains 74–87% water, 13–26% dry matter, 0.8–1.5% protein, 0.4–0.7% fat, 2–7.2% crude fiber, 2.45% acid, 4.45% reducing sugars, 5.23% non-reducing sugars, 9.73 °brix, 0.5–1.0% ash and 260 mg vitamin C/100 g fruit. The compositions differ with the cultivar, stage of maturity and season.[Citation1,Citation5,Citation8] Ripe guava fruit is an excellent source of ascorbic acid (vitamin C), dietary fiber and pectin. Guava is also a good source of vitamin A, phosphorus, calcium and iron as well as thiamine, niacin, riboflavin and carotene.[Citation3] The total pectin content of Guava fruit is in the range of 0.5–1.8%. Reportedly, consumption of guava fruit reduces serum cholesterol levels, triglycerides and hypertension while increasing the level of good cholesterol (high density lipoprotein).Citation[9] Both ripe and green fruits, the root, leaves and bark are used throughout the guava growing region in local medicine for treating gastroenteritis, diarrhea and dysentery.[Citation3,Citation10,Citation11] The fruit, exuding a strong, sweet, musky odor when ripe, may be round, ovate, or pear-shaped, 4–10 cm long, with 4 or 5 protruding floral remnants (sepals) at the apex; and thin, light-yellow skin, frequently blushed with pink. Next to the skin is a layer of somewhat granular flesh, 3–12.5-mm thick, white, yellowish, light- or dark-pink, or near-red, juicy, acid, subacid, or sweet and flavorful. The central pulp, concolorous or slightly darker in tone, is juicy and normally filled with very hard, yellowish seeds, 3-mm long, though some rare types have soft, chewable seeds. The average diameter of fruit varies from 4–8 cm and the weight may range from 50–500 g. Actual seed counts have ranged from 112 to 535 but some guavas are seedless or very limited in numbers.[Citation9,Citation10,Citation12]
The fruit is relished when mature or ripe and freshly plucked from the tree. Guava fruits are used for both, fresh consumption and processing. It is eaten as fresh fruit when ripe, used in sauce and chutney, or cooked as a vegetable when green. It is also processed into a variety of products such as jam, jelly, cheese, toffee, juice, squash, wine, dried fruits, canned fruits, as well as flavoring for other foods.[Citation1,Citation10,Citation12] It excels most other fruit trees in productivity, hardiness, adaptability and vitamin-C content. Besides its high nutritive value, it bears heavy crop every year and gives good economic returns involving very little input.[Citation12] This has prompted several farmers to take up guava cultivation on a commercial scale.
The main objective of storage of perishable commodities such as guava is to prolong their availability on the market throughout year. Certain post harvest constraints like short shelf life, chilling sensitivity, and susceptibility to diseases limit its long duration storage and transportation. Many researchers have studied the influence of altered atmosphere on the commodity storage life. Two main types of altered atmosphere are modified and controlled atmosphere (MA and CA). The use of these methods provide some control of fruit and vegetable ripening, retardation of senescence, or browning in cut produce[Citation13,Citation14] and ultimately prolongs the shelf life. Depending on the specific requirements, Modified Atmosphere (MA) and Control Atmosphere (CA) can prolong market availability of the produce up to the next harvest.
Storage operations can be classified with respect to construction as O2 control systems, CO2 control systems, temperature and relative humidity control systems, ethanol vapor generators, and ethylene absorbent.[Citation15,Citation16] In CA storage, the atmosphere is modified and its composition is precisely adjusted according to the specific requirements throughout the storage period.[Citation17–19] Modified atmosphere packaging (MAP) of fresh produce relies on the modification of the atmosphere inside the package, achieved by the natural interplay between the respiration of the product and the transfer of the gases through the packaging. This atmosphere can potentially reduce respiration rates, ethylene sensitivity and production, decay and physiological changes, namely oxidation.[Citation20,Citation21] MAP is used when the composition of the atmosphere is not closely controlled, whereas CA monitors and controls the gaseous composition more precisely. Hence, the need was realized on the post harvest management of guava fruit in medium/hi-tech way like CA and MA storage. However, accurate measurement of respiration rates and modeling is pivotal to the design of MA/CA for agricultural commodity.[Citation17,Citation20,Citation22]
Respiration is a metabolic process that provides the energy for plant biochemical processes. Various substrates used in important synthetic metabolic pathways in the plant are formed during respiration.Citation[23] Aerobic respiration consists of oxidative breakdown of organic reserves to simpler molecules, including carbon dioxide (CO2) and water, with release of energy. The organic substrates broken down in this process may include carbohydrates, lipids, and organic acids. The process consumes oxygen (O2) in a series of enzymatic reactions. Due to these metabolic processes in fruits, which continue even after harvest, the shelf life of the product is reduced. However, the rates of these metabolic processes are generally directly proportional to the storage temperature, thus increasing the shelf life to a certain level at lower temperatures.
Measurement of respiration rate of produce at a particular storage temperature and gas compositions is time consuming and needs special equipments for gas analysis. Various mathematical models have been developed to correlate the respiration rate with different storage parameters such as gas composition, i.e., O2 and CO2 and temperature. Yang and ChinnanCitation[24] used a quadratic function to correlate the respiration rates of tomato with O2 and CO2 concentrations and storage time. The effect of temperature on respiration rate was not considered in their models. The models adequately predicted the respiration rate of tomatoes stored in a controlled atmosphere. Lee et al.Citation[25] suggested an enzyme kinetics theory for modeling of respiration rate. Cameron et al.Citation[26] used an empirical approach to measure the respiration rate as a function of O2 concentration by observing the rate of O2 depletion in a closed system. Talasila et al.Citation[27] developed a non-linear empirical model for predicting the respiration rate of strawberry as a function of temperature, O2 and CO2 concentration. Most of the model have not incorporated one or other dependent factors such as O2, CO2, temperature and time, and hence, are not flexible enough to predict the respiration rate at various storage conditions.
The recent approach for modeling respiration rates by using Michaelis-Menten type equation is based on enzyme kinetics.[Citation25] This provides a simple description of respiration, based on the assumption that diffusion and solubility of O2 and CO2 in plant tissue regulates reactions catalyzed by enzymes. For agricultural commodity, both O2 and CO2 concentrations have an influence on quality and shelf life. At high CO2 concentrations, quality loss could be reduced for products such as broccoli,Citation[28] pearCitation[29] and mushrooms.Citation[30] High carbon dioxide concentrations can reduce the oxygen consumption rate of a number of fruits and vegetables. The role of CO2 in respiration is suggested to be mediated via inhibition mechanism of the Michaelis-Menen equations. This can be modeled by four types of inhibition in an enzyme kinetics model: (1) the competitive type; (2) the uncompetitive type; (3) a combination of competitive and uncompetitive types; and (4) the non-competitive type. All these models describe the inhibitory effects of CO2 on respiration rate.Citation[31] These inhibition models were tested for describing the CO2 influence on O2 consumption using experimental data supplemented with data from literature.[Citation31] They found the clear influence of high CO2 on the gas exchange rates of some produce and estimated the O2 consumption rate using the inhibition models. This supports the use of Michaelis-Menten kinetics for modeling O2 consumption and CO2 evolution rate. Peppelenbos and Leven[Citation31] suggested the simultaneous existence of both competitive and uncompetitive type of inhibition. However, for reasons of simplicity they preferred the non-competitive type of inhibition.
McLaughlin and O'Beirne[Citation32] described the effect of CO2 on dry coleslaw mix respiration rate in three ways: competitive inhibition, uncompetitive inhibition and non-competitive inhibition. The uncompetitive inhibition model fitted best with the experimental results. But the model was found to be inflexible to include temperature as the independent parameter. However, the Arrhenius equation describes the dependence of the respiration rate on temperature.[Citation17,Citation33–35] have predicted the respiration rate of some commodities using principles of enzyme kinetics with uncompetitive inhibition. However, due to the specificity of the model parameters, the same need to be identified and quantified for each fruit and vegetable product. Hence, the present work was undertaken to develop and verify a combined respiration rate model based on the principle of enzyme kinetics, for dependence of O2 and CO2 and based on Arrhenius equation for dependence of storage temperature.
THEORETICAL CONSIDERATIONS
Enzyme Kinetics
The principles of enzyme kinetics have been suggested as it is being applicable to the respiration rate of fresh produce and Michaelies-Menten equation has been fitted to respiration rates.[Citation25] Considering that CO2 acts as a respiration inhibitor, the effect of CO2 on the product respiration can be described by the un-competitive inhibition.[Citation31,Citation32,Citation36] The uncompetitive type of inhibition occurs where the inhibitor (CO2) does not bind with the enzyme, but binds reversely with the enzyme-substrate complex, reducing the rate of product formation. In this case the increase of substrate concentration (O2 concentration) at high CO2 concentrations has almost no influence on the O2 consumption rate, i.e., increase in the substrate concentration cannot reverse it.Citation[37] Thus the maximum respiration rate is not much influenced at high CO2 concentration. At high levels of CO2 concentration (17–18%), however the respiration mechanism changes form aerobic to anaerobic pathway.[Citation17] Hence principles of enzyme kinetics with uncompetitive inhibition were used to develop a model[Citation25,Citation31,Citation33,Citation38–41] for predicting the respiration rate of products. EquationEquations (1) and Equation(2) express the uncompetitive inhibition mechanisms for respiration process in terms of O2 consumption and CO2 evolution rate respectively. The model has three parameters viz., , and
for both O2 consumption and CO2 evolution. The model parameters are determined using the experimental respiration data.
where is the respiration rate, ml [O2] kg−1h−1;
is the respiration rate, ml [CO2] kg−1h−1,
, and
are the gas concentrations for O2 and CO2 respectively in percentage;
and
are the maximum respiration rate for O2 consumption; and CO2 evolution respectively,
and
are the Michaelis-Menten constant for O2 consumption and CO2 evolution,% O2, respectively; and
and
are the inhibition constants for O2 consumption, CO2 evolution,% CO2, respectively.
The parameters of EquationEqs. (1) and Equation(2) may be estimated by linearization of the equation and subsequent linear regression analysis[Citation25,Citation32,Citation34,Citation35,Citation38–43] or directly by non-linear regression analysis.[Citation31,Citation44–48] However linearizing the equations is equivalent to changing the weight given to the data in the estimation procedure and thus should be avoided.[Citation20,Citation31]
Arrhenius Equations
Temperature is the most important environmental factor in the post harvest life of fresh produce because of its dramatic effect on rates of biological reaction including respiration. Within the physiological range (0 to 30°C), the respiration rate of fresh fruits and vegetables generally increases two to three folds for every 10°C rise in temperature.Citation[49] The Arrhenius relationship describes the dependence of the biological reactions, including respiration of fresh produce, on temperature. The activated complex theory for chemical rates is the basis for the Arrhenius equation, which relates reaction rates to the absolute temperature.Citation[50] Temperature has also major effect on the respiration rate, which has not been considered in the equation of the uncompetitive inhibition of enzyme kinetics (EquationEqs. 10 and Equation11). As per the studies conducted by Bhande et al.,[Citation34] Das,[Citation35] Hong et al.,Citation[51] and Lakakul et al.Citation[52] on banana, sapota fruit, fresh-cut green onion, and sapota slices respectively, the model parameter of Michaelis-Menten type enzyme kinetics was found to vary with the storage temperature. They used Arrhenius plot to describe the relationship between model parameters and storage temperature. The dependence of model parameters of uncompetitive inhibition kinetics on storage temperature can be expressed by EquationEq. (3).[Citation52]
where, Rm is the model parameter of enzyme kinetics; Rp is the respiration pre-exponential factor; Ea is the activation energy, kJ/g mole; Tabs is the storage temperature; and K and R is the Universal gas constant (8.314 kJ/kg mole K). The EquationEq. (3) can be expressed in a linearized formCitation[53] as shown in EquationEq. (4):
The model parameters obtained previously would be used to fit in EquationEq. (4) at different storage temperatures to estimates the values of Ea and Rp.
MATERIALS AND METHODS
Fruit Material
Fresh mature Guava fruit (Psidium guajava L.) of local popular variety Baraipur local were obtained from the orchard. Fruits were washed to remove adhering dirt, and used for the study. Care was taken to ensure that the fruits were of uniform size and weight.
Physicochemical Properties of Fruits
The physicochemical properties were determined in the laboratory to specify the maturity level of fruits prior to the respiration study. Initial fruit color and firmness was determined using Hunter Lab Colorimeter and Texture analyzer respectively.[Citation17,Citation54] The weight of guava fruits was measured using a precision balance (Essae Teraoka, Japan) having an accuracy of 0.01 g. True volume, ascorbic acid, total soluble solids and titrable acidity were determined as suggested by Ranganna[Citation55] and Gungor and Sengul.[Citation56] All the physical and chemical properties were determined for 10 representative samples and the average values are presented in .
Table 1 Physicochemical properties of guava fruits cv. Baruipur
Closed System Respirometer for Gas Exchange Measurement
Measurement of O2 consumption by the fruit tissue as a function of O2 and CO2 concentration in a flow through system is technically difficult, since it requires highly accurate analytical equipment.[Citation26] Hence, the respiration rate data for guava fruits was experimentally generated for different temperatures using the closed system method[Citation31,Citation33–35,Citation41,Citation42] as described in . Closed systems can provide a convenient way of characterizing respiration of fresh produce in a single set of experiment.[Citation20,Citation38,Citation41,Citation51,Citation57] In this process, the changes in O2 and CO2 concentrations resulting from respiration within a sealed container could be measured directly. Air-tight respirometer chambers of size 0.125 m × 0.175 m × 0.23 m made up of acrylic (Perspex) sheet was used. A Ni-Cr thermocouple was inserted into one side of the respirometer and used to measure the storage temperature. Fruits were kept in respirometer from the open topside and were closed with the lid while inserting neoprene gasket in between. Lid was closed with nuts and bolts provided on the respirometer to make it airtight. The system was kept in a humidity control chamber (Digitek System, Kolkata, India) which was maintained at the desired temperature with a tolerance limit of ±0.2°C and relative humidity of 92 ± 2%. Gas composition of the respirometer was analyzed at regular intervals depending on the storage temperature of guava. Typically, the interval chosen were 2 h for the temperatures at 30 and 25°C, 4 h at 20 and 15°C, 8 h at 10, 5 and 0°C, respectively. Gas analysis was done until the CO2 concentration reached 18% and until aerobic respiration persisted.[Citation38] Changes in the concentration of O2 and CO2 over a certain period of time were measured and used to estimate respiration rates. After an interval of time, gas samples of the container were analyzed for O2 and CO2 concentration. Weight of fruits taken during the experiment and its corresponding free volume is shown in . Free volume of the respirometer was the total volume of the respirometer minus volume occupied by its content. The free volume of the respiration chamber () was determined by nitrogen injection method.[Citation58] For this a predetermined quantity (QN) of N2, which could cause a measurable change in N2 level of container's atmosphere was injected. The increase in N2 level was determined by analyzing the gas sample on gas chromatograph. By incorporating these values in EquationEq. (5), the free volume of the respiration container was calculated:
Table 2 Free volume of respirometer and weight of guava taken for generating the respiration data
where, Vf is the free volume of the respiration chamber in cm3; QN is the volume of N2 injected into the respiration chamber in cm3; and Ni and Nf are the initial and final N2 concentration in%.
Gas Analysis
Storage gas sample of about 1 ml was taken periodically with an airtight syringe and was analyzed quantitatively for O2 and CO2 concentrations using gas chromatograph (GC model 100, Knaur, Germany). A column packed with Spherocarb (18–100 mesh, 1.83-m long) was used for separation of CO2, O2 and nitrogen. Hydrogen was used as carrier gas at a flow rate of 25 ml/min. Oven temperature was kept at 50°C whereas injector and detector were set at 125 and 150°C, respectively, with the detector voltage of 5 volt. The sampling intervals were different for different storage temperatures as mentioned in the previous section. Each time three replications were taken for the gas analysis and the average gas composition was recorded. The respiration study was replicated in triplicate for accuracy of measurement and analysis.
Measurement of Respiration rate
Respiration rates can be measured by observing the concentration of O2 consumption or CO2 evolution per unit time per unit weight of the produce. Various external factors such as O2 concentration, CO2 concentration, temperature and time affect respiration.[Citation17,Citation25,Citation38,Citation58,Citation59] The experimental respiration rate was calculated from the concentration difference, weight of fruit and free volume of the chamber. The respiration rates in terms of O2 consumption and CO2 evolution at a given temperature are calculated using the following EquationEqs. (6) and Equation(7) as given by Kays (1991).[Citation59]
where: RO2 is the respiration rate, ml [O2] kg−1h−1, RCO2 is the respiration rate, ml [CO2] kg−1h−1, and
are the gas concentrations for O2 and CO2, respectively, t is the storage time in h, Δt is the time difference between two gas measurements,
is the free volume of the respiration chamber in ml and W is the weight of the fruit in kg.
Verification of the Model
Since the respiration rates were generated at temperature between 0°C to 30°C with a step of 5°C, it is necessary to verify whether the developed models are capable of predicting the respiration rates at any temperature within this domain of experimental temperatures. The experimental respiration data for O2 and CO2 were obtained by the closed system respirometer kept at 12°C. Free volume of respirometer and weight of guava taken for verification of the model were 4852.37 ml and 1.348 kg, respectively. The experimental respiration rates for guava at different combinations of O2 and CO2 at 12°C temperature were determined using EquationEqs. (6) and Equation(7), respectively.
Statistical Analysis
The standard deviations of the physicochemical properties were estimated using standard statistical formulae.[Citation60] The values obtained are reported in . The SYSTAT 8.0 software was used to estimate the model parameters of enzyme kinetics model along with their coefficients of determination. The one way ANOVA was carried out to ascertain the effect of temperature on model parameters and effect of temperature on regression coefficients using SYSTAT 8.0 with completely randomized block design. The mean relative percentage deviation modulus is widely adopted throughout the literature to evaluate the goodness of fit of mathematical expression. Moduli below 10% are indicative of reasonably good fit, 10–20% fairly good fit and 20–30% not satisfactory fit for all practical purposes. The goodness of fit between predicted and experimental respiration rates was obtained by calculating the mean relative percentage deviation modulus.[Citation32] In general, lower the modulus better is the agreement between experimental and predicted values.
RESULTS AND DISCUSSION
Assessment of Maturity Level
The average unit weight and volume were found to be 250.38 g and 228.15 ml respectively (). The L*, a* and b* color values were measured to be 57.83, −17.58 and 39.41, respectively. The negative value of ‘a’ indicates the greenness of guava fruits at maturity. The flesh firmness, puncture strength, and TSS were 9527.40 g, 759.34 g, and 8.5, respectively indicating that the fruits were hard and matured. The results obtained were found to be in agreement with those reported by Adsule and Kadam,[Citation1] Palaniswamy and Shanmugavelu,[Citation8] Morton,[Citation9] and Wilson.[Citation12]
Gas Exchange Rate
Figure 2 shows the periodical changes in O2 and CO2 concentrations inside the closed respirometer containing guava at different storage temperatures. The O2 concentration of the respirometer decreased in proportion to increase in CO2 concentration with the storage period, the rate being higher at the higher storage temperature.
Modeling and Analysis
A regression function is often used to fit the data of gas concentration versus time, and the respiration rate at given time is determined from the first derivative of the regression function.[Citation26,Citation38,Citation40] shows the actual gas composition in terms of O2 and CO2 concentrations inside the closed respirometer at different storage temperature. By using the generated experimental respiration data, a nonlinear regression analysis (Origin 6.1) was done to fit the curve of O2 concentration and CO2 concentration at different storage periods. The resultant regression equations for O2 consumption and CO2 evolution are shown in EquationEqs. (8) and Equation(9) to determine the values of the coefficients ‘a’ and ‘b’:
Figure 2 Experimental data for O2 consumption and CO2 evolution at different temperatures; ▴ is YO2, in%; ▪ is ZCO2, in%.
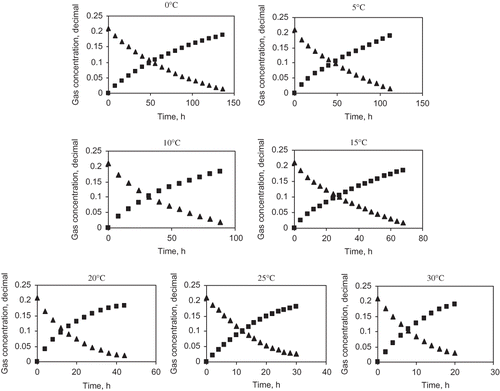
where a and b are the regression coefficients; a is unit less and b is in h; t is storage period in h, and
are the gas concentrations for O2 and CO2, respectively in decimal. The first derivative of the regression functions were used to determine the rate of change of gas concentration as outlined in EquationEqs. (10) and Equation(11):
The respiration rate at any given time (at each sampling time) was then calculated by substituting the values of dYO2 /dt and dZCO2 /dt obtained from EquationEqs. (10) and Equation(11) in EquationEqs. (12) and Equation(13), respectively.
The respiration rate was found to have decreased with time due to decreasing O2 concentrations and increasing CO2 concentrations. The regression coefficients a, and b of EquationEqs. (8) and Equation(9), and correlation coefficients (r2) at different storage temperatures are shown in . The above regression functions described the experimental data very well (r2 ≥ 0. 997) for guava. From the values of the regression coefficients a and b in the , it can be inferred that both the parameters were influenced by the storage temperature and that coefficient ‘b’ was more influenced by temperature than coefficient ‘a’. The analysis of variance of temperature with regression coefficients a and b is given in . It can be inferred that the temperature has a significant effect (1% level of significance) on regression coefficients. For any unknown temperature, the values of a and b can be determined by linear interpolation of the known values. The corresponding values of a and b when substituted in EquationEqs. (10) and Equation(11), allow to estimate dYO2 /dt and dZCO2 /dt, respectively. The respiration rates for the given temperature can then be determined using EquationEqs. (12) and Equation(13).
Table 3 Values of regression coefficients for regression model
Table 4 Analysis of variance of effect of temperature on regression coefficients
The verification of the regression model for respiration rate was done at 12°C storage temperature. The values of the regression coefficient a and b at 12°C were estimated by the linear interpolation of the values of the same coefficients at 10 and 15°C as shown in . The above values of a and b are substituted in EquationEqs. (8) and Equation(9) to obtain the concentration of O2 and CO2 at each sampling time. Then the values of dYO2/dt and dZCO2/dt were obtained from EquationEqs. (10) and Equation(11). The respiration rate at each sampling time was then calculated by substituting the values of dYO2 /dt and dZCO2 /dt in EquationEqs. (12) and Equation(13), respectively. The experimental respiration rates were also obtained using EquationEqs. (6) and Equation(7). The goodness of fit was obtained by calculating the mean relative percentage deviation modulus.
The mean relative percentage deviation modulus was found to be 1.68 and 2.49% for O2 consumption and CO2 evolution, respectively. This suggests that the respiration rates predicted by the regression model were in good agreement with the experimental respiration rates data at different storage periods. Similarly the respiration rate at any storage temperature can be obtained by employing regression model, EquationEqs. (12) and Equation(13).
Estimation of Model Parameters
The model parameters of EquationEqs. (1) and Equation(2) were determined by fitting the experimental data at each temperature using non-linear regression analysis (SYSTAT 8.0, SPSS). According to EquationEqs. (1) and Equation(2), respiration rate was considered as the dependent variable whereas the percent O2 concentration () and percent CO2 concentration were considered as the independent variables for the regression analysis. The model parameters were calculated from the constants of non-linear regression analysis, which are given in along with their corresponding coefficients of determination (R2) values. With the help of these model parameters, EquationEqs. (1) and Equation(2) could now predict the respiration rates with any combination of O2 and CO2 concentration. The values in show that the model parameters were indeed dependent on the storage temperature. The coefficients of determination was found to be higher or equal to 0.975 indicating that the relationship between respiration rate and O2 and CO2 concentrations fitted well with the uncompetitive inhibition enzyme kinetics model.
Table 5 Model parameters for uncompetitive inhibition enzyme kinetics for different storage temperatures
The analysis of variance of temperature with model parameters of enzyme kinetics based model is given in . It can be inferred that the temperature has a significant effect (1% level of significance) on model parameters ,
,
,
,
, and
of enzyme kinetics.
Table 6 Analysis of variance of effect of temperature on model parameters of Enzyme kinetics model
Arrhenius Equation
Since the model parameters such as ,
, and
for O2 and CO2 concentrations were found to vary with temperature and hence, Arrhenius equation was used to correlate the model parameters at different storage temperatures. As per EquationEq. (4), the model parameters were plotted at different storage temperatures; by plotting the log values of the model parameters against the inverse of corresponding temperature in absolute units. The resulting linear plot is shown as . The slope and the Y-axis intercept of EquationEq. (4) for model parameters
,
, and
are shown in . The activation energy was calculated from the slope of the straight line and the pre-exponential factor was calculated from the Y-axis intercept. shows the activation energy and pre-exponential factor for different model parameters of enzyme kinetics. Activation energy was found to be in negative side for
and
valued at −17.54 and −16.40 kJ/g-mol, respectively. The negative values of activation energies for
could be attributed to the inhibitory effect of CO2 concentration on respiration rate. By using these constants (values of activation energy and respiration pre exponential factor), the model parameters of enzyme kinetics at any temperatures can be predicted by using EquationEq. (3) and then, the respiration rate at the given temperature can be estimated by employing EquationEqs. (1) and Equation(2), in terms of O2 consumption and CO2 evolution, respectively.
Table 7 Slope (−Ea/R) and Y axis intercept (ln Rp) of Arrhenius relation for different model parameters of enzyme kinetics
Table 8 Activation energy and pre-exponential factor of Arrhenius type equation for different model parameters of uncompetitive inhibition
Verification of Respiration Rate Model
The respiration rate predicted by the enzyme kinetics model was verified with the experimental respiration rate at 12°C storage temperature. By using activation energy and pre-exponential factor from , the model parameters at 12°C for both O2 consumption and CO2 evolution were calculated and are shown in . Then the respiration rates of guava in terms of O2 consumption and CO2 evolution were estimated by using EquationEqs. (1)and Equation(2). The experimental respiration rates of guava were obtained by the closed system method. The predicted and actual respiration rates of guava are shown in . The mean relative deviation moduli between predicted and experimental respiration rates of guava at 12°C were found to be 8.95% and 8.02% for O2 consumption and CO2 evolution respectively. This indicates that the predicted respiration rates for guava were in good agreement with the experimental respiration rates.
Table 9 Model parameters of uncompetitive inhibition enzyme kinetics at 12°C storage temperature
CONCLUSIONS
The respiration rates were found to decrease with storage time due to diminishing concentrations of O2 concentration and increase in CO2 concentrations in the respirometer. In the enzyme kinetics model, the dependence of respiration rate on O2 and CO2 was found to follow the uncompetitive inhibition. Because of the inflexibility of the model to incorporate the temperature terms, a new model was proposed in which the model parameters were correlated with storage temperature as per the Arrhenius equations. The activation energy and pre-exponential factors of the Arrhenius equations were used for predicting the model parameters at any temperature between 0–30°C. The applicability of the model for the prediction of respiration rate was verified at 12°C storage temperature. There was a good agreement between the predicted and experimental respiration rates for O2 consumption and CO2 evolution.
Nomenclature | ||
| = |
Regression coefficient |
| = |
Regression coefficient, h |
E | = |
Mean relative deviation modulus,% |
Ea | = |
Activation energy, kJ g−1mol−1 |
| = |
Michaelis-Menten constant for O2 consumption,% O2 |
| = |
Michaelis-Menten constant for CO2 evolution,% O2 |
| = |
Inhibition constants for O2 consumption,% CO2 |
| = |
Inhibition constants for CO2 evolution,% CO2 |
| = |
Number of respiration data points |
Ni | = |
Initial N2 concentration of the respirometer,%. |
Nf | = |
Final N2 concentration of the respirometer,%. |
QN | = |
Volume of N2 injected into the respiration chamber, cm3 |
R | = |
Universal gas constant, 8.314 kJ kg−1mol−1K−1 |
| = |
Respiration rate, ml [CO2] kg−1h−1 |
Rexp | = |
Experimental respiration rate, ml kg−1 h−1 |
Rm | = |
Model parameter of enzyme kinetic |
Rpre | = |
Predicted respiration rate, ml kg−1 h−1 |
| = |
Respiration rate, ml [O2] kg−1h−1 |
Rp | = |
Respiration pre-exponential factor |
T | = |
Storage temperature, °C |
Tabs | = |
Storage temperature, K |
| = |
Storage time, h |
| = |
Time difference between two gas measurements |
| = |
Free volume of the respiration chamber, ml |
| = |
Maximum respiration rate for CO2 evolution, ml/kg-h |
| = |
Maximum respiration rate for O2 consumption, ml/kg-h |
| = |
Weight of guava fruit, kg |
| = |
Oxygen concentration, decimal |
| = |
Carbon dioxide concentration, decimal |
References
- Adsule , R.N. and Kadam , S.S. 1995 . “ Guava ” . In Handbook of Fruit Science and Technology , Edited by: Salunkhe , D.D. and Kadam , S.S. 419 – 434 . New York : Marcel Dekker Inc .
- http://wwwchem.uwimona.edu.jm/lectures/guava.html (http://wwwchem.uwimona.edu.jm/lectures/guava.html)
- http://www.ag.fvsu.edu/Publicat/CommoditySheet/fvsu003.htm (http://www.ag.fvsu.edu/Publicat/CommoditySheet/fvsu003.htm)
- http://agricoop.nic.in/hort/hortevo5.htm (http://agricoop.nic.in/hort/hortevo5.htm)
- http://pnbkrishi.com/guava.htm (http://pnbkrishi.com/guava.htm)
- Mukherjee , S.K. and Datta , M.N. 1967 . Physico-chemical changes in Indian Guava during fruit development . Current Science , 36 : 671 – 677 .
- Teotia , S.S. , Tripathy , R.S. and Bhan , S. 1970 . Evaluation of indices for determination of maturity of Guava (Psidium guajava L.) . Horticulture Advances. , 7 : 97 – 103 .
- Palaniswamy , K.P. and Shanmugavelu . 1974 . Physico-chemical characteristics of some guava varieties . South Indian Horticulture , 22 : 5 – 10 .
- Morton , J. 1987 . “ Guava ” . In Fruits of warm climates Edited by: Morton , J.F. 356 – 363 . Miami, FL
- Singh , M. 1988 . Performance of some cultivars of Guava (Psidium guajava) with special reference to their commercial significance in the central Genetic plains . Punjab Horticultural Journal , 32 ( 5 ) : 28 – 50 .
- Singh , S.P. and Pal , R.K. 2008 . Controlled atmosphere storage of guava (Psidium guajava L.) fruit . Postharvest Biology and Technology , 47 : 296 – 306 .
- Wilson , W.C. and Shaw , P.E. 1980 . “ Guava ” . In Tropical and subtropical fruits , Edited by: Nagy , S. 142 – 167 . Westport, CT : AVI .
- Stiles , M.E. and Stiles , M.E. 1991 . “ Scientific principles of controlled/modified atmosphere packaging ” . In Modified Atmosphere Packaging of Food , Edited by: Ooraikul , B. 18 – 25 . New York, NY : Ellis Horwood Limited .
- Rennie , T.J. , Vigneault , C. , DeEll , J.R. and Raghavan , G.S.V. 2003 . “ Cooling and storage ” . In Handbook of Postharvest Technology , Edited by: Chakraverty , A., A.S. and Mujumdar , G.S.V. 505 – 538 . New York : Marcel Dekker Inc .
- Raghavan , G.S.V. and Gariepy , Y. 1985 . Structure and instrumentation aspects of storage systems . Acta Horticulturae , 157 : 5 – 30 .
- Parry , R.T. 1993 . Principles and Applications of Modified Atmosphere Packaging of Foods , 1 – 132 . London, , England : Blackie Academic & Professional .
- Mahajan , P.V. 2001 . Studies on Control atmosphere storage for apple and litchi using liquid nitrogen , Kharagpur, , India : Unpublished Ph.D. Thesis, Department of Agriculture and Food Engineering, Indian Institute of Technology .
- Ryall , A.L and Pentzer , W.T. 1982 . Handling, transportation, and storage of fruits and vegetables , 2nd , Vol. 2 , 610 Westport, CT : The AVI Publishing company, Inc. .
- Deshpande , S.D. and Shukla , B.D. 1995 . Modified atmosphere packaging of fruits and vegetables . Session 4–402, Phala Samskarana , 27 ( 8 ) : 169 – 171 .
- Fonseca , C. , Oliveira , F.A.R. and Brecht , J.K. 2002 . Modelling of respiration rate of fresh fruits and vegetables for modified atmosphere packages: a review . Journal of Food Engineering , 52 : 99 – 119 .
- Zagory , D. and Kader , A.A. 1988 . Modified atmosphere packaging of fresh produce . Food Technology , 42 ( 9 ) : 70 – 77 .
- Kader , A.A. , Zagory , D. and Kerbel , E.L. 1989 . Modified atmosphere packaging of fruits and vegetables . Critical Reviews in Food Science and Nutrition , 28 : 1 – 30 .
- Meyer , B.S. , Anderson , D.B. , Bohling , R.H. and Fratianne , D.G. 1973 . Introduction to plant physiology , 2nd , 326 Princeton, NJ : Van Nostrand .
- Yang , C.C. and Chinnan , M.S. 1988 . Modeling the effect of O2 and CO2 on respiration and quality of stored tomatoes . Transactions of the ASAE , 30 ( 3 ) : 920 – 925 .
- Lee , D.S. , Hagger , P.E. , Lee , J. and Yam , K.L. 1991 . Model for fresh produce respiration in modified atmosphere based on principles of enzyme kinetics . Journal of Food Science , 56 ( 6 ) : 1580 – 1585 .
- Cameron , A.C. , Boylan-Pett , W. and Lee , J. 1989 . Design of modified atmosphere packaging systems: modeling oxygen concentrations within sealed packages of tomato fruits . Journal of Food Science , 54 ( 6 ) : 1413 – 1415 . 1421
- Talasila , P. C. , Chau , K. V. and Brecht , J. K. 1992 . Effects of gas concentrations and temperature on O2 consumption of strawberries . Transactions of the ASAE , 35 ( 1 ) : 221 – 224 .
- Lebermann , K. W. , Nelson , A. I. and Steinberg , M. P. 1968 . Postharvest changes of broccoli stored in modified atmospheres. 1. Respiration of shoots and color of flower heads . Food Technology , 22 ( 4 ) : 487 – 490 .
- Ke , D. , van Gorsel , H. and Kader , A.A. 1990 . Physiological and quality responses of ‘Bartlett’ pears to reduced O2 and enhanced CO2 levels and storage temperature . Journal of American Society of Horticulture Science , 115 : 435 – 439 .
- Peppelenbos , H.W. , van't Leven , J. , van Zwol , B.H. and Tijskens , L.M.M. The influence of O2 and CO2 on the quality of fresh mushrooms . Proceedings of the 6th international controlled atmosphere research conference . Edited by: Blanpied , G. D. , Barstch , J. A. and Hicks , J. R. Vol. 1 , pp. 746 – 758 . Ithaca, NY
- Peppelenbos , H.W. and Van't Leven , J. 1996 . Evaluation of four types of inhibition for modeling the influence of carbon dioxide on oxygen consumption of fruits and vegetables . Postharvest Biology and Technology , 7 : 27 – 40 .
- McLaughlin , C. P. and O'Beirne , D. 1999 . Respiration rate of a dry coleslaw mix as affected by storage temperature and respiratory gas concentrations . Journal of Food Science , 64 ( 1 ) : 116 – 119 .
- Menon Rekha Ravindran; Goswami , T.K. 2008 . Modelling of respiration rates of green matures mango under aerobic conditions . Biosystems Engineering , 99 ( 2 ) : 239 – 248 .
- Bhande , S.D. , Ravindran , M.R. and Goswami , T.K. 2008; 87 . Respiration rates of banana fruits under aerobic conditions at different storage temperatures . Journal of Food Engineering , : 116 – 123 .
- Dash , K.K. 2007 . Modelling of respiration kinetics of fruits for control atmosphere storage and modified atmosphere storage , Kharagpur, , India : Unpublished M.Tech Thesis, Department of Agriculture and Food Engineering, Indian Institute of Technology .
- Maneerat , C. , Tongta , A. , Kanlayanarat , S. and Wongs-Aree , C. A transient model to predict O2 and CO2 concentrations in modified atmosphere packaging of bananas at various temperatures . Proceedings of the 7th international controlled atmosphere research conference . Edited by: Gorny , J. R. Vol. 5 , pp. 191 – 197 . Davis, CA
- Cornish-Bowden , A. 1979 . Fundamentals of enzyme kinetics , London : Butterworth Ltd .
- Hagger , P.E. , Lee , D.S. and Yam , K.L. 1992 . Application of an enzyme kinetic based respiration model to closed system experiments for fresh produce . Journal of Food Process Engineering , 15 : 143 – 157 .
- Lee , D.S. , Song , Y. and Yam , K.L. 1996 . Application of an enzyme kinetics based respiration model to permeable system experiment of fresh produce . Journal of Food Engineering , 27 : 297 – 310 .
- Mahajan , P. V. and Goswami , T. K. 2001 . Enzyme kinetics based modeling of respiration rate for apple . Journal of Agricultural Engineering Research , 79 ( 4 ) : 399 – 406 .
- Song , Y. , Kim , H.K. and Yam , K.L. 1992 . Respiration rate of blueberry in modified atmosphere at various temperatures . Journal of the American Society for Horticultural Science , 117 : 925 – 929 .
- Andrich , G. , Fiorentini , R. , Tuci , A. , Zinnai , A. and Sommovigo , G. 1991 . A tentative model to describe respiration of stored apples . Journal of the American Society for Horticultural Science , 116 : 478 – 481 .
- Andrich , G. , Zinnai , A. , Balzini , S. , Silvestri , S. and Fiorentini , R. 1998 . Aerobic respiration rate of golden delicious apples as a function of temperature and pO2 . Postharvest Biology and Technology , 14 : 1 – 9 .
- Cameron , A.C. , Beaudry , R.M. , Banks , N.H. and Yelanich , M.V. 1994 . Modified atmosphere packaging of blueberry fruit: modeling respiration and package oxygen partial pressures as a function of temperature . Journal of the American Society for Horticultural Science , 119 : 534 – 539 .
- Dadzie , B.K. , Banks , N.H. , Cleland , D.J. and Hewett , E.W. 1996 . Changes in respiration and ethylene production of apples in response to internal and external oxygen partial pressures . Postharvest Biology and Technology , 9 : 297 – 309 .
- Joles , D.W. , Cameron , A.C. , Shirazi , A. , Petracek , P.D. and Beaudry , R.M. 1994 . Modified atmosphere packaging of ‘Heritage’ red raspberry fruit: respiratory response to reduced oxygen, enhanced carbon dioxide and temperature . Journal of the American Society for Horticultural Science , 119 : 540 – 545 .
- Ratti , C. , Raghavan , G.S.V. and Gariepy , Y. 1996 . Respiration rate model and modified atmosphere packaging of fresh cauliflower . Journal of Food Engineering , 28 : 297 – 306 .
- Smyth , A.B. , Song , J. and Cameron , A.C. 1998 . Modified atmosphere packaged cut iceberg lettuce: effect of temperature and O2 partial pressure on respiration and quality . Journal of Agricultural and Food Chemistry , 46 : 4556 – 4562 .
- Wills , R.B.H. , McGlasson , W.B. , Graham , D. , Lee , T.H. and Hall , E.G. 1989 . Post harvest: An introduction to the physiology and Handling of Fruits and Vegetables , 174 New York : Chapman and Hall, Inc .
- Toledo , R.T. 1991 . “ Kinetics of chemical reactions in foods ” . In Fundamentals of food processing engineering , 138 – 176 . New York : Chapman and Hall Inc .
- Hong , S.I. and Kim , D.M. 2001 . Influence of oxygen concentration and temperature on respiratory characteristics of fresh cut green onion . International Journal of Food Science and Technology , 36 ( 3 ) : 283 – 289 .
- Lakakul , R. , Beaudry , R.M and Hernandez , R.J. 1999 . Modelling respiration of apple slices in modified atmosphere packages . Journal of Food Science , 64 ( 1 ) : 105 – 110 .
- Forward , D.F. 1960 . Effect of temperature on respiration . Encyclopedia of plant Physiology , 12 ( 2 ) : 234 – 258 .
- Ruiz-Cabrera , M.A. , Flores-Gomez , G. , Gonzalez-Garcia , R. , Grajales-Lagunes , A. , Moscosa-Santillan , M. and Abud-Archila , M. 2008 . Water diffusivity and quality attributes of fresh and partially osmodehydrated cactus pear (opuntia ficus indica) subjected to air dehydration . International Journal of Food Properties , 11 ( 4 ) : 887 – 900 .
- Ranganna , S. 1995 . Handbook of analysis and quality control for fruits and vegetable products , New Delhi, , India : Tata McGraw Pub. Co .
- Gungor , N. and Sengul , M. 2008 . Antioxidant Activity, Total Phenolic Content and Selected Physicochemical Properties of White Mulberry (Morus Alba L.) Fruits . International Journal of Food Properties , 11 ( 1 ) : 44 – 52 .
- Kang , J.S. and Lee , D.S. 1998 . A kinetic model for transpiration of fresh produce in a controlled atmosphere . Journal of Food Engineering , 35 : 65 – 73 .
- Prasad , M. 1995 . Development of modified atmosphere packaging system with permselective films for storage of red delicious apples , Kharagpur, , India : Unpublished Ph.D. Thesis, Department of Agriculture and Food Engineering, Indian Institute of Technology .
- Kays , S.J. 1991 . “ Metabolic Processes in Harvested Products Respiration ” . In Post Harvest Physiology of Perishable Plant Products , Edited by: Kay , S.J. 75 – 79 . New York : Van Nostrand Reinhold Publication .
- Raghavrao , D. 1983 . Statistical technique: Agricultural and biological research , 487 New Delhi : Oxford IBH Publishing Co .