Abstract
We investigated antioxidant and radical scavenging activity of lyophilized aqueous extract and ethanol extract of roots and barks of Turkish liquorices (Glycyrrhiza glabra L.) by employing various in vitro antioxidant assays such as DPPH• scavenging activity, ABTS•+ scavenging activity, DMPD•+ scavenging activity, O2 •− scavenging activity, total antioxidant activity, Fe3+-Fe2+ and Cu2+-Cu+ reducing abilities H2O2 scavenging and ferrous ions (Fe2+) chelating activities. Aqueous extract of aerial parts (AE-AP), ethanol extract of aerial parts (EE-AP), aqueous extract of root parts (AE-RP) and ethanol extract of root parts (EE-RP) of Turkish liquorices inhibited 87.9, 83.6, 88.6 and 80.1% lipid peroxidation of linoleic acid emulsion at 30 μg/mL concentration, respectively. On the other hand, α-tocopherol and trolox indicated inhibition of 68.1 and 81.3%, respectively.
INTRODUCTION
Licorice (Glycyrrhiza glabra L.) is a ligneous perennial shrub growing in Mediterranean and Asian regions including Turkey. Liquorice roots are cylindrical in shape having a diameter of 0.5 ± 2.5 cm and length of 15 ± 20 cm. It is found in dry grassy plains, and sunny mountainsides. It is widely used worldwide in food, confectionery and pharmaceutical products, such as cough syrups, herbal supplements, chewing gums, drinks, and candy.[Citation1] It has been reported that the extracts of liquorice have been widely used in the food industry as sweetening agent, flavor enhancer and a flavor modifier. It has also found widespread usage as a foaming agent in alcoholic and non-alcoholic beverages, in confectionery products, in halva and sweets. Commercially, the root extract of liquorice is supplied in concentrated liquid or powdered form for ease of transportation. For use in products, the concentrate or powder extract is diluted with water to the required concentration. Also, there is a growing commercial interest in using liquorice root extract in food foams. It can be used to modify food foams, to enhance foam stability or to impart flavor. It can also be used in the development of new foamy foods.[Citation2]
Licorice (Glycyrrhiza glabra L.) is a powerful natural sweetener, 50–170 times sweeter than sucrose. Licorice (Glycyrrhiza glabra L.) contains a variety of substances.[Citation1] Licorice (Glycyrrhiza glabra L.) contains a variety of substances. The chemical constituents of the roots include several bioactive compounds, such as glycyrrhizin (≈16%), different sugars (up to 18%) flavonoids, saponoids, sterols, starches, amino acids, gums and essential oils. Kitagawa reported the detailed structures of 33 constituents in licorice roots and their sweetness.[Citation3] Glycyrrhizin is a water-soluble pentacyclic triterpenoid glycoside responsible for the sweetness of licorice and its aglycone is responsible for various medicinal attributes and clinical applications in the treatment of spleen, sore throat, bronchitis, liver, kidney, and ulcer.[Citation4]
Reactive oxygen species (ROS) are continuously produced by the body's normal use of oxygen such as respiration and some cell mediated immune functions. ROS include free radicals such as O2 .−, hydroxyl radicals (OH·) and non free-radical species such as hydrogen peroxide (H2O2) and singlet oxygen (1O2).[Citation5–6] They are capable of damaging crucial biomolecules such as nucleic acids, lipids, proteins, polyunsaturated fatty acids and carbohydrates and may cause DNA damage that can lead to mutations. If ROS are not effectively scavenged by cellular constituents, they can stimulate free radical chain reactions subsequently damaging the cellular biomolecules such as proteins, lipids and nucleic acids and finally they lead to disease conditions. ROS have been implicated in more than 100 diseases.[Citation7,Citation8]
All aerobic organisms have antioxidant defenses, including antioxidant enzymes and antioxidant food constituents to remove or repair the damaged molecules. Antioxidant compounds can scavenge free radicals and increase shelf life by retarding the process of lipid peroxidation, which is one of the major reasons for deterioration of food and pharmaceutical products during processing and storage.[Citation9–11] Antioxidants can protect the human body from free radicals and ROS effects. They retard the progress of many chronic diseases as well as lipid peroxidation. Hence, a need for identifying alternative natural and safe sources of food antioxidants has been created, and the search for natural antioxidants, especially of plant origin, has notably increased in recent years.[Citation10,Citation11] Antioxidants are often added to foods to prevent the radical chain reactions of oxidation, and they act by inhibiting the initiation and propagation step leading to the termination of the reaction and delay the oxidation process.[Citation13–16] At the present time, the most commonly used antioxidants are BHA, BHT, propylgallate and tert-butyl hydroquinone. BHA and BHT are restricted by legislative rules because of doubts over their toxic and carcinogenic effects.[Citation15] Therefore, there is a growing interest on natural and safer antioxidants in food applications, and a growing trend in consumer preferences for natural antioxidants, all of which has given more impetus to explore natural sources of antioxidants.[Citation18–20]
The objective of this study was to investigate the inhibition of lipid peroxidation in linoleic acid system, ferric ions (Fe3+) reducing antioxidant power assay cupric ions (Cu2+) reducing antioxidant power assay (Cuprac method), DPPH· radical scavenging, ABTS•+ radical scavenging, DMPD•+ radical scavenging, superoxide anion radical scavenging in the riboflavin − methionine − illuminate system, hydrogen peroxide scavenging and ferrous ions (Fe3+) chelating activities aqueous extract of aerial parts (AE-AP), ethanol extract of aerial parts (EE-AP), aqueous extract of root parts (AE-RP) and ethanol extract of root parts (EE-RP) of Turkish liquorices (Glycyrrhiza glabra L.).
MATERIAL AND METHODS
Chemicals
N,N-Dimethyl-p-phenylenediamine (DMPD), neocuproine (2,9-dimethyl-1,10-phenanthroline) 2,2′-azino-bis(3-ethylbenzthiazoline-6-sulfonic acid) (ABTS), butylated hydroxyanisole (BHA), butylated hydroxytoluene (BHT), 1,1-diphenyl-2-picryl-hydrazyl (DPPH·), 3-(2-pyridyl)-5,6-bis (4-phenyl-sulfonic acid)-1,2,4-triazine (Ferrozine), riboflavin, methionine, linoleic acid were obtained from Sigma (Sigma-Aldrich GmbH, Sternheim, Germany). All other chemicals used were analytical grade and obtained from either Sigma-Aldrich or Merck.
Extraction Procedures
Aerial parts (500 g) and roots (500 g) of Turkish Liquorice (Glycyrrhiza glabra L.) were purchased from a local market from Gaziantep province of Turkey. Extraction carried out as described previously.[Citation21,Citation22] Turkish liquorices (Glycyrrhiza glabra L.) was shade dried initially. For water extraction, 25 g of air-dried Turkish liquorices (Glycyrrhiza glabra L.) ground into a fine powder in a mill and was mixed with 400 mL boiling water by magnetic stirrer during 15 min. Then the extract was filtered over cheesecloth and Whatman No. 1 paper, respectively. The filtrates were frozen at −84°C in ultra low temperature freezer (Sanyo, Japan) and lyophilized in a lyophilizator at 5 mm-Hg pressures at −50°C (Labconco, Freezone, Japan). The sample was lyophilized during three days. For extraction using ethanol, 25-g sample of Turkish liquorice was ground into a fine powder using a mill and then mixed with 500 mL ethanol. The residue was re-extracted under same condition until extraction solvents became colorless. The extracts thus obtained were filtered over Whatman No. 1 paper and the filtrate was collected, which was followed by ethanol removal using a rotary evaporator (RE 100 Bibby, Stone Staffordshire England, ST15 OSA) at 40°C to obtain dry extract. All the extracts were stored at −20°C in plastic bottles until used.[Citation16]
Determination of Total Phenolic Contents
Total phenolics were determined in aqueous extract of aerial parts (AE-AP), ethanol extract of aerial parts (EE-AP), aqueous extract of root parts (AE-RP) and ethanol extract of root parts (EE-RP) of Turkish liquorices according to a modified method of Slinkard and Singleton [Citation23] using by Folin-Ciocalteu phenolic reagent. Gallic acid was used as a standard phenolic compound. The amount of total phenolic compounds in the AE-AP, EE-AP, AE-RP, and EE-RP determined as microgram of gallic acid equivalent using an equation (Eq. 1) that was obtained from standard gallic acid graph (r 2: 0.9944).
The content of total phenolics in each extract was calculated from a standard curve prepared using gallic acid and expressed as micrograms of gallic acid equivalents (GAE).[Citation16]
Determination of Total Flavonoids
Total flavonoids amount in AE-AP, EE-AP, AE-RP and EE-RP was described formerly by Koksal and Gülçin.[Citation25] Total flavonoids concentration was calculated using quercetin as standard (Eq. 2):[Citation11,Citation16]
The content of total flavonoids in AE-AP, EE-AP, AE-RP and EE-RP was calculated from a standard above curve prepared using quercetin and expressed as micrograms of quercetin equivalents (QE).[Citation11,Citation16]
Total Antioxidant Activity Determination by Ferric Thiocyanate Method
The ferric thiocyanate method was used to evaluate the effect of AE-AP, EE-AP, AE-RP and EE-RP and reference antioxidants on preventing peroxidation of linoleic acid described previously.[Citation24,Citation25] The peroxide levels were determined by reading the absorbance at 500 nm in a spectrophotometer (Shimadzu, UV-1208 UV-VIS Spectrophotometer, Japan) after reaction with FeCl2 and thiocyanate at intervals during incubation. The peroxides formed during linoleic acid peroxidation will oxidize Fe+2 to Fe+3, which forms a complex with thiocyanate that has a maximum absorbance at 500 nm. The assay steps were repeated every 5 h until reaching a maximum. The percent inhibition was calculated at this point (50 h). Solution without AE-AP, EE-AP, AE-RP and EE-RP was used as blank samples. Linoleic acid mixture without the addition of sample was used as a control. The percent inhibition of lipid peroxidation in linoleic acid emulsion was calculated by following EquationEq. (3):
Ferric Cyanide (Fe3+) Reducing Antioxidant Power Assay (FRAP)
Reducing power was measured by the direct reduction of Fe3+(CN−)6 to Fe2+(CN−)6 and was determined by absorbance measurement of the formation of the Perl's Prussian Blue complex following the addition of excess Fe3+, as described previously.[Citation28] For this reason, the ferric reducing antioxidant power method of Oyaizu[Citation29] with slight modification[Citation31,Citation32] was used to measure the reducing capacity of AE-AP, EE-AP, AE-RP and EE-RP. Increased absorbance of the reaction mixture indicates grater reduction capability.[Citation33,Citation35]
Cupric Ions (Cu2+) Reducing Power-Cuprac Assay
For reducing ability of AE-AP, EE-AP, AE-RP and EE-RP, the cupric ions (Cu2+) reducing power method was also used[Citation24,Citation33] with slight modification. Absorbance against a reagent blank was measured at 450 nm after 30 min. Increased absorbance of the reaction mixture indicates increased reduction capability.
Chelating Activity on Ferrous Ions (Fe2+)
Ferrous ions (Fe2+) chelating activity was measured by inhibition of the formation of Fe2+-ferrozine complex after treatment of test material with Fe2+, following the method of Dinis.[Citation34] Fe2+-chelating ability of AE-AP, EE-AP, AE-RP and EE-RP was monitored by the absorbance of the ferrous iron-ferrozine complex at 562 nm. The percentage of inhibition of ferrozine-Fe2+ complex formation was calculated by using the formula (Eq. 4) given bellow:
Hydrogen Peroxide Scavenging Activity
The hydrogen peroxide scavenging assay was carried out following the procedure of Ruch and co-workers.[Citation35] The principle of this method is there a decrease in absorbance of H2O2 upon oxidation of H2O2. The concentration of hydrogen peroxide (mM) in the assay medium was determined using a standard curve (Eq. 5) (r2 :0.9956):
The percentage of H2O2 scavenging by AE-AP, EE-AP, AE-RP and EE-RP and standard compounds was calculated using the following equation (Eq. 6):
DPPH• Free Radical Scavenging Activity
The hydrogen atom or electron donation abilities of some pure compounds were measured by the bleaching of a purple colored methanol solution of the stable DPPH radical. This spectrophotometric assay uses the stable radical, 1,1-diphenyl-2-picrylhydrazyl (DPPH•), as a reagent. The method of Blois[Citation36] previously described by Ak and Gülçin[Citation28] was used with slight modifications in order to assess the DPPH· free radical scavenging capacity of AE-AP, EE-AP, AE-RP and EE-RP. A standard curve was prepared using different concentrations of DPPH·. The DPPH· scavenging capacity was expressed as mM in the reaction medium and calculated from the calibration curve (EquationEq. 7) determined by linear regression (r2 :0.9845):
The capability to scavenge the DPPH· radical was calculated using the following equation (Eq. 8):
ABTS Radical Decolourization Assay
In this method, an antioxidant is added to a pre-formed 2,2′-azino-bis(3-ethylbenzthiazoline-6-sulfonic acid) (ABTS) radical solution and after a fixed time period the remaining ABTS•+ is quantified spectrophotometrically at 734 nm.[Citation26] The extent of depolarization is calculated as percentage reduction of absorbance. For preparation of a standard curve, different concentrations of ABTS•+ (0.033-0.33 mM) were used. The ABTS•+ concentration (mM) in the reaction medium was calculated from the following calibration curve (Eq. 9), determined by linear regression (r 2:0.9899):
The scavenging capability of test compounds was calculated using the following equation (Eq. 10):
Superoxide Anion Radical Scavenging Activity
Superoxide radicals were generated by method of Beauchamp and Fridovich[Citation40] with slight modification.[Citation38] Superoxide radicals are generated in riboflavin, methionine, illuminate and assayed by the reduction of NBT to form blue formazan. The photochemically reduced riboflavin generated O2 •- which reduced NBT to form blue formazan. The un-illuminated reaction mixture was used as a blank. The absorbance was measured at 560 nm. AE-AP, EE-AP, AE-RP, and EE-RP were added to the reaction mixture, in which O2 •- was scavenged, thereby inhibiting the NBT reduction. Decreased absorbance of the reaction mixture indicates increased superoxide anion scavenging activity. The percentage of superoxide anion scavenged was calculated by using the following formula (Eq. 11):
where λ560-C is the absorbance of the control; and λ560-S is the absorbance in presence of AE-AP, EE-AP, AE-RP and EE-RP or standards.[Citation41,Citation42]
Measurement of DMPD•+ Scavenging Ability
N,N-dimethyl-p-phenyl-enediamine dihydrochloride (DMPD) radical scavenging ability of AE-AP, EE-AP, AE-RP and EE-RP was determined according to Fogliano and co-workers.[Citation43] The DMPD•+ concentration (mM) in the reaction medium was calculated from the following calibration curve (Eq. 12), determined by linear regression (r 2:0.9993):
The scavenging capability of ABTS•+ radical was calculated using the following equation (Eq. 13):
Statistical Analysis
The experimental results were performed in triplicate. The data were recorded as mean ± standard deviation and analyzed by SPSS (version 11.5 for Windows 2000, SPSS Inc.). One-way analysis of variance (ANOVA) was performed by procedures. Significant differences between means were determined by Duncan's Multiple Range tests, and p < 0.05 was regarded as significant and p 0.01 was very significant.
RESULTS AND DISCUSSION
Phenolic compounds are widely distributed in plants and are very important in human diet and health. For determining total phenolic contents, calibration curves were obtained using known quantities of standard gallic acid. As can be seen in , the phenolic compounds of 1 mg of AE-AP, EE-AP, AE-RP, and EE-RP ranged from 75.7 to 185.7 μg GAE, respectively. AE-AP possessed the highest the phenolic compounds. Flavonoids are an important group of natural compounds, which can prevent coronary heart disease and have antioxidant properties. Most of them belong to the Mediterranean food culture. During the last few decades, they attracted great interest because of their beneficial effects on human health.[Citation39] On the other hand, total content flavonoids in AE-AP, EE-AP, AE-RP and EE-RP was determined spectrophotometrically and found to be 2.6, 4.4, 5.1, and 4.2 μg quercetin equivalents, respectively ().
Figure 1 Total phenolic contents as gallic acid equivalent (GAE/1 mg of dried extract) in roots and aerial parts of Licorice.
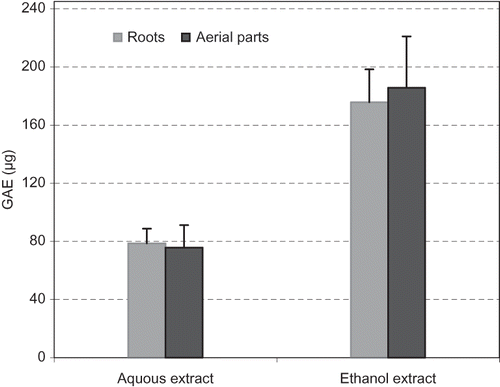
Figure 2 Total flavonoids contents as quercetin equivalent (QE/1 mg of dried extract) in roots and aerial parts of Licorice.
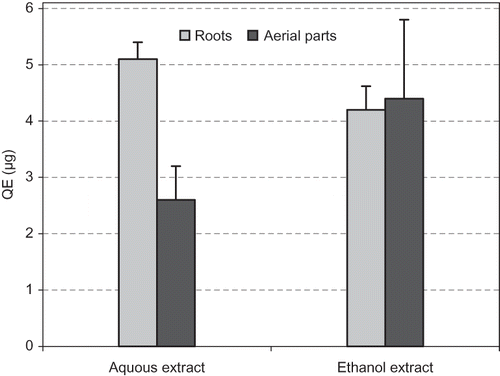
Antioxidant activity is defined as the ability of a compound to inhibit oxidative degradation, such as lipid peroxidation and widely used as a parameter for medicinal bioactive components. The ferric thiocyanate method measures the amount of peroxide produced during the initial stages of oxidation, which are the primary products of oxidation. AE-AP, EE-AP, AE-RP, and EE-RP exhibited effective antioxidant activity in the linoleic acid emulsion system. The effect of 30 μg/mL concentration of AE-AP, EE-AP, AE-RP and EE-RP on lipid peroxidation of linoleic acid emulsion is shown in and was found to be 87.9, 83.6, 88.6, and 80.1%, respectively. On the other hand α-tocopherol and trolox exhibited 68.1 and 81.3% on peroxidation of linoleic acid emulsion at the same concentration, respectively. The autoxidation of linoleic acid emulsion without AE-AP, EE-AP, AE-RP, and EE-RP or standard compounds was accompanied by a rapid increase of lipid peroxides. Consequently, these results clearly indicated that all of extracts had effective and powerful antioxidant activity. Lipid hydroperoxides are formed in membranes in vivo. Their detoxification appears to be critical for the survival of an organism in oxidative stress.[Citation44] Therefore antioxidants play a vital role in inhibition of lipid peroxidation or by protecting against cellular damage by free radicals. In this method, ferrous chloride and thiocyanate react with each other to produce ferrous thiocyanate by means of hydroperoxides.[Citation46] The ferric thiocyanate method measures the amount of peroxide produced during the initial stages of oxidation, which is the primary product of lipid oxidation. In this assay, hydroperoxides produced from linoleic acid added to the reaction mixture, which has oxidized in air during the experimental period, were indirectly measured.
Table 1 Total antioxidant activity by ferric thiocyanate method, 2,2′-azino-bis(3-ethylbenzthiazoline-6-sulfonic acid) (ABTS) radical scavenging activity, 1,1-diphenyl-2-picryl-hydrazyl (DPPH•) free radical scavenging activity, N,N-dimethyl-p-phenylenediamine (DMPD) radical scavenging activity, superoxide anion radical (O2 •-) scavenging activity and hydrogen peroxide scavenging activity of aqueous extract of aerial parts (AE-AP), ethanol extract of aerial parts (EE-AP), aqueous extract of root parts (AE-RP), and ethanol extract of root parts (EE-RP) of Turkish liquorices (Glycyrrhiza glabra L.)
Antioxidants can be reductants and inactivation of oxidants by reductants can be described as redox reactions in which one reaction species is reduced at the expense of the oxidation of the other. Also, AE-AP, EE-AP, AE-RP, and EE-RP had effective reducing power using the potassium ferricyanide reduction and cupric ions (Cu2+) reducing methods when compared to the standards like α-tocopherol and trolox. For the measurements of the reductive ability of AE-AP, EE-AP, AE-RP, and EE-RP, the Fe3+-Fe2+ transformation was investigated in the presence of these extracts using the method of Oyaizu.[Citation27] The reducing capacity of a compound or crude extract can be measured by the direct reduction of Fe[(CN)6]3 to Fe[(CN)6]2. As can be seen from , AE-AP, EE-AP, AE-RP, and EE-RP demonstrated potent Fe3+ reducing ability and these differences were statistically very significant (p 0.01). Reducing power of AE-AP, EE-AP, AE-RP, and EE-RP and standard compounds exhibited the following order: trolox > α-tocopherol > EE-AP > EE-RP > AE-AP > AE-RP. The results demonstrated that AE-AP, EE-AP, AE-RP and EE-RP had marked ferric ions (Fe3+) reducing ability and had electron donor properties for neutralizing free radicals by forming stable products. The outcome of the reducing reaction is to terminate the radical chain reactions that may otherwise be very damaging.
Table 2 Total reducing power by potassium ferricyanide reduction method and cupric ion (Cu2+) reduction capacity by Cuprac method and ferrous ions (Fe2+) chelating activity of aqueous extract of aerial parts (AE-AP), ethanol extract of aerial parts (EE-AP), aqueous extract of root parts (AE-RP) and ethanol extract of root parts (EE-RP) of Turkish liquorices (Glycyrrhiza glabra L.)
The Cuprac method was developed for a reducing power assay. Cupric ions (Cu2+) reducing ability of AE-AP, EE-AP, AE-RP, and EE-RP and standard compounds was shown in . It was found a correlation between the cupric ions (Cu2+) reducing ability and AE-AP, EE-AP, AE-RP, and EE-RP concentrations. Cupric ions (Cu2+) reducing capability of AE-AP, EE-AP, AE-RP, and EE-RP by Cuprac method was found concentration dependently (30 μg/mL). Cupric ions (Cu2+) reducing power of all extracts and standard compounds at the same concentration (30 μg/mL) exhibited the following order: α-tocopherol > trolox > EE-RP > AE-RP > AE-AP > EE-AP. Cuprac method is capable of measuring thiol-type antioxidants such as glutathione and non-protein thiols unlike the widely applied FRAP test, which is non-responsive to -SH group antioxidants.[Citation45]
Ferric ions also produce radicals from peroxides although the rate is tenfold less than that of ferrous ion. Ferrous ion is the most powerful pro-oxidant among the various species of metal ions. Minimizing ferrous ion may afford protection against oxidative damage by inhibiting production of ROS and lipid peroxidation. Ferrozine can quantitatively form complexes with Fe2+ in this method. In the presence of chelating agents, complex formation is disrupted, resulting in a decrease in the red colour of the complex. Measurement of colour reduction therefore allows estimation of the metal chelating activity of the coexisting chelators. Lower absorbance indicates higher metal chelating activity. Metal chelation is an important antioxidant property[Citation46,Citation47] and hence AE-AP, EE-AP, AE-RP and EE-RP were assessed for its ability to compete with ferrozine for ferrous ion in the solution.
AE-AP, EE-AP, AE-RP and EE-RP had effective chelating effect on ferrous ions (Fe2+). At the same concentration of (20 μg/mL) AE-AP, EE-AP, AE-RP, and EE-RP were differ from control values (p < 0.01). In addition, AE-AP, EE-AP, AE-RP and EE-RP exhibited 87.9%, 83.2%, 88.6%, and 80.1% halation of ferrous ion at 20 μg/mL concentration. As can be seen in , the ferrous ion chelating effect of the AE-AP, EE-AP, AE-RP, and EE-RP was compared to that of α-tocopherol and EDTA. On the other hand, the ferrous ion chelating capacity of the same concentration of α-tocopherol and trolox were found to be 90.9 and 49.3%, respectively. These results show that the ferrous ion chelating effect of AE-AP, EE-AP, AE-RP and EE-RP was statistically similar to α-tocopherol but higher than that of trolox. In this assay, AE-AP, EE-AP, AE-RP, and EE-RP interfered with the formation of the ferrous-ferrozine complex. It suggests AE-AP, EE-AP, AE-RP, and EE-RP have chelating activity and is able to capture ferrous ion before ferrozine.[Citation26]
Hydrogen peroxide can produce in biological systems. It is not very reactive; however, it can sometimes be toxic to cells. H2O2 can attack many cellular energy-producing systems. For instance, it deactivates the glycolytic enzyme glyceraldehyde-3-phosphate dehydrogenase.[Citation48] The ability of AE-AP, EE-AP, AE-RP, and EE-RP to scavenge H2O2 is shown in and compared with that of α -tocopherol and trolox as reference compounds. Hydrogen peroxide scavenging activity of AE-AP, EE-AP, AE-RP and EE-RP at 30 μg/mL was found to be 34.6%, 37.5%, 51.2%, and 41.4%. On the other hand, α-tocopherol and trolox exhibited 39.1% and 37.7% hydrogen peroxide scavenging activity at the same concentration, respectively. These results show that the used extracts have an effective H2O2scavenging activity. At the above concentration, the H2O2scavenging effect of all extracts and standard compounds decreased in the order of AE-RP > EE-RP > α-tocopherol > trolox > EE-AP > AE-AP.
DPPH•, ABTS•+, DMPD•+ or radical-scavenging methods are common spectrophotometric procedures for determining the antioxidant capacities of components. When an antioxidant is added to the radicals, there is a degree of depolarization owing to the presence of the antioxidants, which reverses the formation of the DPPH• radical, ABTS•+ and DMPD•+ cation:
These chromogens have a high sensitivity, are easy to use, and allow for rapid analysis of the antioxidant activity of a large number of samples. DPPH assay has been widely used to evaluate the free radical scavenging effectiveness of various antioxidant substances.[Citation30,Citation51] In this assay, the antioxidants were able to reduce the stable radical DPPH to the yellow colored diphenyl-picrylhydrazine. This method is based on the reduction of DPPH in alcoholic solution in the presence of a hydrogen-donating antioxidant due to the formation of the non-radical form DPPH-H in the reaction. DPPH is usually used as a reagent to evaluate free radical scavenging activity of antioxidants. DPPH is a stable free radical and accepts an electron or hydrogen radical to become a stable diamagnetic molecule.[Citation49]
illustrates a significant decrease in the concentration of DPPH radical due to the scavenging ability of AE-AP, EE-AP, AE-RP, and EE-RP and the reference compounds. α-Tocopherol and trolox were used as references for radical scavenger activity. At the same concentration (30 μg/mL), DPPH radical scavenging decreased as follow EE-AP > trolox > EE-RP > AE-RP > α-tocopherol > AE-AP and were found as 57.5, 56.5, 57.4, 52.2, 51.9, and 42.9.
ABTS•+ radicals are more reactive than DPPH radicals and unlike the reactions with DPPH radical, which involve H atom transfer, the reactions with ABTS•+ radicals involve an electron transfer process. As seen in , AE-AP, EE-AP, AE-RP, and EE-RP is an effective ABTS•+ radical scavenger in a concentration-dependent manner (20 μg/mL). There is a significant decrease (p < 0.01) in the concentration of ABTS•+ due to the scavenging capacity at all AE-AP, EE-AP, AE-RP, and EE-RP concentrations. On the other hand, ABTS•+ radical scavenging activity of AE-AP, EE-AP, AE-RP, and EE-RP, α-tocopherol and trolox were found to be 80.3.98.7, 81.7, 95.8, 55.9, and 90.7 μg/mL, respectively. The scavenging effect of these extracts and standard compounds on the ABTS•+ decreased in the order: EE-AP ≈ EE-RP ≈ trolox > AE-RP ≈ AE-AP > α-tocopherol, at this concentration (30 μg/mL). ABTS•+ radical scavenging method is a direct generation of a stable form of radical to create a blue-green ABTS•+ chromophore prior to the reaction with antioxidants.[Citation50,Citation51]
AE-AP, EE-AP, AE-RP, and EE-RP had marked inhibition of superoxide radical generation. As seen in , the inhibition of superoxide anion radical generation by 30 μg/mL concentration of AE-AP, EE-AP, AE-RP, and EE-RP was found to be 39.7%, 40.2%, 41.0%, and 21.6%, respectively. On the other hand, at the same concentration α-tocopherol and trolox exhibited 21.3 and 23.9% superoxide anion radical scavenging activity, respectively. According to these results, AE-AP, EE-AP and AE-RP had higher superoxide anion radical scavenging activity than all of tested reference compounds and these differences statically were found as significant (p < 0.01). Also, EE-RP had close superoxide anion radical scavenging activity to α-tocopherol and trolox.
The UV-visible spectrum of DMPD•+ shows a maximum absorbance at 505 nm. This assay reflects the ability of radical hydrogen-donors to scavenge the single electron from DMPD•+.[Citation24]As shown in , AE-AP, EE-AP, AE-RPM, and EE-RP exhibited an effective DMPD•+ radical scavenging activity. Percent DMPD•+ radical scavenging activity of AE-AP, EE-AP, AE-RP, and EE-RP were found to be 44.2%, 38.2%, 69.6%, and 54.2%, respectively. On the other hand, at the same concentration this value was found as 88.8 μg/mL for trolox. There was a significant decrease (p < 0.05) control value and DMPD•+ scavenging capacity of AE-AP, EE-AP, AE-RP, and EE-RP.
Superoxide is important as the product of the one-electron reduction of dioxygen, which occurs widely in nature. It is an oxygen-centered radical with selective reactivity. Superoxide anions derived from dissolved oxygen by the riboflavin-methionine-illuminate system will reduce NBT in this system. In this method, superoxide anion reduces the yellow dye (NBT2+) to produce the blue formazan, which is measured spectrophotometrically at 560 nm. Antioxidants are able to inhibit the blue NBT formation.[Citation39,Citation48,Citation52] The decrease of absorbance at 560 nm with antioxidants indicates the consumption of superoxide anion in the reaction mixture. shows the inhibition of superoxide radical generation by 15 μg/mL concentration of AE-AP, EE-AP, AE-RP and EE-RP and standards.
In conclusion, AE-AP, EE-AP, AE-RP, and EE-RP were found to be an effective antioxidant in different in vitro assays including: reducing power, DPPH•, ABTS•+, DMPD•+, and O2 •- radical scavenging, hydrogen peroxide scavenging and metal chelating activities when it is compared to standard antioxidant compounds such as α-tocopherol, a natural antioxidant, and trolox which is a water-soluble analogue of α-tocopherol. Based on the discussion above, AE-AP, EE-AP, AE-RP, and EE-RP can be used for minimizing or preventing lipid oxidation in pharmaceutical products, retarding the formation of toxic oxidation products, maintaining nutritional quality and prolonging the shelf life of pharmaceuticals.
ACKNOWLEDGMENT
This study was supported by Research Fund of Atatürk University. The author is grateful to the Research Fund of Atatürk University for financial support (Project no: 2008/75).
REFERENCES
- Xu , H. , Fabricant , D.S. , Piersen , C.E. , Bolton , J.L. , Pezzuto , J.M. Fong , H. 2002 . A preliminary RAPD-PCR analysis of Cimicifuga species and other botanicals used for women's health . Phytomedicine , 9 : 757 – 762 .
- Ibanoglu , E. and Ibanoglu , S. 2000 . Foaming behaviour of liquorice (Glycyrrhiza glabra) extract . Food Chemistry , 70 : 333 – 336 .
- Kitagawa , I. 2002 . Licorice root. A natural sweetener and an important ingredient in Chinese medicine . Pure and Applied Chemistry , 74 : 1189 – 1198 .
- Mukhopadhyay , M. and Panja , P. 2008 . A novel process for extraction of natural sweetener from licorice (Glycyrrhiza glabra) roots . Separation and Purification Technology , 63 : 539 – 545 .
- Gülçin , İ. 2009 . Antioxidant activity of L-Adrenaline: An activity-structure insight . Chemico-Biological Interaction , 179 : 71 – 80 .
- Farombi , E.O. and Fakoya , A. 2005 . Free radical scavenging and antigenotoxic activities of natural phenolic compounds in dried flowers of Hibiscus sabdariffa . L Molecular Nutrition and Food Research , 49 : 1120 – 1128 .
- Gülçin , İ. 2006 . Antioxidant and antiradical activities of L-carnitine . Life Sciences , 78 : 803 – 811 .
- Halliwell , B. and Gutteridge , J.M.C. 1990 . Role of free radicals and catalytic metal ions in human disease: an overview . Methods in Enzymology , 186 : 1 – 85 .
- Gülçin , İ. , Oktay , M. , Kireçci , E. and Küfrevioğlu , Ö.İ . 2003 . Screening of antioxidant and antimicrobial activities of anise (Pimpinella anisum L.) seed extracts . Food Chemistry , 83 : 371 – 382 .
- Gülçin , İ. 2010 . Antioxidant properities of resveratrol: a structure-activity insight . Innovative Food Science and Emerging Technologies , 11 : 210 – 218 .
- Gülçin , İ. , Mshvildadze , V. , Gepdiremen , A. and Elias , R. 2004 . Antioxidant activity of saponins isolated from ivy: a-Hederin, hederasaponin-C, hederacolchiside-E and hederacolchiside F . Planta Medica , 70 : 561 – 563 .
- Köksal , E. and Gülçin , İ. 2008 . Antioxidant activity of cauliflower (Brassica oleracea L.) . Turkish Journal of Agriculture and Forestry , 32 : 65 – 78 .
- Lai , L.S. , Chou , S.T. and Chao , W.W. 2001 . Studies on the antioxidative activities of Hsian-tsao (Mesona procumbens Hemsl) leaf gum . Journal of Agricultural and Food Chemistry , 49 : 963 – 968 .
- Gülçin , İ. , Büyükokuroğlu , M.E. , Oktay , M. and Küfrevioğlu , Ö.İ. 2002 . On the in vitro antioxidant properties of melatonin . Journal of Pineal Research , 33 : 167 – 171 .
- Shahidi , F. , Janitha , P.K. and Wanasundara , P.D. 1992 . Phenolic antioxidants . Critical Reviews in Food Science and Nutrition , 32 : 67 – 103 .
- Gülçin , İ. , Şat , İ.G. , Beydemir , Ş. , Elmastaş , M. and Küfrevioğlu , Ö.İ . 2004 . Comparison of antioxidant activity of clove (Eugenia caryophylata Thunb) buds and lavender (Lavandula stoechas L.) . Food Chemistry , 87 : 393 – 400 .
- Gülçin , İ. 2005 . The antioxidant and radical scavenging activities of black pepper (Piper nigrum) seeds . International Journal of Food Sciences and Nutrition , 56 : 491 – 499 .
- Gülçin , İ. 2007 . Comparison of in vitro antioxidant and antiradical activities of L-tyrosine and L-Dopa . Amino Acids , 32 : 431 – 438 .
- Moure , A. , Cruz , J.M. , Franco , D. , Dominguez , J.M. , Sineiro , J. , Dominguez , H. , Nunez , M.J. and Parajo , J.C. 2001 . Natural antioxidants from residual sources . Food Chemistry , 72 : 145 – 171 .
- Oktay , M. , Gülçin , İ. and Küfrevioğlu , Ö.İ. 2003 . Determination of in vitro antioxidant activity of fennel (Foeniculum vulgare) seed extracts . Lebensmittel Wissenchaft und Technologie , 36 : 263 – 271 .
- Gülçin , İ. 2006 . Antioxidant activity of caffeic acid (3,4-dihydroxycinnamic acid) . Toxicology , 217 : 213 – 220 .
- Gülçin , İ. , Şat , İ.G. , Beydemir , Ş. and Küfrevioğlu , Ö.İ . 2004 . Evaluation of the in vitro antioxidant properties of extracts of broccoli (Brassica oleracea L.) . Italian Journal of Food Sciences , 16 : 17 – 30 .
- Elmastaş , M. , Gülçin , İ. , Beydemir , Ş. , Küfrevioğlu , Ö.İ and Aboul-Enein , H.Y. 2006 . A study on the in vitro antioxidant activity of juniper (Juniperus communis L.) seeds extracts . Analytical Letters , 39 : 47 – 65 .
- Slinkard , K. and Singleton , V.L. 1977 . Total phenol analyses: Automation and comparison with manual methods . American Journal of Enology and Viticulture , 28 : 49 – 55 .
- Köksal , E. , Gülçin , İ. , Öztürk Sarıkaya , S.B. and Bursal , E. 2008 . On the in vitro antioxidant activity of silymarin . Journal of Enzyme Inhibition and Medicinal Chemistry , 24 : 395 – 405 .
- Gülçin , İ. 2008 . In vitro prooxidant effect of caffeine . Journal of Enzyme Inhibition and Medicinal Chemistry , 23 : 149 – 152 .
- Gülçin , İ. , Elias , R. , Gepdiremen , A. and Boyer , L. 2006 . Antioxidant activity of lignans from fringe tree (Chionanthus virginicus L.) . European Food Research and Technology , 223 : 759 – 767 .
- Gülçin , İ. , Mshvildadze , V. , Gepdiremen , A. and Elias , R. 2006 . Antioxidant activity of a triterpenoid glycoside isolated from the berries of Hedera colchica: 3-O-(β-D-glucopyranosyl)-hederagenin . Phototherapy Research , 20 : 130 – 134 .
- Ak , T. and Gülçin , İ. 2008 . Antioxidant and radical scavenging properties of curcumin . Chemico-Biological Interaction , 174 : 27 – 37 .
- Oyaizu , M. 1986 . Studies on product of browning reaction prepared from glucose amine . Japanese Journal of Nutrition , 44 : 307 – 315 .
- Elmastas , M. , Gülçin , İ. , Işıldak , Ö. , Küfrevioğlu , Ö.İ , İbaoğlu , K. and Aboul-Enein , H.Y. 2006 . Antioxidant capacity of bay (Laurus nobilis L.) leave extracts . Journal of the Iranian Chemical Society , 3 : 258 – 266 .
- Büyükokuroğlu , M.E. , Gülçin , İ. , Oktay , M. and Küfrevioğlu , Ö.İ. 2001 . In vitro antioxidant properties of dantrolene sodium . Pharmacological Research , 44 : 491 – 495 .
- Gülçin , İ. , Elmastas , M. and Aboul-Enein , H.Y. 2007 . Determination of antioxidant and radical scavenging activity of basil (Ocimum basilicum) assayed by different methodologies . Phytotherapy Research , 21 : 354 – 361 .
- Apak , R. , Güçlü , K. , Özyürek , M. , Karademir , S.E. and Erça , E. 2006 . The cupric ion reducing antioxidant capacity and polyphenolic content of some herbal teas . International Journal of Food Sciences and Nutrition , 57 : 292 – 304 .
- Dinis , T.C.P. , Madeira , V.M.C. and Almeida , L.M. 1994 . Action of phenolic derivates (acetoaminophen, salycilate, and 5-aminosalycilate) as inhibitors of membrane lipid peroxidation and as peroxyl radical scavengers . Archive of Biochemistry and Biophysics , 315 : 161 – 169 .
- Ruch , R.J. , Cheng , S.J. and Klaunig , J.E. 1989 . Prevention of cytotoxicity and inhibition of intracellular communication by antioxidant catechins isolated from Chinese green tea . Carcinogenesis , 10 : 1003 – 1008 .
- Blois , M.S. 1958 . Antioxidant determinations by the use of a stable free radical . Nature , 26 : 1199 – 1200 .
- Gülçin , İ. , Beydemir , Ş. , Alici , H.A. , Elmastaş , M. and Büyükokuroğlu , M.E. 2004 . In vitro antioxidant properties of morphine . Pharmacological Research , 49 : 59 – 66 .
- Gülçin , İ. , Berashvili , D. and Gepdiremen , A. 2005 . Antiradical and antioxidant activity of total anthocyanins from Perilla pankinensis decne . Journal of Ethnopharmacology , 101 : 287 – 293 .
- Gülçin , İ. , Kirecci , E. , Akkemik , E. , Topal , F. and Hisar , O. 2010 . Antioxidant and antimicrobial activities of an aquatic plant: duckweed (Lemna minor L.) . Turkish Journal of Agriculture and Forestry , 34 ( 2 ) : 175 – 188 .
- Beauchamp , C. and Fridovich , I. 1971 . Superoxide dismutase: improved assays and an assay applicable to acrylamide gels . Analytical Biochemistry , 44 : 276 – 287 .
- Gülçin , İ. , Küfrevioğlu , Ö.İ. , Oktay , M. and Büyükokuroğlu , M.E. 2004 . Antioxidant, antimicrobial, antiulcer and analgesic activities of nettle (Urtica dioica L.) . Journal of Ethnopharmacology , 90 : 205 – 215 .
- Gülçin , İ. , Oktay , M. , Küfrevioğlu , Ö.İ. and Aslan , A. 2002 . Determination of antioxidant activity of lichen Cetraria islandica . (L) Ach. Journal of Ethnopharmacology , 79 : 325 – 329 .
- Fogliano , V. , Verde , V. , Randazzo , G. and Ritieni , A. 1999 . Method for measuring antioxidant activity and its application to monitoring the antioxidant capacity of wines . Journal of Agricultural and Food Chemistry , 47 : 1035 – 1040 .
- Inatani , R. , Nakatani , N. and Fuwa , H. 1983 . Antioxidative effect of the constituents of rosemary (Rosemarinus officinalis L.) and their derivatives . Agricultural and Biological Chemistry , 47 : 521 – 528 .
- Apak , R. , Güçlü , K. , Özyürek , M. and Karademir , S.E. 2004 . A novel total antioxidant capacity index for dietary polyphenols, vitamin C and E, using their cupric ion reducing capability in the presence of neocuproine: The CUPRAC method . Journal of Agricultural and Food Chemistry , 52 : 7970 – 7981 .
- Gulcin , İ. , Tel , A.Z. and Kirecci , E. 2008 . Antioxidant, antimicrobial, antifungal and antiradical activities of Cyclotrichium niveum (Boiss.) Manden and Scheng . International Journal of Food Properties , 11 ( 2 ) : 450 – 471 .
- Kehrer , J.P. 2000 . The Haber–Weiss reaction and mechanisms of toxicity . Toxicology , 149 : 43 – 50 .
- Gülçin , İ. , Elias , R. , Gepdiremen , A. , Boyer , L. and Köksal , E. 2007 . A comparative study on the antioxidant activity of fringe tree (Chionanthus virginicus L.) extracts . African Journal of Biotechnology , 6 : 410 – 418 .
- Gülçin , İ. , Mshvildadze , V. , Gepdiremen , A. and Elias , R. 2006 . Screening of antioxidant and antiradical activity of monodesmosides and crude extract from Leontice smirnowii . Tuber. Phytomedicine , 13 : 343 – 351 .
- MacDonald-Wicks , L.K. , Wood , L.G. and Garg , M.L. 2006 . Methodology for the determination of biological antioxidant capacity in vitro: A review . Journal of Sciences and Food Agriculture , 86 : 2046 – 2056 .
- Gülçin , İ. , Elias , R. , Gepdiremen , A. , Taoubi , K. and Köksal , E. 2009 . Antioxidant secoiridoids from fringe tree (Chionanthus virginicus L.) . Wood Sciences and Technology , 43 : 195 – 212 .
- Elmastas , M. , Türkekul , İ. , Öztürk , L. , Gülçin , İ. , Işıldak , Ö. and Aboul-Enein , H.Y. 2006 . The antioxidant activity of two wild edible mushrooms (Morchella vulgaris and Morchella esculanta) . Combinatorial Chemistry and High Throughput Screening , 9 ( 6 ) : 443 – 448 .