Abstract
The Dynamic Dewpoint isotherm method directly determines sample aw using chilled-mirror technology, while changes in sample weight are tracked gravimetrically. The objective of this research was to compare the Dynamic Dewpoint isotherms to saturated salt slurry isotherms for five materials: dent corn starch, isolated soy protein, microcrystalline cellulose, crystalline sucrose, and corn flakes. The Dynamic Dewpoint isotherms were obtained using the AquaSorp Isotherm Generator between 0.10 and 0.95 aw at 25°C. Comparison working isotherms were obtained using 12 saturated salt slurries, between 0.064 and 0.973 aw in desiccators and proximity equilibration cells at 25°C. The Dynamic Dewpoint isotherms exhibited similar sorption behavior to the saturated salt slurry isotherms, except for corn flakes, where there was a marked decrease in moisture content compared to the saturated salt slurry isotherms between 0.40 and 0.70 aw. This difference was attributed to the slow diffusion of water into the very dense laminated corn flake matrix, highlighting the rate of sorption (time) dependency of the Dynamic Dewpoint isotherm method. The Dynamic Dewpoint isotherm method offers the opportunity for real-time investigation of water sorption-related material properties, such as the glass to rubbery transition, recrystallization, hydrate formation, and deliquescence.
INTRODUCTION
Water is a key component involved in numerous facets of food systems, such as processing, occurrence of phase transitions, chemical, biochemical and physical stability, sensory perception, and, most importantly, microbial safety. Water activity (aw), which is defined as the ratio between the vapor pressure of water in equilibrium with a sample and the vapor pressure of pure water at the same temperature and atmospheric pressure, is used to express the availability of water in a food system. Moisture content of a material (usually expressed as a mass of water per unit mass of dry material) plotted as a function of aw (also termed the relative vapor pressure or food relative humidity) at a constant temperature yields a very useful assessment tool identified as the moisture sorption isotherm. A moisture sorption isotherm relates the amount of water sorbed (either adsorption or desorption) at equilibrium (or steady state) by a material at a given aw (or relative humidity, %RH) at a constant temperature. Sorption isotherms are used for a variety of processing and product stability applications, such as prediction of moisture transfer, development of new products, determination of product stability and shelf life, and process design and control.Citation[1,Citation2]
Traditionally, isotherms have been generated by placing the food material over saturated salt slurries with specific temperature dependent aw values in closed chambers (e.g., desiccators) and waiting until the food material attains a steady state, that is, reaches the same aw as the saturated salt slurry, as shown by minimal weight change. The advantage of this method is that the saturated salt slurries generate accurate and precise aw (or %RH) values as a function of temperature and, if made properly, act as “infinite aw sinks.” However, Levogeur and WilliamsCitation[3] have identified four main issues with employing the standard saturated salt slurry method: (1) the lengthy period of time it takes the material to achieve equilibrium; (2) the difficulty of obtaining conclusive, accurate measurements due to the protocol of continuously removing the sample from the chamber and exposing it to the environment, which is often at a different %RH, and possibly temperature, than the sample;Citation[4,Citation5] (3) the requirement of using large sample sizes (typically greater than one gram); and (4) the time-consuming, cumbersome steps required of the researcher to obtain weight measurements during the equilibration period. Additional drawbacks include the finite number of aw values available (only those associated with specific saturated salt slurries) and the potential mold growth problems during lengthy equilibration times at aw values ≥0.70.Citation[6]
These drawbacks associated with the saturated salt slurry method have, in large part, been overcome by the recent development of automated dynamic water vapor sorption instruments. A number of these automated instruments operate by continuously monitoring sample weight changes and controlling %RH at select temperatures by adjusting a mixture of dry and water vapor saturated gas streams using mass flow controllers. These instruments are usually programmed to automatically change the %RH in a stepwise fashion, progressively obtaining weight change data used for determining an isotherm on the same sample in a reduced amount of time (compared to the saturated salt slurry methodCitation[5]), while virtually eliminating sample handling by the researcher and exposure to room conditions during sample weight measurements. The sample is held at each selected %RH value until the sample weight does not change within a narrow, pre-specified tolerance level before moving to the next %RH value. Some commercially available instruments include the Dynamic Vapor Sorption (DVS) instrument from Surface Measurement Systems Ltd (Middlesex, UK), the IGAsorp from Hiden Analytical (Warrington. UK), the VTI Sorption Analyzer and the Q5000SA from TA Instruments (New Castle, DE, USA), the CI Sorp Water Sorption Analyzer from CI Electronics Limited (North Brunswick, NJ, USA), the SPS Moisture Sorption Analyzers from Projekt Messtechnik (Ulm, Germany), the HydrosorbTM 75 1000 Water Vapor Sorption Analyzer from Quantachrome Instruments (Boynton Beach, FL, USA), and the HygroGen 2—Precision Humidity and Temperature Generator from Rotronic (Hauppauge, NY, USA). As a group, these instruments and isotherms so obtained can be termed controlled atmosphere microbalances (CAM) and CAM isotherms. The interested reader is referred to a study by Rahman and Al-BelushiCitation[7] comparing the saturated salt slurry method (static) to the dynamic CAM method (specifically the Symmetrical Gravimetric Analyzer [Model 100] by VTI, Hialeah, FL, USA).
Another new automated dynamic water vapor sorption instrument was recently introduced, the AquaSorp Isotherm Generator (, Decagon Devices Inc., Pullman, WA, USA), which uses the Dynamic Dewpoint Isotherm (DDI) method for obtaining isotherms.Citation[8] The DDI method differs from the CAM isotherm methods described above, as the %RH of the gas stream in contact with the sample is not controlled. Rather, aw is directly measured using a chilled mirror dewpoint sensor, while changes in sample weight are tracked gravimetrically using a magnetic force balance. Adsorption (or wetting) is accomplished by saturating the air with water before it enters the sample chamber and desorption (or drying) is achieved by passing the air through desiccant before it enters the sample chamber, both at a user-selected flow rate.Footnote 1 After a small change in aw, approximately 0.015, airflow is halted and a snapshot of the sorption process is obtained by directly measuring sample aw and weight, which is subsequently converted to moisture content. DDIs are obtained very rapidly (e.g., several hours to a few days) because the sample is not required to equilibrate to a known %RH value; rather, the sample aw is directly measured at each successive adsorption or desorption point, allowing for the collection of a large number of aw points, often >75 data points, over a sizable aw range (0.03 to 0.95 aw) and temperature (15 to 40°C) range. Because samples are not required to come to equilibrium in the DDI method, the resultant isotherm may or may not be similar to the saturated salt slurry or CAM isotherms described above. Whether the resultant DDIs are similar to the saturated salt slurry or CAM isotherms depends on the nature of the sample (e.g., state of the material [crystalline or amorphous], particle size, porosity, density, and spatial distribution of the components), the water vapor diffusion rates into or out of the material being investigated (as influenced by the material properties and the selected flow rates), and the presence of any physical changes that may occur in the material, such as the glassy to rubbery transition, recrystallization, hydrate formation, and deliquescence.
Figure 1 Schematic diagram of the AquaSorp Isotherm Generator used to obtain Dynamic Dewpoint isotherms.
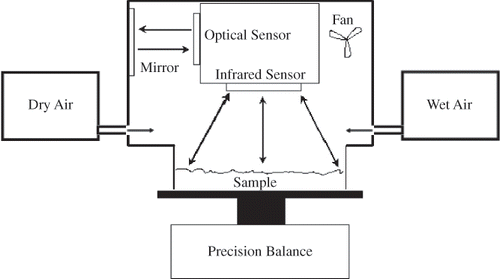
To date, a few published research papers have employed the DDI method to obtain sorption isotherms,Citation9–12 however, no publications were found that compare the DDI method to the standard saturated salt slurry isotherm method. Thus, the objective of this research was to obtain DDIs of five diverse food materials, dent corn starch, isolated soy protein, microcrystalline cellulose, crystalline sucrose, and corn flakes, and compare them to previously obtained saturated salt slurry isotherms using a desiccator (DES) and proximity equilibration cell (PEC) chambers.
MATERIALS AND METHODS
Materials
Five samples, normal dent corn starch (Tate & Lyle, Decatur, IL, USA), isolated soy protein (ADM, Decatur, IL, USA), microcrystalline cellulose (FMC Corporation, Philadelphia, PA, USA), crystalline sucrose (Sigma-Aldrich, St. Louis, MO, USA), and corn flakes (medium size whole flakes [average size 1.8 by 1.1 cm]; Kellogg's, Battle Creek, MI, USA), were used in this study “as is.” The crystalline sucrose particle size was measured using USA standard testing sieves (W.S. Tyler, Mentor, OH, USA), where 98.2% of the sucrose was greater than 425 μm and all particles were greater than 300 μm. Samples were stored in their original containers, and the containers were placed into sealable low water vapor transmission rate plastic bags at room temperature and relative humidity conditions.
The “as is” dent corn starch, isolated soy protein, microcrystalline cellulose, and corn flakes moisture contents were determined by placing triplicate samples in a vacuum oven (30 in. vacuum mercury) at 60°C for 24 h. The “as is” crystalline sucrose moisture content was determined in triplicate using Karl Fisher titration. The “as is” aw values of all five materials were determined in triplicate at 25°C using the AquaLab series 3 water activity meter (Decagon Devices, Pullman, WA, USA). The average “as is” moisture content values, aw values at 25°C, and associated standard deviations for each material are given in . In addition, the average starting DDI aw values, estimated by the AquaSorp Isotherm Generator (Decagon Devices Inc., Pullman, WA, USA) and associated standard deviations are also listed in . The DES and PEC methods were carried out by Yu,Citation[5] whereas the DDI method was carried out a few months later on the same lot of the materials (as stored in the lab as described above).
Table 1 Average “as is” moisture content and AquaLab measured aw values and associated standard deviations at 25°C for the materials used in the DES and PEC and the DDI methods.Footnote* The average starting DDI aw values for both adsorption and desorption parts of the working isotherm, estimated by the AquaSorp Isotherm Generator, and associated standard deviations are also listed. Visually observed mold growth for some of the materials in both the DES and PEC chambers is also indicated. No visual mold growth was observed in the DDI method
Methods
Saturated salt slurry method in DES and PEC chambers
Saturated salt slurry working isotherms were obtained in triplicate for 5 materials using both DES and PECCitation[13] chambers at 12 saturated salt slurry aw values: 0.064, 0.113, 0.225, 0.328, 0.432, 0.576, 0.689, 0.753, 0.843, 0.903, 0.936, and 0.973 at 25°C.Citation[5] All saturated salt slurry aw values were from Greenspan,Citation[14] except for 0.903, which was from Young.Citation[15] Samples (1 to 2 g) were weighed at 7-day intervals until each sample did not change by more than 2 mg/g dry weight for two successive weighing times.Citation[16] The average time for a material to reach equilibrium was weeks to months in the DES chambers and days to weeks in the PEC chambers. For example, it took between 2 and 10 weeks to obtain isolated soy protein and corn flake isotherms using the DES chamber and between 1.5 and 2 weeks using the PEC chamber, with specific times dependent upon material type and saturated salt slurry aw value. After the equilibrium weight had been reached, the percent dry basis (%db) equilibrium moisture content was calculated using the initial and final weight readings and the initial measured moisture content values (). Mold growth was visually observed for some of the materials in both DES and PEC chambers and is recorded in . Since weight change data from samples with mold growth are unreliable,Citation[6] these data were excluded from the DES and PEC isotherm plots. No visual mold growth was observed in the DDI method.
DDI Method
The Dynamic Dewpoint isotherms (DDIs) were obtained using the AquaSorp Isotherm Generator (Decagon Devices Inc., Pullman, WA, USA). Before the DDIs were obtained, the aw dewpoint sensor was verified using two unsaturated aqueous salt solutions purchased from the instrument manufacturer, 13.41 molal LiCl (0.250 ± 0.003 aw) and 6.0 molal NaCl (0.760 ± 0.003 aw). Working DDIs were obtained for each material (1 to 2 g in a stainless steel sample cup half filled, except for crystalline sucrose where the sample covered the bottom of the cup; internal radius 1.87 cm, internal height 0.973 cm, and sample volume 10.7 cc) between 0.10 to 0.95 aw (except for crystalline sucrose that was brought to 0.93 aw) using the start from current aw feature (AquaSorp firmware version ASIG 1.19, Decagon Devices, Pullman, WA, USA) with a 300 ml/min flow rate at 25°C. The adsorption and desorption sections of the working isotherm were obtained in triplicate, each on a different sample. The average DDI starting aw and associated standard deviation for each material for both adsorption and desorption parts of the working isotherm are given in . Weight change at each aw was converted to moisture content (%db) using the SorpTrac software (version 1.03.3, Decagon Devices, Pullman, WA, USA) using the initial measured moisture content value (). The average DDI starting aw is given since overlap sometimes occurs between the adsorption and desorption isotherm sections of the DDI. This overlapFootnote 2 may be attributed to samples being run continuously, leaving a “humidity memory” in the sample chamber, which sometimes affects the initial conditions of the subsequent sample.
RESULTS AND DISCUSSION
Triplicate measurements were plotted for all isotherm methods (except for crystalline sucrose, where only a single DDI was plotted, since plotting all three DDI, which fell right on top of each other, made it impossible to observe the higher aw DES isotherms points), so the variation in the isotherm methods could be visually assessed. Statistical analysis was not carried out between the two isotherm methods for all five materials, since, as discussed previously, the DDI method does not always yield an isotherm that is comparable to traditional equilibrium isotherms; thus, the DDI method should be viewed as a unique isotherm method.Citation[8,Citation17] As a unique isotherm method, some advantages as well as limitations are expected. In regards to its limitations, for example, in the case where the DDI is not comparable to the traditional equilibrium isotherm, isotherm equations, such as the BET and GAB, can still be applied to the DDI data; however, the resultant parameters (e.g., the monolayer moisture content value) are probably not comparable to the isotherm equation parameters associated with the equilibrium isotherm data.
In regards to its advantages, the DDI provides a new opportunity to probe time dependent water sorption-related material properties, such as the glassy to rubbery transition, recrystallization, hydrate formation, and deliquescence. In cases where the DDIs are similar to the saturated salt slurry isotherms, statistical analysis may be done. However, since the saturated salt slurry method yields discrete aw points and the DDI method near continuous aw points with each run yielding a unique set of aw values, formal statistical analysis is not possible; rather the DDI moisture content values may be globally compared to the calculated and, subsequently, connected confidence interval for the discrete DES and PEC isotherm moisture content values. In this study, a global statistical comparison between the two isotherm methods was done for microcrystalline cellulose.
Dent Corn Starch
The dent corn starch DDI () exhibited similar sorption behavior to its corresponding saturated salt slurry isotherms. This indicates that dent corn starch exhibits relatively fast water vapor diffusion; that is, water vapor penetrates rapidly into or out of the entire sample yielding a DDI that is comparable to the DES and PEC saturated salt slurry isotherms. There, however, appears to be a slight difference between the dent corn starch DDI and the DES and PEC isotherms near 0.576 aw, indicating that a time dependent change in the sorption process may be occurring in the dent corn starch near this aw. It is interesting to note that an earlier DDI run, done on the same dent corn starch, did not exhibit this mid-isotherm dip.Citation[8] The appearance of the mid-isotherm dip in some DDI runs and not others, further suggests the occurrence of some type of time dependent physical change in the material that is perhaps related to the initial starting conditions of the material (e.g., moisture content and aw) and/or the age and history of the sample. The exact nature of this time dependent physical change is difficult to identify, since native (raw) dent starch is a complex biomaterial, composed of granular structures containing both amylose and amylopectin polymers, with both crystalline and amorphous regions. Pregelatinized starch (no crystalline regions) may be a better material choice to use for comparing these isotherm methods and for further investigation into the nature of the slight difference in the isotherms obtained by these two methods.
Isolated Soy Protein
The isolated soy protein DDI () was similar to the corresponding saturated salt slurry isotherms, however, the DDI exhibited slightly lower moisture contents near 0.432 aw. This difference in the isotherms may be attributable to the occurrence of a glassy to rubbery transition in the amorphous portion of the isolated soy protein. However, additional research is required to confirm this hypothesis. The Savitsky Golay second derivative methodCitation[18] was applied to the DDI aw data,Citation[17] yielding 0.458 as the critical aw (or critical %RH) at the experimental temperature, 25°C. If our hypothesis is correct, this critical aw corresponds to the glass transition temperature of the material for the experimental conditions employed. However, it is important to keep in mind that the critical aw at which the glassy to rubbery transition occurs at a given temperature (the temperature of the experiment that corresponds to the glass transition temperature) will most likely be dependent on the time scale under which the DDI measurements are obtained (e.g., flow rate and aw measurement time) as well as the nature of the sample. The difference in the measurement time between the DES and PEC (long time) and the DDI (short time) isotherms may allow changes in the physical state of the material to occur to different extents, which are then reflected in the isotherms produced by the two different methods. The low data resolution in the standard saturated salt slurry methods makes it very difficult to observe these sorption driven transitions. Further investigation into the relationship between the critical aw as determined via the DDIs and the glassy to rubbery transition is currently being carried out using polydextrose as a model system.Citation[19]
Microcrystalline Cellulose
The microcrystalline cellulose DDI () exhibited similar sorption behavior to its corresponding saturated salt slurry isotherms, indicating that microcrystalline cellulose exhibits relatively fast water vapor diffusion. Since the DDI appears similar over the entire aw range to the saturated salt slurry DES and PEC isotherms, a global statistical comparison of oneFootnote 3 of the working DDIs to the connected upper and lower 99% DES/PEC confidence limits (±0. 506 mc %db) is shown in . As can be seen in , the DDI data remained in between or on (although, in general, nearer to the lower confidence limit) the 99% DES/PEC confidence limits, except for the last two high aw DDI points, indicating that overall, the DDI, in the case of MCC, was the same as the DES and PEC isotherms. It is not possible to carry out a formal statistical analysis since the saturated salt slurry method yields discrete aw points and the DDI method near continuous points with each run yielding a unique set of isotherm points.
Figure 4 DES, PEC, and DDI isotherms for microcrystalline cellulose (color figure available online).
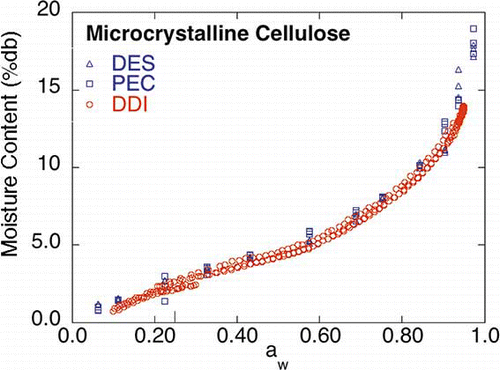
Figure 5 Informal statistical comparison of one of the working DDIs to the connected upper and lower 99% DES and PEC confidence limits (±0. 506 mc %db) (color figure available online).
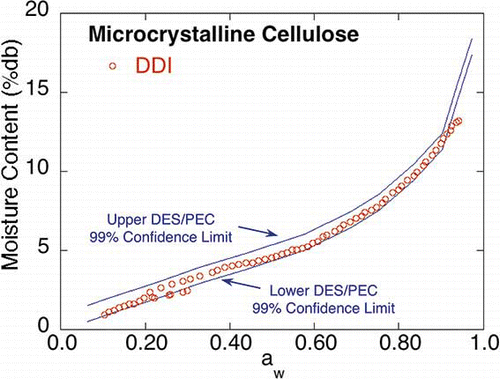
It is interesting to note that for microcrystalline cellulose the two sections of the working isotherm that overlapped (the desorption and the adsorption sections) were further apart than for the other materials; that is, the moisture contents were slightly different at the same aw (around the 0.20 to 0.30 aw range). Further investigation is needed to determine the cause of this gap. However, it is also interesting to note that the moisture contents for the DES and PEC isotherms in the 0.20 to 0.30 aw range (0.225 aw) also exhibited a larger variation, compared to the moisture contents at other nearby aw values.
Crystalline Sucrose
The crystalline sucrose DDI () was also similar to the corresponding saturated salt slurry isotherms; however, the DDI was able to completely capture the deliquescence process, whereas the saturated salt slurry method only allows for isotherm values to be obtained at a few discrete aw values. The deliquescence point, as determined by taking the average of the aw values before and after the turning point, was 0.852 ± 0.001 at 25°C, which is virtually the same as the value reported by Salameh and othersCitation[20] of 0.85 at 25°C obtained by extrapolation using a VTI Sorption Analyzer (VTI, Hialeah, FL, USA). Comparing the deviation of the upper vertical DDI to the saturated sucrose solution aw and moisture content values plotted in shows that during the extensive water sorption that accompanies the deliquescence process, a slightly less than saturated sucrose solution layer begins to form, which is subsequently measured by the dewpoint sensor. This is due to the dynamic, diffusion-limited nature of the DDI measurement method. Despite the formation of this less than saturated sucrose solution layer, the DDI method provides one of the most insightful tools for observing the deliquescence process, as well as one of the most accurate means of determining the deliquescence point. Further discussion of the use of the DDI method to determine the deliquescence aw (or %RH) is given in Yao and others.Citation[12]
Figure 6 DES, PEC, and DDI isotherms for crystalline sucrose. The deliquesce point and the literature saturated sucrose solution aw (at 25°C from Salameh et al.Citation[20]) and associated moisture content (at 25°C from Bubnick and ZadlecCitation[21]) are indicated on the figure. Mold growth aw limits for DEC and PEC methods are given in (color figure available online).
![Figure 6 DES, PEC, and DDI isotherms for crystalline sucrose. The deliquesce point and the literature saturated sucrose solution aw (at 25°C from Salameh et al.Citation[20]) and associated moisture content (at 25°C from Bubnick and ZadlecCitation[21]) are indicated on the figure. Mold growth aw limits for DEC and PEC methods are given in Table 1 (color figure available online).](/cms/asset/53fd5ca8-b09b-4860-9996-76238bdcb0ff/ljfp_a_478323_o_f0006g.jpg)
Corn Flakes
The corn flake DDI () was substantially different than the corresponding saturated salt slurry isotherms in the aw region between 0.40 and 0.70. The Savitsky GolayCitation[18] second derivative yielded 0.673 as the critical aw at the experimental temperature, 25°C. The difference in the isotherms is attributed to slow diffusion of water into (absorption) the very dense laminated corn flake matrix, which shows up as the difference between the rapidly obtained DDI and the more slowly obtained equilibrium DES and PEC isotherms. The location of the gap between the DES and PEC and DDI isotherms is most likely associated with an aw-dependent permeability of the very dense laminated corn flake matrix, where the large width of the gap is attributed to the time-dependent nature of the DDI sorption process. At the critical aw, the permeability ceases to be a limiting water sorption factor and the DDI isotherm again agrees with the DES and PEC isotherms since additional time is no longer needed for the water to diffuse through the laminate surface of the corn flake. Increasing the surface permeability or decreasing the path length of diffusion should decrease the gap between the isotherms. Shands and LabuzaCitation[22] recently illustrated these effects by comparing the DDI of whole, coarsely ground, and finely ground corn flakes to the static saturated salt slurry isotherm method. They reported that grinding the corn flakes increased the surface area available for water sorption and decreased the diffusion distance, resulting in the ground corn flake DDIs being similar to the static saturated salt slurry desiccator isotherm method.
Figure 7 DES, PEC, and DDI isotherms for corn flakes. Mold growth aw limits for DEC and PEC methods are given in (color figure available online).
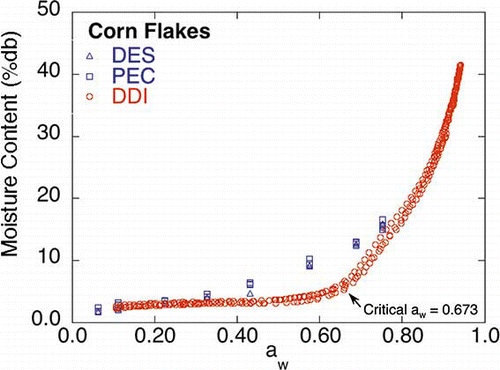
Below 0.40 aw, it is hypothesized that water is mainly being adsorbed onto (adsorption section of the working isotherm) or desorbed from (desorption section of the working isotherm) the surface of the corn flakes, following Fickian diffusion (as the corn flake exhibits a type III or “J” shaped isotherm) in each of the working isotherm methods, resulting in very little difference in moisture content.Citation[23] As aw increases above 0.40, permeability of the laminate surface becomes sufficient for increased adsorption of moisture if given sufficient time (the time required being dependent on the permeability and, hence, the aw). Consequently, over the aw range of 0.40 to 0.70, DES and PEC isotherms adsorb more water than the DDI, since the DES and PEC methods provide sufficient time to allow moisture movement beyond the surface and into the corn flake matrix. The adsorption of moisture for the DDI method will continue to lag behind until the permeability is high enough (at 0.673 aw under the conditions of the current experiment) to allow rapid diffusion of moisture into the matrix. At this point, diffusion of water into the corn flake matrix is fast enough that the DDI and DES and PEC methods are once again in agreement (mold growth at aw values ≥0.843 in the DES and PEC methods precludes additional higher aw comparisons). It is reasonable to assume under this scenario that anything that increases diffusion into the sample, such as increasing permeability or decreasing particle size (results discussed above[22]) or decreased flow rate, will increase the amount of water sorbed by the sample in the DDI method and result in better agreement between the sorption methods. These results also suggest the possibility of further investigating the aw dependency of permeability by utilizing a combination of equilibrium and DDI methods.
CONCLUSIONS
In general, the DDIs exhibited similar sorption behavior to the saturated salt slurry isotherms for the dent corn starch, isolated soy protein, microcrystalline cellulose, and crystalline sucrose, where relatively minor, but noteworthy, deviations were discussed. The DDIs differed more dramatically from the saturated salt slurry isotherms for corn flakes in the aw transition region, between 0.40 and 0.70 aw. This difference was attributed to slow diffusion of water vapor into the very dense laminated corn flake matrix. The benefits of the DDI method compared to the saturated salt slurry isotherm method are: (1) increased analysis speed, a few days compared to weeks or months; (2) a dramatic increase in data point resolution, with often >75 data points per isotherm; (3) prevention of mold growth problems experienced for some materials at high aw values (mm); and (4) the opportunity to explore sample specific, time dependent water sorption-related material properties, such as the glassy to rubbery transition, recrystallization, hydrate formation, and deliquescence. Along with its benefits, the non-equilibrium nature of the DDI method also has its limitations, including: (1) the resultant DDI not always being equal to the equilibrium saturated salt slurry isotherm (as observed for the corn flakes herein); and (2) in the case where limitation one applies the resultant DDI isotherm equation parameters (e.g., obtained by applying the BET or GAB equation to the DDI data) are not equivalent to those equation parameters obtained for the equilibrium isotherm. This last limitation is important since moisture sorption isotherms are frequently used to obtain the monolayer moisture content value. Based on the initial DDI results reported herein, additional, more in-depth experiments are currently being carried out on these and other food materials. Specially, the DDI method is being investigated for its promise as a new method for determining the relative humidity at which the glassy to rubbery transition takes place at a fixed temperature.
ACKNOWLEDGMENTS
This project was supported in part by the USDA National Institute of Food and Agriculture, Hatch project number 385. In addition, the authors gratefully acknowledge the financial support of the 2008 Marcel Loncin Research Prize from the Institute of Food Technologists and the 2008 Midwest Advanced Food Manufacturing Alliance (University of Nebraska-Lincoln) grant. The helpful scientific discussions with Brady Carter of Decagon Devices (Pullman, WA, USA) and Dr. Anthony J. Fontana, Jr. of Silliker, Inc. (Homewood, IL, USA) are also gratefully acknowledged.
Notes
1The user selected flow rate can range from 10 to 1000 ml/min, where a lower flow rate allows more time for moisture penetration.
2Subsequent to the data collected herein, to minimize this overlap the manufacturer now suggests conditioning the sample chamber between sample runs by running an activated charcoal sample. In addition, the manufacturer added a 30 min stabilization step before the sorption run begins.
3For clarity purposes, only one microcrystalline cellulose DDI was plotted. However, the other two microcrystalline cellulose DDIs behaved similarly.
REFERENCES
- Schmidt , S.J. 2004 . “ Water and solids mobility in foods ” . In Advances in Food and Nutrition Research , Edited by: Taylor , S. Vol. 48 , 1 – 101 . London , UK : Academic Press .
- Damodaran , S , Parkin , K and Fennema , O.R. 2008 . Fennema's Food Chemistry , 4th , 1144 Boca Raton , FL : CRC Press .
- Levogeur , C.L. and Williams , D.R. The Characterization of Pharmaceutical Materials by Dynamic Vapor Sorption , London , UK : Application Note 101. SMS .
- Bohn , D.M. 2004 . The use of dynamic vapor sorption techniques to investigate the release of volatile flavor in amorphous sucrose glasses. Ph.D. Dissertation , Urbana , Illinois : University of Illinois at Urbana-Champaign .
- Yu , X. 2007 . Investigation of moisture sorption properties of food materials using saturated salt solution and humidity generating techniques , Urbana , Illinois : Ph.D. Dissertation, University of Illinois at Urbana-Champaign .
- Yu , X. , Martin , S.E. and Schmidt , S.J. 2008 . Exploring the problem of mold growth and the efficacy of various mold inhibitor methods during moisture sorption isotherm measurements . Journal of Food Science , 73 ( 2 ) : E69 – E81 .
- Rahman , M.S. and Al-Belushi , R.H. 2006 . Dynamic isopiestic method (DIM): Measuring moisture sorption isotherm of freeze-dried garlic powder and other potential uses of DIM . International Journal of Food Properties , 9 ( 3 ) : 421 – 437 .
- Carter , B. and Fontana , A. 2008 . Dynamic Dewpoint Isotherm versus Other Moisture Sorption Isotherm Methods , Pullman , WA : Application Note. Decagon Devices .
- Smith , W.A. , Thompson , D.N. , Thompson , V.S. , Radtke , C.W. and Carter , B. 2009 . Assessment of xylanase activity in dry storage as a potential method of reducing feedstock cost . Applied Biochemistry and Biotechnology , 154 : 287 – 301 .
- Merkh , G. , Dambacher , M. and Isengard , H.D. 2009 . Rapid moisture measurement with microwave resonance technology in infant formulas . Journal of Agroalimentary Processes and Technologies , 15 ( 1 ) : 4 – 11 .
- Spackman , C.C.W. and Schmidt , S.J. 2010 . Characterization of sugar gum paste by water sorption isotherm behavior and texture analysis . Food Chemistry , 119 ( 2 ) : 490 – 499 .
- Yao , W. , Yu , X. , Lee , J. , Yuan , X. and Schmidt , S.J. 2011 . Measuring the deliquescence point of crystalline sucrose as a function of temperature using an automatic isotherm generator . International Journal of Food Properties , 14 ( 4 ) : 882 – 893 .
- Lang , K.W. , McCune , T.D. and Steinberg , M.P. 1981 . A proximity equilibration cell for rapid determination of sorption isotherms . Journal of Food Science , 46 : 936 – 938 .
- Greenspan , L. 1976 . Humidity fixed points of binary saturated aqueous solution . Journal of Research of the National Bureau of Standards—A Physics and Chemistry , 81A ( 1 ) : 89 – 96 .
- Young , J.F. 1967 . Humidity control in the laboratory using salt solution—A review . Journal of Applied Chemistry , 17 : 241 – 245 .
- Bell , L.N. and Labuza , T.P. 2000 . Moisture Sorption—Practical Aspects of Isotherm Measurement and Use , 2nd , 122 American Association of Cereal Chemists, Inc.: St. Paul, MN .
- Fontana , A.J. April 2008 . “ Investigating the moisture sorption behavior of foods using a dynamic dewpoint isotherm method ” . In Euro Food's Water—5th Conference on Water in Food , April , 6 – 8 . Germany : Nürtingen .
- Savitsky , A. and Golay , M.J.E. 1964 . Smoothing and differentiation of data by simplified least squares procedures . Analytical Chemistry , 36 : 1627 – 1639 .
- Yuan , X. , Schmidt , A.R. and Schmidt , S.J. June 2009 . “ Investigating the use of automatic water vapor sorption instruments for the determination of the critical relative humidity at which the glassy to rubbery transition occurs in amorphous food materials ” . In 70th Annual Institute of Food Technologists June , 6 – 9 . Anaheim , CA
- Salameh , A.K. , Mauer , L.J. and Taylor , L.S. 2006 . Deliquescence lowering in food ingredient mixtures . Journal of Food Science , 71 ( 1 ) : E10 – E16 .
- Bubnik , Z. and Kadlec , P. 1995 . “ Sucrose solubility ” . In Sucrose Properties and Applications , Edited by: Mathlouthi , M. and Reiser , P. 107 Glasgow : Blackie Academic and Professional . 1995
- Shands , J. and Labuza , T.P. June 2009 . “ Comparison of the new dynamic dewpoint method to the static and dynamic vapor sorption methods for the generation of moisture sorption isotherms ” . In 70th Annual Institute of Food Technologists , June , 6 – 9 . CA : Anaheim .
- Peppas , N. and Brannon-Peppas , L. 1994 . Water diffusion and sorption in amorphous macromolecular systems and foods . Journal of Food Engineering , 22 : 189 – 210 .