Abstract
The effects of high hydrostatic pressure (483 and 676 MPa at 20°C for 5 min) and heat treatment (63°C for 30 min and 72°C for 15 s) of milk on the rheological properties of coagulant-induced milk gels were investigated. The rheological properties of gels were determined using a dynamic Physica Rheometer and Texture Analyzer. Milk turbidity was measured by a spectrophotometer. The gels produced from a pressure of 483 MPa had higher storage modulus (G*) and firmer gel at cutting compared to heated milks. Increasing the pressure to 676 MPa caused a reduction in G*, less firm gels, and an increase in milk turbidity compared to 483 MPa and heated milks. The differences between the gels formed from different pressure- and heat-treated milks, suggesting that the structure network of gels were considerably altered by high hydrostatic pressure treatment of milk. Disruption of casein micelles and denaturation of whey proteins caused by high hydrostatic pressure treatments were responsible for most of the effects observed in this study. In general, pressurizing the milk at 483 MPa at 20°C was a promising treatment for the manufacture of coagulant-induced milk gels with good rheological properties; however, pressurization at 676 MPa could not be recommended.
INTRODUCTION
High hydrostatic pressure (HHP) has been known as a potential food preservation method for over a century. A considerable amount of information has become available in the last 20 years demonstrating increasing interest in HHP processing in food production.[Citation1,Citation2] Interests have increased since recent progress in the engineering of large-scale equipment has allowed this technology to be adapted to the needs of the food industry.[Citation2,Citation3] Several articles pertaining to inactivation of microorganisms and preservation of milk products have been published.[Citation4–6] In addition to microbial destruction, HHP caused changes in milk components, particularly milk proteins, which may lead to modifications in the quality of the resultant milk product.[Citation7–10] Studies showed that casein micelles were disrupted and disintegrated and whey proteins, particularly β-lactoglobulin, were denatured and aggregated when milk was subjected to HHP treatment.[Citation11–13] Additionally, HHP caused changes in milk properties that enhance enzymatic milk coagulation, improved gel firmness and cheese yield, increased water holding capacity of acid gels, and changed light scattering of milk compared to normal milk.[Citation8,Citation14–19]
Heat treatments in the range of 63–75°C are widely used as unit operations in the manufacture of cheese products because they considerably reduce the microbial load in milk without profoundly changing the structure of whey proteins.[Citation20,Citation21] On the other hand, excessive heat treatments may bring about undesirable changes in flavor and loss of nutrients.[Citation11] Heating milk above 75°C induced denaturation of whey proteins and their complex formation with casein micelles involving κ-casein[Citation22,Citation23] without changes in the casein structure and stability.[Citation24,Citation25] Alternatively, HHP treatment reduces the microbial load without influencing flavor or nutrients. However, modifications in whey proteins and caseins induced by HHP may affect rheological properties of milk gels.
Coagulation of milk is a fundamental processing technique employed in the manufacture of cheese.[Citation26] The casein network is responsible for the formation of coagulant-induced milk gels;[Citation27] caseins contribute to gel strength during the coagulation process and provide firmness to the gel at cutting.[Citation28] Changes in milk proteins caused by heating have been studied from various physical aspects (e.g., viscosity, gel formation, and strength) by many researchers.[Citation29–33] However, limited studies[Citation8,Citation12,Citation17] have reported the relationship between pressure-induced denaturation of milk proteins and changes in coagulation time, characteristics, and gel firmness that are important in the processing of cheese. The objective of the present study was to investigate the effects of HHP (483 and 676 MPa at 20°C for 5 min) and heat treatment (63°C for 30 min and 72°C for 15 s) of milk on the rheological properties of coagulant-induced milk gels. Additional information about the turbidity of the milk after treatments was obtained as an indirect tool to quantify gel structure formation.
MATERIALS AND METHODS
Materials
Raw cow's milk was obtained fresh on a daily basis from Washington State University Creamery (Pullman, WA, USA). The milk was standardized with cream (40 g fat/100 milk) addition to obtain 4.0 g ± 0.1 fat/100 milk and 3.35 g ± 0.05 protein/100 milk. The milk was kept at 4°C for no more than 2 h prior to treatment. Anti-microbial agent, sodium azide (Sigma-Aldrich Chemicals Co., Steinheim, Germany), was added (0.1 g/100 milk) to all milk samples before storing in the cold store at 4°C to minimize proliferation of microorganisms during storage and coagulation. Sodium azide did not affect the coagulant-induced coagulation of milk.[Citation34]
High Hydrostatic Pressure Treatment (HHP)
Standardized milk samples (1000 ml) were poured into lay-flat polyethylene tubing (4 mm, No. 89986LR; Consolidated Plastic Co., Twinsburg, OH, USA) and sealed without headspace. The milk samples were pressurized at 483 or 676 MPa utilizing a high-pressure unit (Engineered Pressure System, Wilmington, MA, USA). Both pressure treatments were conducted at 20°C. The pressure was raised to the desired value at a rate of 2.16 MPa/s, and then maintained for 5 min. These pressure levels were chosen because milks treated at pressures up to 500 MPa have been shown to have a shelf life at least equivalent to pasteurized milk.[Citation6]
Batch Heat Treatment (BHT)
Standardized milks (1000 ml) were heated to 63°C, maintained at this temperature for 30 min, with continuous rocking, in a thermostatically controlled water bath (VWR Co., Batavia, IL, USA). After heat treatment, the milk samples were rapidly cooled by immersion in ice water until the temperature was below 10°C.
Continuous Heat Treatment (CHT)
Standardized milk was pasteurized in a plate heat exchanger (Y1991; Alfa Laval, Lund, Sweden) at 72°C at a flow rate of 9000 l/h, with a holding time of 15 s and cooling to 4°C.
Rheological Measurements
Small-strain oscillatory shear measurements were performed on a Physica Rheometer (Physica-Rheolab MC 20/UM; Physica USA, Inc., Spring, TX, USA) using the Z1 DIN concentric cylinders. The measuring geometry consisted of two coaxial cylinders; the outer and inner radius of the measuring bob are 2.35 and 2.275 cm, respectively; the outer and inner of the measuring cup are 2.40 and 2.25 cm, respectively; and the measuring length is 11.1 cm. The rheometer was driven by a microprocessor, and the temperature was maintained at 35 ± 0.1°C by a circulating water bath.
A double strength microbially-derived cheese coagulant, CHYMAX (Chr. Hansen's Laboratory Inc., Milwaukee, WI, USA) was diluted 40 times; the dilution was selected based on the setting time at the WSU Creamery. Upon addition of coagulant solution (2 ml) to the milk (300 ml) at 35°C, the milk was stirred for 30 s and then 17 ml of the milk was transferred into the cylinder of the rheometer. To prevent evaporation, paraffin oil was layered on the surface of the milk in the rheometer cup. The gel was subsequently formed in situ.
The rheometer was programmed to incubate the milk for 650 s, and then three measurements were conducted as follows: The initial measurements involved monitoring gelation as the samples were oscillated at a frequency of 1 Hz and 0.7 mNm torque. Measurements were taken every 22 s for 11 min. After gel formation, the rheological properties of the set gel were determined by varying the frequency from 1 to 10 Hz at 0.7 mNm torque. Measurements were taken every 10 s for 3.3 min. Oscillatory measurements, torque sweeps, were made over the torque range of 0.7 to 50 mNm at a frequency of 1 Hz. Measurements were taken every 4 s for 2 min. During the measurements, the temperature of the samples was maintained at 35 ± 0.5°C. Three rheological functions, storage modulus (G*), loss modulus (G**), and loss tangent (tan δ), were obtained in triplicate.
Gel Firmness Measurement
A 90-ml volume of milk plus coagulant was placed in a 400-ml glass beaker in a controlled temperature, covered water bath (VWR Co., Batavia, IL, USA) to maintain the coagulation temperature in the beaker (35 ± 0.1°C). The firmness of the gel was measured after exactly 22 min using the Texture Analyzer type TA-XT2i (Stable Micro System, Surrey, England) equipped with a 2-kg compression load cell. A specially designed transparent rigid plastic test cell with an outside diameter of 2.5 cm and length of 3.5 cm and a cylindrical rod made of stainless steel with a diameter of 1.5 cm and length of 10 cm were attached to the load cell. At a speed of 2 mm/s, the test cell penetrated the curd only once, to a distance of 0.5 cm below the surface. The 400-ml beakers used to hold the coagulum were selected to have the same inside diameters (8 cm) so that the distance between the test cell and the inside wall of the beaker was always 5.5 cm. The gel firmness was measured as the amount of force in grams using a digitizer connected to a computer. Values of the firmness of the gel were generated over nine measurements.
Turbidity and pH Measurements
Turbidity of milk samples[Citation35] was measured by using an Ultrospec 4000 UV/visible spectrophotometer (Pharmacia Biotech, Alameda, CA, USA). The spectrophotometer was driven by a microprocessor and the temperature was held at 20 ± 0.5°C during measurements. Three milliliters of diluted milk (0.1 ml of milk + 25 ml of distilled water) at 20°C was placed in a plastic cuvette and inserted into the cuvette holder. The turbidity was determined by monitoring optical density at 440 nm. The pH value of the milk samples was determined by immersing the Orion Research (Cambridge, MA, USA) Model 601A/digital analyzer pH electrode into the milk samples. Both measurements were conducted after 10 min of pressure treatment.
Statistical Analysis
Statistical differences for the gel firmness and the turbidity of milk were determined using analysis of variance ANOVA.[Citation36] When significant (p ≤ 0.05), Tukey's least significant differences test was used to separate treatment means.
RESULTS
The development of a milk casein network during gelation can be monitored by changes in viscoelasticity using the dynamic oscillatory method.[Citation37] The rheological properties including the storage modulus (G*), a measure of the elastic component of the network, and loss modulus (G**), a measure of the viscous components[Citation38] during gelation of HHP and heat treatment (HT) of milks, were monitored. In all cases, except gel made from milk treated with HHP at 676 MPa, a sharp increase in G* and G** gave rise to a well-formed gel network. The gel obtained from HHP at 483 MPa had the highest G* value followed by CHT, BHT, and HHP at 676 MPa gels, respectively (). The increase of G** paralleled that of G′ but showed a slightly lower value (data not shown).
Figure 1 Storage Modulus G* as function of Time for rennet-induced milk gels. (a) Milks were pressurized at 483 MPa (▼) or 676 MPa (Δ). (b) Milks heated at BHT (Δ) or CHT (▼).
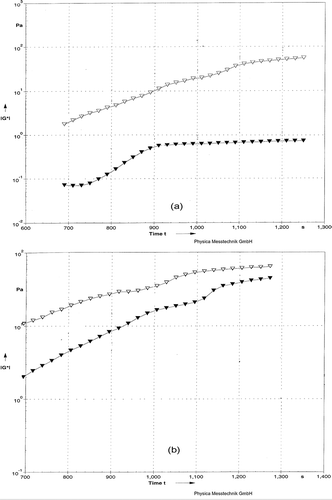
The effects of HHP and HT on tan δ during coagulation are presented in . As tan δ values represent a combination of G* and G** (tan δ = G**/G*), it is a direct measure of the relative importance of the viscous (G** high values) and elastic (G* high values) effect in the milk gel network. During gel formation, there was no major change in the tan δ values as a function of time in all samples, suggesting that more elastic than viscous structure was produced with time of coagulation. The tan values for gels made with milk treated with HHP at 483MPa were higher than the other samples, indicating a more elastic network. The differences in the tan δ value between the HHP and HT samples within the linear viscoelastic region indicate that the nature and type of interaction forces were similar but strength of elasticity varied.
Figure 2 Storage Modulus G* as function of Frequency for rennet-induced milk gels. (a) Milks were pressurized at 483 MPa (▼) or 676 MPa (Δ). (b) Milks heated at BHT (Δ) or CHT (▼).
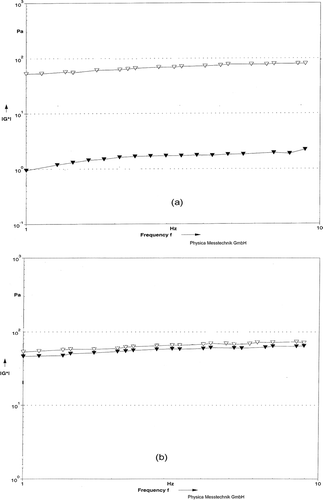
The rheological properties of the HHP and HT gel networks were examined by conducting frequency sweep tests. Frequency sweep data for the two parameters (G* and tan δ) were described as linear relationships between parameter and frequency. Representative curves for HHP and HT gels are given in and . The existence of nearly straight curves to all of these lines reflects the viscoelastic nature of the gel networks obtained (). A straight line, in which G* is independent of frequency, will result if an elastic network has been obtained.[Citation39] Within the linear viscoelastic region, the gel obtained from HHP at 483 MPa had the greatest G′, followed by CHT, BHT, and HHP at 676 MPa gels, respectively. Similarly, within the linear viscoelastic region, the tan δ values of the gels were not significantly different, except the gel obtained from HHP at 676 MPa (). It should be noted that even for these gel networks, the contribution of the elastic components was greater than the viscous components as evidenced by the fact that the tan δ values were less than one, except for the HHP at 676 MPa gel. The line of the latter gel suggests that the network had a more viscous character at low and high frequencies and a less elastic character in the intermediate frequencies.
Figure 3 Storage Modulus G* as function of Torque for rennet-induced milk gels. (a) Milks were pressurized at 483 MPa (▼) or 676 MPa (Δ). (b) Milks heated at BHT (Δ) or CHT (▼).
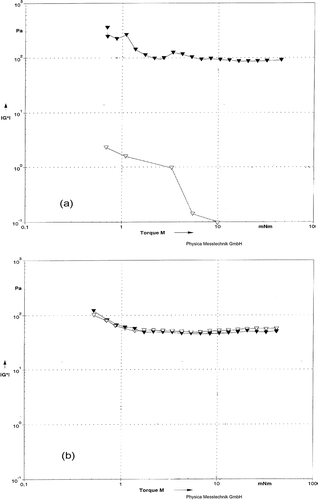
Torque sweeps have been used to differentiate weak and strong milk gels; the strong gels may remain in the linear viscoelastic region over greater strains than weak gels.[Citation40] In order to gain some information about gel strength after gel formation, the gels of milk treated by HHP and HT were evaluated over a range of small torques (). There were no major differences in the shapes of the G* lines for the HHP at 483 MPa, CHT, and BHT (). Structural degradation occurred at some points in the torque range in the gel prepared from HHP at 676 MPa. Similar trends were seen for the tan δ (). After milk was treated with 676 MPa, there was an increase in tan δ for milk gel, indicating a very weak network due to the viscous behavior. An increase in tan δ means a relatively less elastic and more viscous like behavior, with spontaneous breaking of bonds.[Citation41] The time scale of dynamic measurements is roughly the inverse of the angular torque; this implies that with increasing torque, bonds spontaneously break within short time. These levels of HHP treatment corresponded to the micro-structural transition from unstructured mass to a cross-linked network.
Figure 4 Loss tangent as function of Time for rennet-induced milk gels. (c) Milks were pressurized at 483 MPa (▼) or 676 MPa (Δ). (d) Milks heated at BHT (Δ) or CHT (▼).
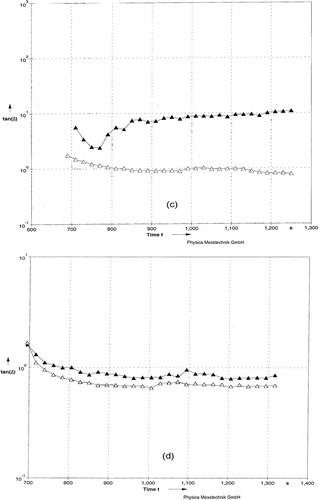
Figure 5 Loss tangent as function of Frequency for rennet-induced gels. (c) Milks were pressurized at 483 MPa (▼) or 676 MPa (Δ). (d) Milks heated at BHT (Δ) or CHT (▼).
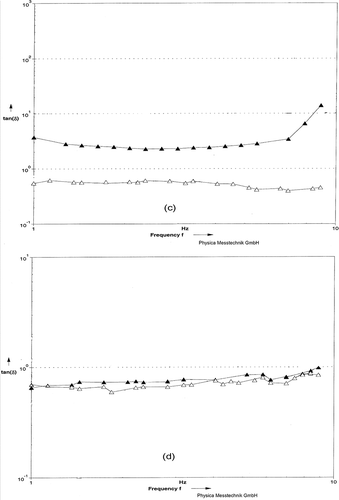
The firmness of the coagulant-induced milk gel at cutting is considered one of the main factors that influence cheese characteristics, particularly cheese yield.[Citation42] The effects of HHP and HT on gel firmness at cutting are shown in . The gels made from CHT milk and milk treated by HHP at 483 MPa had higher firmness (p < 0.05), compared to gels obtained from raw and BHT milk and milk treated with HHP at 676 MPa. The gel strength (G′) values of HHP and HT milk gels obtained over the range of torques appeared to be related to the gel firmness data of the same milks conducted in the Texture Analyzer ().
Table 1 Mean absorbance of milk and mean firmness (G′) of rennet-induced milk gels from raw milk and milk treated by high hydrostatic pressure (483 or 676 MPA) or heat (63°C, 30 min, BHT or 72°C, 15 s, CHT)
Figure 6 Loss tangent as function of Torque for rennet-induced gels. (c) Milks were pressurized at 483 MPa (▼) or 676 MPa (Δ). (d) Milks heated at BHT (Δ) or CHT (▼).
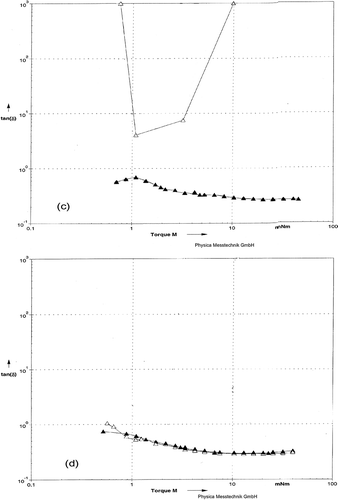
Differences in light scattering may provide indirect evidence of altered gel structure. shows the absorbance or turbidity of milk samples held at 25°C after HHP and HT treatments. Turbidity increased in the order 676 MPa < 483 MPa < BHT < CHT < Raw. Raw milk had significantly higher turbidity than milks treated with either 676 or 483 MPa. Milk samples treated with 676 MPa showed lower (p < 0.05) turbidity than all samples except milk treated with 483 MPa.
DISCUSSION
The main factor responsible for the formation of coagulant-induced milk gels is the casein fraction of the milk proteins, for they constitute the gel matrix.[Citation27] Protein gels are distinguished by their viscoelastic or rubber-like characteristics.[Citation41] Our results show that the level of pressure influenced the rheological characteristics of coagulant-induced milk gels. Pressurizing milk at 483 MPa resulted in higher G* value during gelation (), more elastic behavior (), lower tan δ value (), more gel strength (), stronger gel firmness at cutting (), and reduced milk turbidity () compared to milk treated at 676 MPa. The molecular mechanisms by which higher pressures affect the rheological properties of milk gels are complex and poorly understood. Pressurizing milk at high levels causes partial loosening within casein particles in the gel network due to solublization of calcium and phosphate.[Citation43] The removal of these minerals from the colloidal phase may alter the balance between viscous and elastic components in the gel network, resulting in soft gel.[Citation44] Whey proteins are denatured at high pressures and aggregate to casein micelles or sub-micelles.[Citation45] The interaction of denatured whey proteins with casein micelles reduces the sensitivity of the κ-casein fraction towards chymosin, leading to weak gels.[Citation46] Applying higher pressure (676 Mpa) caused an increase in milk turbidity due to the disruption of casein micelles[Citation15] and the denaturation of whey proteins, particularly β-lactoglobulin.[Citation47]
In general, our results show that there were no major differences in the gels obtained from milk treated with 483 MPa HHP or HT with respect to gel formation and strength during the coagulation process, gel strength, and the gel firmness at cutting. However, the structures of the gel network and the protein-protein interactions were different. HHP and HT of milk may be distinguished by the different sensitivities of the milk proteins to denaturation or aggregation under both treatments.[Citation11] Heat treatment of milk for cheese making, BHT or CHT, causes partial denaturation of whey proteins without affecting the casein micelles.[Citation25]
The results in this study show that the slow heating (BHT) resulted in a softer gel than rapid heating (CHT, ). Apparently, molecular interactions were initiated during heating, which were affected by heating rate. Hermansson[Citation48] and Foegeding et al.[Citation49] have shown that heating rate has a marked influence on properties of protein gels, presumably by altering the relative rates of conformational change of protein-protein aggregation. This study demonstrated how HHP treatments affect the coagulation process and gel firmness at cutting of coagulant-induced milk gels as compared to the HT milk gels.
ACKNOWLEDGMENTS
The authors wish to thank Jordan University of Science and Technology; M. San Martin, J. Rodriguez, and F. Younce for their technical assistance; and Dr. J. Tang for use of the Texture Analyzer.
REFERENCES
- Che , L. , Li , D. , Wang , L. , Özkan , N. , Dong , C.X. and Mao , Z. 2007 . Effect of high-pressure homogenization on the structure of cassava starch . International Journal of Food Properties , 4 : 911 – 922 .
- Atılgan , M.R. and Unluturk , S. 2008 . Rheological properties of liquid egg products (LEPS) . International Journal of Food Properties , 2 : 296 – 309 .
- Mertens , B. and Knorr , D. 1992 . Development of non-thermal process for food preservation . Food Technology , 46 : 124 – 133 .
- Metrick , C. , Hoover , D.G. and Farkas , D.F. 1989 . Effect of high hydrostatic pressure on heat-resistance and heat-sensitive strains of Salmonella . Journal of Food Science , 54 : 1547 – 1549 .
- Patterson , M.F. , Quinn , M. , Simpson , R. and Gilmour , R. 1995 . Sensitivity of vegetative pathogens to high hydrostatic pressure in phosphate buffered saline and foods . Journal of Food Protection , 58 : 524 – 529 .
- Datta , N. and Deeth , H.C. 1999 . High pressure processing of milk and dairy products . Australian Journal of Dairy Technology , 54 : 41 – 48 .
- Cheftel , J.C. and Dumay , E. 1996 . “ Effects of high pressure on dairy proteins: A review ” . In High Pressure Bioscience and Biotechnology , Edited by: Hayashi , R. and Balny , C. 299 – 308 . New York , , USA : Elsevier Science B.V. .
- Drake , M.A. , Harrison , S.L. , Asplund , M. , Barbosa-Canovas , G.V. and Swanson , B.G. 1997 . High-pressure treatment of milk and effects on microbiological and sensory quality of cheddar cheese . Journal of Food Science , 62 : 843 – 846 . 860
- Velez-Ruiz , J.F. , Swanson , B.G. and Barbosa-Canovas , G.V. 1998 . Flow and viscoelastic properties of concentrated milk treated by high hydrostatic pressure . LWT—Food Science and Technology , 31 : 182 – 195 .
- López-Fandino , R. 2006 . High pressure-induced changes in milk proteins and possible applications in dairy technology . International Dairy Journal , 16 : 1119 – 1131 .
- Felipe , X. , Capellas , M. and Law , J.R. 1997 . Comparison of the effect of high pressure treatments and heat pasteurization on the whey proteins in goat's milk . Journal of Agricultural and Food Chemistry , 45 : 627 – 631 .
- Needs , E.C. , Stenning , R.A. , Gill , A.L. , Ferragut , V. and Rich , G.T. 2000 . High-pressure treatment of milk: Effects on casein micelle structure and on enzymatic coagulation . Journal of Dairy Research , 67 : 31 – 42 .
- Anema , S.G. 2008 . Effect of milk solids concentration on whey protein denaturation, particle size changes and solubilization of casein in high-pressure-treated skim milk . International Dairy Journal , 18 : 228 – 235 .
- Ohmiya , K. , Kajino , T. , Shimizu , S. and Gekko , K. 1989 . Dissociation and reassociation of enzyme treated caseins under higher pressure . Journal of Dairy Research , 56 : 435 – 442 .
- Johnston , D.E. , Austin , B.A. and Murphy , R.J. 1992 . Effect of high hydrostatic pressure on milk . Milchwissenschaft , 47 : 760 – 763 .
- Johnston , D.E. , Austin , B.A. and Murphy , R.J. 1993 . Properties of acid set gel prepared from high pressure treated skim milk . Mlichwissenschaft , 48 : 206 – 209 .
- Desobry- Banon , S. , Richard , F. and Hardy , J. 1994 . Study of acid and rennet coagulation of high-pressurized milk . Journal of Dairy Science , 77 : 3267 – 3274 .
- López-Fandino , R. , Carrascova , A.V. and Olano , A. 1996 . The effect of high pressure on whey protein denaturation and cheese-making properties of raw milk . Journal of Dairy Science , 79 : 929 – 936 .
- Molina , E. , Alvarez , M.D. , Ramos , M. , Olano , A. and Lopez-Fandino , R. 2000 . Use of high-pressure treated milk for the production of reduced-fat cheese . International Dairy Journal , 10 : 467 – 475 .
- Parris , N. , Purcell , J.M. and Ptashkin , S.M. 1991 . Thermal denaturation of whey proteins in skim milk . Journal of Agricultural and Food Chemistry , 39 : 2167 – 2170 .
- Gay , M.F. , Jaubert , G. and Saboureau , S. 1993 . Effect of pasteurization and microfiltration on the hygienic quality of goats milk and on soft goats cheese . Lait , 73 : 499 – 509 .
- Dalgleish , D.G. 1990 . Denaturation and aggregation of serum proteins and caseins in heated milk . Journal of Agricultural and Food Chemistry , 38 : 1995 – 1999 .
- Singh , H. and Latham , J.M. 1993 . Heat stability of milk: Aggregation and dissociation of proteins at ultra-high temperatures . International Dairy Journal , 3 : 225 – 237 .
- Fox , P.F. 1982 . “ Heat-induced coagulation of milk ” . In Developments in Dairy Chemistry , Edited by: Fox , P.F. 189 – 228 . London : Applied Science Publishers .
- Law , A.J.R. , Horne , D.S. , Banks , J.M. and Leaver , J. 1994 . Heat-induced changes in the whey proteins and caseins . Mlichwissenschaft , 49 : 125 – 129 .
- Gunasekaran , S. and Ay , C. 1994 . Evaluating milk coagulation with ultrasonic . Food Technology , 12 : 74 – 78 .
- Nolan , E.J. , Holsinger , V.H. and Shieh , J.J. 1989 . Dynamic rheological properties of natural and imitation mozzarella cheese . Journal of Texture Studies , 20 : 179 – 189 .
- Burgess , K.J. 1978 . Measurement of the firmness of milk coagulum . Irish Journal of Food Science and Technology , 2 : 129 – 134 .
- Renner-Nantz , J.J. and Shoemaker , C.F. 1999 . Rheological properties of chymosin-induced skim milk gels as affected by milk storage time and temperature . Journal of Food Science , 64 : 86 – 89 .
- Beaulieu , M. , Pouliot , Y. and Pouliot , M. 1999 . Thermal aggregation of whey proteins in model solutions as affected by casein/whey protein ratios . Journal of Food Science , 64 : 776 – 780 .
- Beaulieu , M. , Pouliot , Y. and Pouliot , M. 1999 . Composition and microstructure of casein: Whey proteins aggregates formed by heating model solutions at 95C . International Dairy Journal , 9 : 393 – 394 .
- Shaker , R.R. , Jumah , R.Y. and Abu-Jdayil , B. 2000 . Rheological properties of plain yogurt during coagulation process: Impact of fat content and preheat treatment . Journal of Food Engineering , 44 : 175 – 180 .
- Renault , C. , Gastald , E. , Cud , J.L. and Tarodo de Lafuente , B. 2000 . Effect of temperature of milk acidification on rennet gel properties . Journal of Food Science , 65 : 630 – 634 .
- Van Hooydonk , A.C.M. , Hagedoorn , H.G. and Boerrigter , I.J. 1986 . pH induced physcio-chemical changes of casein micelles in milk and their effect on renneting. Effect of acidification on physico-chemical properties . Netherlands Milk and Dairy Journal , 40 : 281 – 296 .
- Buchheim , W. , Schrader , K. , Morr , C.V. , Ferde , E. and Schutt , M. 1996 . Effect of high pressure on the protein, lipid and mineral phase of milk . International Dairy Federation Bulletin , 2 : 202 – 213 .
- Minitab, Macintosh Inc . 1991 . Minitab User's Guide (Release 8): Statistical Analysis Software , State College , PA : Minitab Inc .
- Kim , B.Y. and Kinsella , J.E. 1989 . Rheological changes during slow acid induced gelation of milk by D-glucono-lactone . Journal of Food Science , 54 : 894 – 898 .
- Ferry , J.D. 1980 . Viscoelastic Properties of Polymers , 3rd , New York : John Wiley & Sons, Inc .
- Stading , M. , Langton , M. and Hermansson , A. 1995 . Small and large deformation studies of protein gels . Journal of Rheology , 39 : 1445 – 1450 .
- Shoemaker , C.F. , Renner-Nantz , J. , Bonnans , S. and Noble , A.C. 1992 . Rheological characterization of dairy products . Food Technology , 1 : 98 – 103 .
- Clark , A.H. and Ross-Murphy , S.B. 1987 . Structural and mechanical properties of biopolymer gels . Advances in Polymer Science , 83 : 57 – 192 .
- Aleandri , R. , Buttazzoni , L.G. , Caroli , A. and Daroli , R. 1989 . The effect of milk protein polymorphisms on milk components and cheese producing ability . Journal of Dairy Science , 73 : 241 – 255 .
- Gaucheron , F. , Famelart , M.H. , Mariette , F. , Raulot , K. , Michel , F. and Legraet , Y. 1997 . Combined effects of temperature and high-pressure treatments on the physicochemical characteristics of skim milk . Food Chemistry , 59 : 439 – 447 .
- Zoon , P. , Van Vliet , T. and Walstra , P. 1988 . Rheological properties of rennet-induced skim milk gels. 2. The effect of calcium and phosphate . Netherlands Milk and Dairy Journal , 42 : 295 – 312 .
- Brooker , B. , Farragut , V. , Gill , A. and Stenning , R. 1998 . “ Properties of rennet gels formed from high-pressure treated milk ” . In Fresh Novel Foods by High Pressure (VTT Symposium) , 55 – 60 . Espoo , , Finland : Technical Research Center of Finland .
- Van Hooydonk , A.C.M. , De Koster , P.G. and Boerrigter , I.J. 1987 . The renneting properties of heated milk . Netherlands Milk and Dairy Journal , 41 : 3 – 9 .
- Hinrichs , J. and Kessler , H.G. 1997 . “ Kinetics of pressure induced denaturation of whey proteins at different temperatures and functional properties ” . In High Pressure Research in the Bioscience and Biotechnology , Edited by: Heremans , K. 407 – 410 . Leuven , Belgium : Leuven University Press .
- Hermansson , A.M. 1978 . Physico-chemical aspects of soy protein structure formation . Journal of Texture Studies , 9 : 33 – 37 .
- Foegeding , E.A. , Allen , C.E. and Dayton , W.R. 1986 . Effect of heating rate on thermally formed myosin, fibrinogen and albumin gels . Journal of Food Science , 51 : 104 – 108 .