Abstract
The aim of this work was to determine low-molecular volatile compounds in milk supplemented with the strain Bifidobacterium animalis subsp. lactis Bb-12 with or without fermentation process, stored at 6°C for 4 weeks. The chromatographic analysis of probiotic-supplemented non-fermented milk and milk fermented by strain Bb-12 revealed the presence of volatile compounds, such as ketones, organic acid, and alcohols. The changes in the volatile profile were influenced by fermentation process and/or prolonged cold storage. The fermentative activity of strain Bb-12 and its intensification between the 2nd and 4th weeks were observed in the cold storage condition of milk.
INTRODUCTION
Among the variety of applications of gas chromatography, it could be used to analyze fermented food ingredients produced by probiotic bacteria, especially aroma profile.Citation[1] Probiotic bacteria are defined as live microorganisms that, when administered in adequate amounts, confer a health benefit on the host. They are incorporated as live cultures into foods, mainly fermented dairy products.Citation[2] Besides health-promoting properties, probiotic strains should guarantee the adequate quality and the preferred flavours in the products in which they are used. In the case of traditional fermented milk products, a selection of starter culture is based on precise and desirable fermentative properties of lactic acid bacteria applied in the dairy industry. The ability of lactose fermentation was the first criterion, whereas probiotics are normally found in the gastrointestinal tract of humans and animals. The origin of probiotics explains their weak ability to lactose fermentation (not all strains are able to ferment lactose) and ability to produce many substances untypical to lactic acid fermentation.Citation3–5
Several species of Bifidobacterium are considered to be the most important among the probiotic organisms. They were first isolated from the faeces of breast-fed-infants.Citation[6] Due to metabolic capacities of bifidobacteria, they are often included in the lactic acid bacteria family, even though they are phylogenetically distinct and belong to the family of Actinomycetaceae.Citation[7] Cow's milk is not a good environment for their growth.Citation[3, Citation5] One of the bifidogenic factors, which enhance the growth and the viability of bifidobacteria, is methyl-N-acetyl D-glucosamine in human milk.Citation[8,Citation9] Although bifidobacteria strains ferment lactose, they are unable to generate enough lactic acid for the manufacture of fermented foods with characteristic aroma and flavour, especially when used as a monoculture.Citation[3]
Compared with yoghurt bacteria, much less is known about the flavour compounds produced by probiotic bacteria. Volatile analysis of yoghurt has shown that acetaldehyde is a key compound for typical yoghurt aroma. In addition, 2,3-butanedione (diacetyl), ethanol, 2-butanone, and acetone are also important.Citation10–12 Ott et al.Citation[13] demonstrated the importance of acidity and the balance (ratios) of more than 60 flavour compounds in the perception of yoghurt aroma. The monocultures of probiotics are responsible for low consumer acceptance of fermented products, compared to products fermented by traditional lactic acid bacteria cultures.Citation[4,Citation5] Therefore, bifidobacteria are generally propagated in pure cultures and then mixed with lactic acid bacteria.Citation[3] The mixed cultures form volatile aromatic compounds more actively than the pure cultures.Citation[14] Using multi-species cultures has a favourable effect, not only on sensory features of products, but also on growth of the applied microflora.Citation[15] Probiotic strains of Bifidobacterium sp. or Lactobacillus acidophilus are mixed with yoghurt cultures of Streptococcus thermophilus and Lactobacillus delbrueckii subsp. Bulgaricus.Citation[16] Typical yoghurt bacteria guarantee the formation of suitable flavour of fermented products, because they are selected on this basis, while a probiotic strains addition was selected on the basis on their safety and health-promoting properties. They increase nutritive value of the fermented products, while acceptable flavour is one of the essential components of dairy shelf-life, in addition to the nutritional value, texture, and appearance of the product.
Østlie et al.Citation[17] demonstrated that the probiotic strains (B. animalis Bb-12, Lb. rhamnosus GG, Lb. acidophilus 1748, and Lb. reuteri SD2112) produced different amounts of metabolic products (volatile compound included) according to temperature and fermentation time. The amounts of volatile compound produced varied greatly both among the organisms tested and according to different incubation temperatures. Acetic acid levels were highest in milk inoculated with B. animalis Bb-12. The highest amounts of acetaldehyde were produced by Lb. acidophilus La5. Lb. reuteri SD2112 produced considerably higher amounts of ethanol than the other strains at all temperatures. Maximum acetoin levels were observed in milk incubated with Lb. reuteri SD2112 after 2, 4, and 12 h corresponding to an incubation temperature of 45, 37, and 30°C. B. animalis Bb-12 produced the highest amount of acetoin after 48 h incubation at 30°C.
The suitable proportion of substances, such as acetaldehyde, acetic acid, butanoic acid, and ketones, as well as some carbonyl compounds (acetoin, diacetyl) is responsible for desirable flavour of fermented milk.Citation[16,Citation18–22 Our previous studies on the mixed cultures of yoghurt bacteria, Lb. acidophilus and Lb. casei, demonstrated the intensive production of several volatile compounds, present at the end of 4 weeks of cold storage in the fermented and probiotic-supplemented non-fermented milk.Citation[23] The aim of this study was to determine low-molecular volatile compounds responsible for flavour changes in fermented and non-fermented milk supplemented with the monoculture of Bifidobacterium animalis subsp. lactis probiotic strain Bb-12, under prolonged cold storage conditions.
MATERIALS AND METHODS
Preparation of Probiotic Culture and Milk Samples
A probiotic culture of Bifidobacterium animalis subsp. lactis strain Bb-12 (Chr. Hansen, Hørsholm, Denmark) was used for the study. A 2-g sample of lyophilized culture was dissolved into 50 ml of sterile milk. Then, samples of ultra high temperature pasteurized (UHT) milk were inoculated with prepared culture, in a quantity of 1 ml per 150 ml. The first sample was control UHT milk, 3.2% fat content, without live bacteria. The second milk sample was inoculated with the B. animalis subsp. lactis strain Bb-12, fermented at 37°C for 18 h and chilled down to 6°C (a model of fermented milk). The third milk sample was placed in a fridge at 6°C immediately after inoculation with B. animalis subsp. lactis strain Bb-12 (a model of probiotic-supplemented non-fermented milk). The samples of control UHT milk, fermented milk, and probiotic-supplemented non-fermented milk were stored at 6°C for the whole time of the experiment (4 weeks). The pH value and volatile organic compounds were analyzed on the 0, 2nd, and 4th weeks. In this study, we studied the fermented and non-fermented milk samples containing probiotic strain B. animalis subsp. lactis Bb-12, by monitoring volatile compounds responsible for undesirable flavour changes in the dairy product during the cold storage.
Head Space-Solid Phase Micro Extraction (HS-SPME)
The SPME fiber was inserted into a 100-ml clear glass bottle tight fitted containing 5 g of sample and internal standard. 1-Propanol was used as an internal standard (1 μg of 1-propanol mixed with 3 g of sodium chloride per sample). Samples were first equilibrated at 30°C for 25 min and then a divinylbenzene/carboxene/polydimethylsiloxane-type fibre (DVB/CAR/PDMS; Supelco, Bellefonte, PA, USA) with phases thickness of 30/50 μm was exposed to the samples for 25 min at the same temperature. Our previous experiences (data not published) proved that this equilibration programme (time and temperature) as well as choice of the fibre can be applied to fermented milk products' volatile compounds analysis. This fibre has been used for several foods.Citation24–27 Before the analysis, the fibre was preconditioned in the injection port of the GC as indicated by the manufacturer. After equilibration, the fibre was removed from the sample and the analytes were thermally desorbed in the injector port of the gas chromatograph. Thermal desorption was performed in the injector glass liner at 220°C for 2 min.
Gas Chromatography–Mass Spectrometry (GC–MS) Conditions
Volatile compounds were desorbed in the injector port of a gas chromatograph coupled with a mass spectrometer (Shimadzu GC-MS QP20105, Shim-Pol, Warsaw, Poland). The injector temperature was 220°C, and an injection was equipped with a narrow liner. Separation was completed on a 30 m × 0.25 mm i.d. × 0.25 μm film thickness polar capillary column with a stationary phase ZB WAX (Phenomenex GC Columns; Zebron, Shim-Pol, Warsaw, Poland) with helium carrier gas linear flow rate of 0.69 ml/s. The oven temperature was programmed from 40 to 220°C at a rate of 4°C/min with initial hold time of 2 min, and final hold time of 5 min. Desorption of compounds was realized for 2 min. Separation was completed on detector settings: ion source temperature: 190°C; GC-MS interface line temperature: 200°C; electron ionization energy: 70 eV; detector voltage: 0.9 kV; quadruple filter scanning range: 40–300 m/z. The chromatographic analysis was continued for 45 min. Our previous experiences (data not published) proved that this temperature programme can be applied to fermented milk products' volatile compounds analysis. The compounds were identified by comparison with mass spectra from a library database (WILEY7N2, NIST147, and PAL600K) and retention index. For the calculation of the retention indexes, n-alkanes C8–C20 (Sigma-Aldrich, Poznań, Poland) were used (). The percentage composition of the volatile compounds was calculated in peak areas. The results are expressed as means of three replicates for each experimental point.
Table 1 The calculated retention indexes of volatile compounds
pH Value
pH value measurement was performed using a LPH33OT-type pH-meter (Tocussel Electronique, Solea, France) providing readings up to the second decimal number and temperature compensation.
Statistical Analysis
Statgraphics Plus software, version 5.1 (StatPoint Technologies, Inc., Herndon, VA, USA) was used to perform the basic statistical analysis of the obtained data. Analysis of variance (Tuckeys HSD Intervals, Multifactor ANOVA) was applied to the data to determine the presence of significant differences between milk samples and during refrigerating storage. The model was statistically significant with a p-value <0.05. Principal component analysis (PCA) was performed using the multivariate method on the Statistica software (version 9; StatSoft, Inc., Tulsa, OK, USA) to obtain a visual overview of correlations between the volatile compounds, fermentation, and cold storage time.
RESULTS AND DISCUSSION
Bifidobacterium animalis subsp. lactis are classified as heterofermentative bacteria. Their heterofermentative metabolic pathway differs from heterofermentative pathway of typical lactic acid bacteria. It comprises fructose-6-phosphate phosphoketolase in bifidobacteria, and aldolase and glucose-6-phosphatase dehydrogenase in lactobacilli.Citation[28] Bifidobacteria ferment glucose to lactic acid and acetic acid in the proportion of 3:2.Citation[5,Citation28] These compounds and other volatile organic compounds formed during fermentation could be responsible for undesired flavour of different fermented milk products. It is known that acetic acid has the utmost unfavourable influence on fermented milk flavour, because it is responsible for the unpleasant acetic smell and undesirable bitter taste.Citation[20] The comparison of volatile profiles of three samples: control milk, probiotic-supplemented non-fermented milk, and milk fermented by B. animalis subsp. lactis Bb-12 could indicate the bacteria metabolites responsible to form undesirable changes in milk products during fermentation and/or long-time cold storage.
A total of 14 volatile compounds were identified in all milk samples: organic acids, ketones, alcohols, etc. The volatile fatty acids (acetic acid, butanoic, capronic, caprylic, propanoic, and isovaleric acids) detected in all milk samples (Figs. ) may originate from lipolysis, degradation of lactose and amino acids, and they can also be derived from ketones, esters, and aldehydes by oxidation or microbial fermentation.Citation[14,Citation29–31 Acetic acid dominated in the volatile profile of milk fermented by bifidobacteria (), although probiotic-supplemented non-fermented milk contained significantly more quantity of acetic acid than control milk, immediately after the beginning of the experiments and during cold storage (p-value <0.05). The differences in the volatile profiles of probiotic-supplemented non-fermented milk () and milk fermented by B. animalis subsp. lactisBb-12 () proved the significance of the fermentation process, and indicated definitely the formation of volatile compounds at low temperature. The biggest difference among milk samples was observed in acetic acid content, especially between control UHT milk () and milk fermented by B. animalis subsp. lactis Bb-12 (). In spite of weak ability of bifidobacteria to ferment lactose, acetic acid content in fermented milk confirmed their heterofermentative nature.
Figure 1 Profile of volatile compounds in probiotic-supplemented non-fermented milk (containing B. animalis subsp. lactis strain Bb-12) during 4 weeks of storage at 6°C (average values and standard deviation).
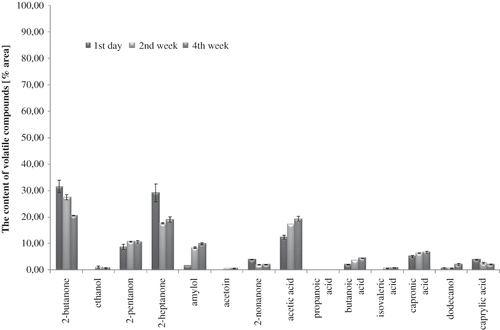
Figure 2 Profile of volatile compounds in milk fermented by B. animalis subsp. lactis strain Bb-12 during 4 weeks of storage at 6°C (average values and standard deviation).
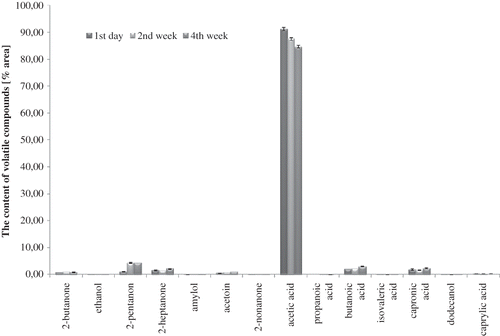
Figure 3 Profile of volatile compounds in control UHT milk during 4 weeks of storage at 6°C (average values and standard deviation).
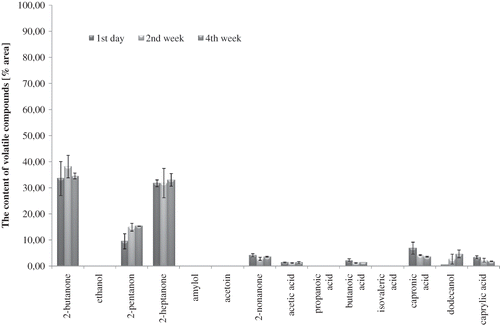
After 2 weeks of cold storage, a significant increase of butanoic, isovaleric, and capronic acids contribution in volatile profile was observed in probiotic-supplemented fermented milk and fermented milk samples (p < 0.05). These changes, as well as the decrease of acetic acid contribution to the volatile profile of fermented milk observed after the 2nd week of cold storage, indicated that the aroma is not stable even at low temperature. At the same time, the contribution of butanoic and capronic acids decreased to the volatile profile of control UHT milk. These changes did not influence the pH value, which was stable in almost all milk samples during 4 weeks of cold storage (). Neutral pH of control milk was unchanged during cold storage. The increase in butanoic acid and capronic acid contribution to the volatile profile in the last 2 weeks of the experiments could influence the undesirable changes in flavours of probiotic-supplemented non-fermented milk and fermented milk samples in the direction of perceptible rancid flavour.Citation[32] Beshkova et al.[14] confirmed the influence of fermentation process and cold storage on butanoic acid level and capronic acid level, as well as acetic acid level.
Figure 4 pH value in control UHT milk, probiotic-supplemented non-fermented milk (NF milk), and milk fermented by B. animalis subsp. lactis strain Bb-12 (F milk) during 4 weeks of storage at 6°C (average values and Tuckey's HSD Intervals, Multifactor ANOVA, α = 0.05).
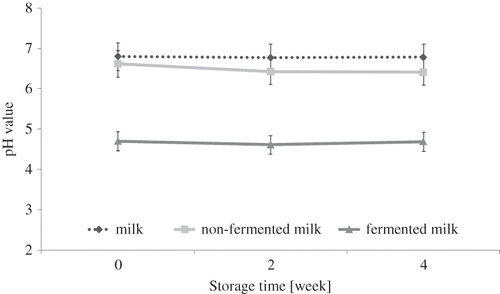
It is known that ketones (2-pentanone, 2-butanone, 2-heptanone, 2-nonanone, and acetoin) are important to total flavour quality of fermented milk products,Citation[14,Citation33] but they are also present in small quantities in pure cow's milk (). The adequate concentration of these substances creates the natural, pleasant flavour of milk. In the first day of analysis, the participation of ketones in the volatile profile was higher in control UHT milk () and probiotic-supplemented fermented milk () than in fermented milk (). The content of 2-butanone changed during cold storage, but its contribution in volatile profile of control milk () and probiotic-supplemented non-fermented milk () was comparable, whereas in fermented milk (), it was 20 to 30 times lower during whole cold storage (p-value <0.05). During the cold storage, the contribution of the other ketones in the volatile profile significantly changed (p < 0.05) in all milk samples. These changes observed in control milk after the 2nd week of cold storage could be the result of biochemical changes catalyzed by native milk enzymes, as well as auto-oxidation processes.Citation[31,Citation34]
The results of the present work confirmed that ketones are products of fatty acid, sugar, and amino acid breakdown, and not only as an effect of microbial activity. 2-Butanone is a compound occurring in significant quantity in yoghurts as well as kefir, besides other substances, such as diacetyl and ethanol.[16,33] This could explain why the products supplemented with monocultures of Bifidobacterium sp. have low consumer acceptance. Aghlara et al.[33] detected 40 volatile compounds in fermented milk with kefir starter culture (including ethanol, ethyl acetate, ethyl butyrate, 2-butanone, acetone, acetoin, diacetyl, and acetaldehyde). The concentration of 2-butanone was stable during fermentation of kefir, and other volatile compounds increased throughout the fermentation process. Beshkova et al.[14] showed the presence of a significant amount of acetic acid, butanoic acid, and capronic acid in yoghurt, but in the present work some of these compounds (2-pentanone, 2-butanone, 2-heptanone, butanoic acid, capronic acid, dodecanol, and caprylic acid) were also detected in the control milk, but not in a considerable quantity.
The other volatile compounds identified in milk samples were alcohols: dodecanol, ethanol, and amylol. The volatile alcohols are typical products of biochemical activity of lactic acid bacteria.Citation[21,Citation33] The alcohols may contribute to flavour because of their ability to form esters with fatty acids, and they might be expected to result from reduction of corresponding aldehydes.Citation[35] Ethanol and amylol were observed only in milk samples supplemented with B. animalis subsp. lactis strain Bb-12 ( and ). The duration of cold storage significantly influenced the content level of these compounds (p-value <0.05 for both). Ethanol was detected in fermented milk immediately after fermentation (), and its presence in probiotic-supplemented non-fermented milk () was observed just in the fourth week of cold storage. It proved that bacteria cells had some fermentative activity even during storage at 6°C. Ethanol was a result of bacterial alcohol dehydrogenase activity, which catalysed the reduction of acetaldehyde to ethanol. Low concentration of ethanol was the result of an overproduction of acetic acid, which is a compound competing in bacterial metabolic pathway with acetaldehyde formation.Citation[36,Citation37] Østlie et al.[17] demonstrated that the amount of acetaldehyde produced by probiotic strains (B. animalis Bb-12, Lb. rhamnosus GG, Lb. acidophilus 1748, and Lb. reuteri SD 2112) varied greatly both among the organisms tested and according to different incubation temperatures.
Observed amylol contribution in the volatile profile of probiotic-supplemented non-fermented milk proved the activities of alcohol dehydrogenase, glutamate dehydrogenase, and keto acid dehydrogenase in bifidobacteria. Interestingly, the contribution of amylol in volatile profile of milk fermented by B. animalis subsp. lactis Bb-12 was significantly lower than that of probiotic-supplemented non-fermented milk (p-value <0.05). This could be a result of low pH value of environment reducing activity of the enzymes mentioned above (pH value of fermented milk decreased to 4.70 and probiotic-supplemented non-fermented milk decreased to 6.43, after 4 weeks of cold storage). The highest contribution of amylol in the volatile profile was observed in probiotic-supplemented non-fermented milk () in the fourth week of cold storage (p-value <0.05). The contribution of amylol in volatile profile of probiotic-supplemented non-fermented milk was 10 times higher than in milk fermented by B. animalis subsp. lactis Bb-12 (). Among identified volatile alcohols, the presence of dodecanol was observed (lauryl alcohol). Dodecanol was detected in all milk samples during the whole cold storage period. It is a lipid alcohol and natural compound of milk. It is produced as a result of the auto-oxidation of fatty acids, and in excessive amounts it could influence the appearance of off-flavours.Citation[32,Citation36,Citation37] Initially, the concentration of dodecanol was comparable in all samples (Figs. ).
In order to determine the differences among milk samples (), PCA, using the relative percentage values for all detected volatile compounds, was performed. All milk samples segregated over 99.5% of total variance, accounting for the first, second, and third principal components, respectively. All samples of control UHT milk (regardless of the storage time) were segregated from samples of milk fermented by B. animalis subsp. lactis Bb-12. All samples of 2-week-old and 4-week-old probiotic-supplemented non-fermented milk were also segregated from milk fermented by B. animalis subsp. lactis Bb-12. This analysis indicated that control UHT milk and (regardless of the storage time) probiotic-supplemented non-fermented milk immediately after inoculation had identical volatile profile.
Figure 5 A three-dimensional plot of control UHT milk (M), probiotic-supplemented non-fermented milk (NF), and milk fermented by B. animalis subsp. lactis strain Bb-12 (F) during 4 weeks (1: 1st day; 2: 2nd week; and 4: 4th week) of storage at 6°C.
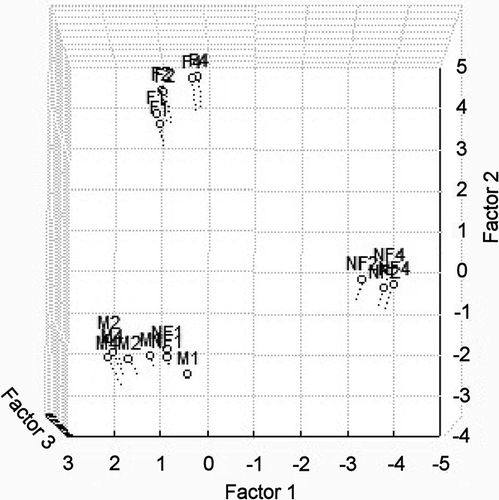
Østlie et al.Citation[38] demonstrated the importance of controlling the fermentation time since the probiotic strains (Lactobacillus acidophilus La5, Lb. acidophilus 1748, Lactobacillus rhamnosus GG, Lactobacillus reuteri SD 2112, and B. animalis subsp. lactis Bb-12) produced different amounts of metabolic products according to fermentation time. All probiotic strains showed very different profiles of metabolites (lactic acid, acetic acid, diacetyl, acetoin, and acetaldehyde included) during fermentation. Østlie et al.[17] and Gallardo-Escamilla et al.[20] proved the importance of controlling milk fermentation parameters. Gallardo-Escamilla et al.[20] found that the volatile compounds contributed most to prediction of yoghurt odour were acetaldehyde and 2,3 pentanedione. The volatile compounds important for prediction of “fruity” odour were propan-1-ol, ethanol, acetone, acetic acid, 2-butanone, diacetyl, and acetoin. Our work confirmed the presence of some of these volatile substances in probiotic-supplemented non-fermented milk and milk fermented by B. animalis subsp. lactis Bb-12. In addition, the concentration level of amylol and 2-butanone in probiotic-supplemented non-fermented milk should be taken into consideration, whereas the flavour of milk fermented by B. animalis subsp. lactis Bb-12 was strongly influenced by acetic acid, butanoic acid, capronic acid, and 2-pentanone. Motyl and LibudiszCitation[39] demonstrated that the shelf-life of milk fermented by bifidobacteria should be shortened to ca. 17 days, because after this time the undesirable changes in flavour occur. In the present work, the fermentative activity of B. animalis subsp. lactis Bb-12 and its intensification between the 2nd and 4th weeks of storage at 6°C were observed despite the cold condition of storage of samples. This could be important for the shelf-life definition of probiotic-supplemented milk products.Citation[40]
CONCLUSION
The changes in the volatile profile are influenced by the fermentation process, as well as by the cold storage period. The differences in the volatile profiles of probiotic-supplemented non-fermented milk and milk fermented by B. animalis subsp. lactis Bb-12 indicated the formation of volatile compounds not only during the fermentation process, but also during storage at low temperature.
ACKNOWLEDGMENT
This work was supported by a grant from Warsaw University of Life Sciences—WULS-SGGW.
REFERENCES
- Álvarez-Martín , P. , Belén Flórez , A. , Hernández-Barranco , A. and Mayo , B. 2008 . Interaction between dairy yeasts and lactic acid bacteria strains during milk fermentation . Food Control , 19 ( 1 ) : 62 – 70 .
- Saarela , M. , Mogensen , G. , Fonden , R. , Matto , J. and Mattila , S.T. 2000 . Probiotic bacteria: Safety, functional and technological properties . Journal of Biotechnology , 84 : 197 – 215 .
- Samona , A. , Robinson , R.K. and Marakis , S. 1996 . Acid production by bifidobacteria and yoghurt bacteria during fermentation and storage of milk . Food Microbiology , 13 : 275 – 280 .
- Adhikari , K. , Mustapha , A. , Grun , I.U. and Fernando , L. 2000 . Viability of microencapsulated bifidobacteria in set yogurt during refrigerated storage . Journal of Dairy Science , 83 : 1946 – 1951 .
- Doleyres , Y. and Lacroix , C. 2005 . Technologies with free and immobilised cells for probiotic bifidobacteria production and protection . International Dairy Journal , 15 : 973 – 988 .
- Ishibashi , N. , Yaeshima , T. and Hayasawa , H. 1997 . Bifidobacteria: Their significance in human intestinal health . Malaysian Journal of Nutrition , 3 : 149 – 159 .
- Klein , G. , Pack , A. , Bonaparte , C. and Reuter , G. 1998 . Taxonomy and physiology of probiotic lactic acid bacteria . International Journal of Food Microbiology , 41 : 103 – 125 .
- Petschow , B.W. and Talbott , R.D. 1990 . Growth promotion of bifidobacterium species by whey and casein fractions from human and bovine milk . Journal of Clinical Microbiology , 28 : 287 – 292 .
- Mountzouris , K.C. , McCartney , A.L. and Gibson , G.R. 2002 . Intestinal microflora of human infants and current trends for its nutritional modulation . British Journal of Nutrition , 87 : 405 – 420 .
- Ulberth , F. and Kneifel , W. 1992 . Aroma profiles and sensory properties of yogurt and yogurt-related products. II . Classification of starter cultures by means of cluster analysis. Milchwissenschaft , 47 : 432 – 434 .
- Marshall , V.M. 1993 . Starter cultures for milk fermentation and their characteristics . Journal of Society Dairy Technology , 46 : 49 – 56 .
- Skriver , A. , Stenby , E.;. , Folkenberg , D.M. , Runge , M. and Bang Jensen , N. 2003 . Tools in the development of future starter cultures for fermented milk . IDF Seminar on Aroma and Texture of Fermented Milk, Kolding, Denmark, June, 2002 International Dairy Federation, Brussels, Belgium , : 55 – 66 .
- Ott , A. , Hugi , A. , Baumgartner , M. and Chaintreau , A. 2000 . Sensory investigation of yogurt flavour perception: Mutual influence of volatiles and acidity . Journal of Agricultural Food Chemistry , 48 : 441 – 450 .
- Beshkova , D. , Simova , E. , Frengova , G. and Simov , Z. 1998 . Production of flavour compounds by yogurt starter cultures . Journal of Industrial Microbiology & Biotechnology , 20 : 180 – 186 .
- Rajiv , I. and Shah , P. 1997 . Viability of yoghurt and probiotic bacteria in yoghurts made from commercial starter cultures . International Dairy Journal , 7 : 31 – 41 .
- Gardini , F. , Lanciotti , R. , Guerzoni , M.E. and Torriani , S. 1999 . Evolution of aroma production and survival of Str. salivarius subsp. thermophilus, Lb. delbrueckii subsp. bulgaricus and Lb. acidophilus in fermented milks . International Dairy Journal , 9 : 125 – 134 .
- Østlie , H.M. , Treimo , J. and Narvhus , J.A. 2005 . Effect of temperature on growth and metabolism of probiotic bacteria in milk . International Dairy Journal , 15 ( 10 ) : 989 – 997 .
- Tamime , A.Y. and Robinson , R.K. 1999 . Yoghurt. Science and Technology , 551 – 559 . Cambridge : Woodhead Publishing Ltd .
- Leroy , F. and De Vuyst , L. 2004 . Lactic acid bacteria as functional starter cultures for the food fermentation industry . Trends in Food Science and Technology , 15 : 67 – 78 .
- Gallardo-Escamilla , F.J. , Kelly , A.L. and Delahunty , C.M. 2005 . Influence of starter culture on flavor and headspace volatile profiles of fermented whey and whey produced from fermented milk . Journal of Dairy Science , 88 : 3745 – 3753 .
- Smit , G. , Smit , B.A. and Engels , W.J.M. 2005 . Flavour formation by lactic acid bacteria and biochemical flavours profiling of cheese products . FEMS Microbiology Review , 29 : 591 – 610 .
- Macciola , V. , Candela , G. and De Leonardis , A. 2008 . Rapid gas-chromatographic method for the determination of diacetyl in milk, fermented milk and butter . Food Control , 19 ( 9 ) : 873 – 878 .
- Zareba , D. , Obiedzinski , M. and Ziarno , M. 2008 . The comparison of volatile compounds profile of milk fermented and non-fermented by yoghurt bacteria and probiotic strains . Żywność , 57 : 18 – 32 . in Polish
- Marsili , R.T. 1999 . Comparison of solid-phase microextraction and dynamic headspace methods for the gas chromatographic–mass spectrometric analysis of light-induced lipid oxidation products in milk . Journal of Chromatographic Science , 37 : 17 – 23 .
- Mills , G.A. and Walker , V. 2000 . Headspace solid-phase microextraction procedures for gas chromatographic analysis of biological fluids and materials . Journal of Chromatography A , 902 : 267 – 287 .
- Roberts , D.D. , Pollien , P. and Milo , C. 2000 . Solid-phase microextraction method development for headspace analysis of volatile flavour compounds . Journal of Agricultural and Food Chemistry , 48 : 2430 – 2437 .
- Wyllie , S.G. and Fellman , J.K. 2000 . Formation of volatile branched chain esters in bananas . Journal of Agricultural and Food Chemistry , 48 : 3493 – 3496 .
- Dinakar , P. and Mistry , P.P. 1994 . Growth and viability of bifidobacterium bifidum in cheddar cheese . Journal of Dairy Science , 77 : 2854 – 2864 .
- Curioni , P.M.G. and Bosset , J.O. 2002 . Key odorants in various cheese types as determined by gas chromatography-olfactometry . International Dairy Journal , 12 : 959 – 984 .
- Helinck , S. , Le Bars , D. , Moreau , D. and Yvom , M. 2004 . Ability of thermophilic lactic acid bacteria to produce aroma compounds from amino acids . Applied Environmental Microbiology , 70 : 3855 – 3861 .
- Baranowska , M. 2004 . The content of volatile free fatty acids in milk cultured with yoghurt bacteria . Polish Journal of Food and Nutrition Sciences , 17 : 13 – 21 .
- Marsili , R. 2002 . “ Flavours and off-flavours in dairy foods ” . In Encyclopedia of Dairy Science , Edited by: Roginski , H. 1069 – 1073 . London : Academic Press .
- Aghlara , A. , Mustafa , S. , Manap , Y.A. and Mohamad , R. 2009 . Characterization of headspace volatile flavor compounds formed during kefir production: Application of solid phase microextraction . International Journal of Food Properties , 12 ( 4 ) : 808 – 818 .
- Ardo , Y. 2006 . Flavour formation by amino acid catabolism . Biotechnology Advances , 24 : 238 – 242 .
- Labropoulos , A.E. , Palmer , J.K. and Tao , P. 1982 . Flavor evaluation and characterization of yogurt as affected by ultra-high temperature and vat processes . Journal of Dairy Science , 65 : 191 – 196 .
- Fernandez Murga , M. , De Ruiz Holgado , A.P. and De Valdez , G.F. 1998 . Survival rate and enzyme activities of lactobacillus acidophilus following frozen storage . Cryobiology , 36 : 315 – 319 .
- Robinson , R.K. Yoghurt . 2003 . “ role of starter cultures ” . In Encyclopedia of Dairy Science , Edited by: Roginski , H. 1059 – 1062 . London : Academic Press .
- Østlie , H.M. , Helland , M.H. and Narvhus , J.A. 2003 . Growth and metabolism of selected strains of probiotic bacteria in milk . International Journal of Food Microbiology , 87 : 17 – 27 .
- Motyl , I. and Libudisz , Z. 2001 . Zmiany Wybranych Cech Jakościowych Podczas Przechowywania Nieukwaszonego i Ukwaszonego Mleka Bifidusowego . Żywność , 28 : 107 – 117 . in Polish
- Condurso , C. , Verzera , A. , Romeo , V. , Ziino , M. and Conte , F. 2008 . Solid-phase microextraction and gas chromatography mass spectrometry analysis of dairy product volatiles for the determination of shelf-life . International Dairy Journal , 18 : 819 – 825 .