Abstract
The molecular structure and physicochemical properties of acid-alcohol-treated (0.36% HCl in methanol at 25°C up to 216 h) chickpea starch were investigated. The recovery for modified chickpea starch ranged from 82 to 91%. A significant decrease in the swelling power, pasting viscosity, and gel strength was observed upon treatment with acid-methanol; however, the granule morphology remained unchanged. Structural studies revealed preferential degradation of amylose and long-chain amylopectin during treatment and the same was attributed to cause alterations in the functional properties. Molecular studies further revealed that the lower content ratio of short-chains to long-chains of amylopectin in chickpea starch resulted in the faster degradation rate upon acid-methanol treatment. An exponential model was also developed from the average molecular weight data to illustrate the degradation kinetics of acid-methanol-treated chickpea starch.
INTRODUCTION
Acid hydrolysis of starch in an aqueous solution of dilute HCl or H2SO4 at certain temperatures for various times to produce “soluble starch” has been in practice for many years. The product thus obtained, called thin-boiling starch, is used extensively in the food, textile, and paper industries.[Citation1] However, recently acid hydrolysis in the presence of alcohol is becoming more popular[Citation2–4 as it can yield higher recovery of modified starch by using less amounts of acid. Cleavage of glycosidic bonds between monosaccharides in polysaccharides is the observed mechanism for acid hydrolysis,[Citation5] while the mechanism of acid-alcohol treatment involves hydrolysis of glycosidic bonds with the water inside the granules.[Citation6] High-performance size-exclusion chromatography (HPSEC) has been reported to be helpful in elucidating the effect of acid-alcohol treatment on molecular structure of starches by observing their molecular weight and chain length distributions.[Citation2–4 Among various alcohols (methanol, ethanol, 2-propanol, and 1-butanol) used to produce modified potato and waxy maize starches, Ma and Robyt[Citation7] experienced the highest average degree of polymerization (DP) with methanol.
Grain legumes constitute an important part in human and animal nutrition due to their desirable nutritional properties. In general, these are rich in starch, protein, and dietary fiber along with significant amounts of vitamins and minerals and high energetic value.[Citation8–10 Thus, historically these have been used mainly as whole seeds. But recently, characterization[Citation11–14 and utilization[Citation15–17 of legumes has drawn attention of researchers to investigate opportunities of value-addition activities. Furthermore, legumes are being explored for extraction of starch and protein—their main fractions.[Citation18–21 Since the utilization of both of the main components, starch and protein, simultaneously is highly important for making such processes profitable, therefore, investigations[Citation4,Citation22,Citation23] are undertaken primarily to provide possible alternative applications for legume starches.
Among various legumes, chickpea (Cicer arietinum L.) is considered of high economic importance as being the fifth in total worldwide production with 87.4% being contributed by Asia.[Citation24] These are classified into two types, desi and kabuli, depending upon their color and origin,[Citation25] with the former being three times higher in production.[Citation21] Their seeds are reported to contain 52.4 to 70.9% of total carbohydrates, the major proportion (37.5–50.8%) being of starch,[Citation8] which is considered to be competitive in the food industry.[Citation26,Citation27] Although there have been several reports on acid-alcohol modified starches obtained from cereals and tubers,[Citation2,Citation3,Citation7,Citation28–31 there is a dearth of such investigations for legume starches. Hence, with a theme of providing innovative speciality legume starch to the food industry, the molecular structure and physicochemical properties of acid-methanol-treated desi chickpea starch were explored in the present study.
MATERIALS AND METHODS
Materials
The desi chickpea sample was procured from a local market in Amritsar, Punjab, India. Isoamylase (EC 3.2.1.68) of Pseudomonas amyloderamosa (59,000 UI/mg) was purchased from Hayashibara Biochemical Laboratories, Inc. (Okayama, Japan). All other chemicals and solvents were of analytical grade.
Starch Isolation
The starch was isolated from chickpea using a method as described by Singh et al.[Citation19] The amylose content of the isolated starch determined by the method of Williams et al.[Citation32] was 28.1%.
Morphological Properties
Scanning electron micrographs (SEM) were obtained with a scanning microscope (Jeol JSM-6100, Jeol Ltd., Tokyo, Japan). Starch samples were suspended in ethanol to obtain 1% suspension. One drop of the starch-ethanol solution was applied on an aluminum stub, and the starch was coated with gold-palladium (60:40). An acceleration potential of 15 kV was used during observation.
Acid-Alcohol Modification
Starch was modified using the method of Lin et al.[Citation2] Starch (25 g) was suspended in 100 ml methanol (<0.3% water) in a 250-ml glass stopper flask. The suspension was stirred and maintained at 25°C. Reaction was started by adding 1 ml of concentrated (36% by weight) HCl, and allowed to proceed for 4, 8, 12, 24, 36, 48, 72, 120, 168, 216 h, respectively. The reaction was stopped by adding 14 ml of 1 M sodium bicarbonate, and then cooled in an ice bath. The starch was centrifuged at 3500× g for 5 min and was washed four times with 50% ethanol. The precipitate was air oven dried at 40°C. The yield was calculated by weight of the recovery starch to the initial weight of dry starch.
Swelling Power (g/g), Solubility (%), and Pasting Properties
Swelling power and solubility of starches were determined using method of Leach et al.[Citation33] Evaluation of pasting properties of acid-alcohol-modified starches was done using a Rapid Visco Analyzer (RVA-4, Newport Scientific Warriewood, Australia).[Citation34] Starch (3 g, 14% wb) was directly weighed in a RVA sample aluminum canister, and 25 ml of distilled water was added to it. It was then subjected to a programmed heating and cooling cycle where samples were held at 50°C for 1 min, heated to 95°C in 3.7 min, held at 95°C for 2.5 min before cooling to 50°C in 3.8 min and holding at 50°C for 2 min. The rotating speed of the paddle was initiated at 960 rpm for 10 s to allow thorough dispersion followed by a constant speed at 160 rpm for the remainder of the test. Parameters recorded were pasting temperature (Ptemp), peak viscosity (PV), hot paste viscosity (HPV) (minimum viscosity at 95°C), cold paste viscosity (CPV) (final viscosity at 50°C), breakdown (BD) (= PV-HPV), and setback (SB) (= CPV-HPV).
Gel Hardness
Hardness of the starch gels from a RVA test were evaluated using a TA/XT2 texture analyzer (Stable Microsystems, Surrey, England).[Citation35] The starch slurry formed in the canister after RVA testing was covered and kept at 4°C overnight and allowed to gel. The gel formed in the can (37 mm diameter, 20 mm height) was used directly for texture analysis. The gel was compressed to a distance of 10 mm using a flat cylindrical probe (5 mm diameter) at a cross head speed of 0.5 mm/s. The peak force obtained during the compression was recorded as gel hardness.[Citation36]
Molecular Weight Distribution
The molecular weight distributions of starches were determined by HPSEC.[Citation2] The solution of native starch was prepared by dissolving 75 mg (db) of starch with 15 ml, 90% dimethyl sulfoxide (DMSO) solution in a boiling water bath for 1 h with constant stirring, and then continuously stirred for 24 h at room temperature. Starch was precipitated from an aliquot of DMSO solution (2.1 ml) with excess absolute ethyl alcohol and centrifuged at 4000× g for 10 min. The precipitated amorphous starch pellet was re-dissolved in deionized water (15 ml, 95°C) and stirred with a magnetic stirrer in a boiling water bath for 30 min. To the acid–alcohol-modified starch, the starch solution was prepared by dissolving 10 mg (db) of starch with 15 ml deionized water and stirred with a magnetic stirrer in a boiling water bath for 1 h.
Each starch solution was filtered through a 5.0-μm syringe filter, and then the filtrate (100 μl) was injected into an HPSEC system. The system consisted of an HP G1310A isocratic pump (Hewlett Packard, Wilmington, DE, USA), a refractive index (RI) detector (HP 1047A Wilmington, DE, USA), and a multiangle laser light scattering (MALLS) detector (Dawn DSP, Wyatt Tech., Santa Barbara, CA, USA). The columns used were PWH (guard column), G5000PW and G4000PW (TSK-Gel, Tosoh, Japan) columns connected in series and kept at 70°C. The mobile phase was 100 mM phosphate buffer (pH 6.2) containing 0.02% NaN3 at a flow rate of 0.5 ml/min.
The electronic outputs of the RI and MALLS detectors were collected by ASTRA software (ver. 4.50, Wyatt Tech., Santa Barbara, CA, USA). Peaks were assigned using the RI chromatograms. The MALLS and RI signals were used to determine the molecule weight of amylopectin (first peak). Because of the reduced sensitivity of MALLS for small molecular weight species, the molecular weight of the second peak (amylose and degraded amylopectin fragments) of starches was calculated from the RI signal using a calibration curve constructed from a series of pullulan molecular weight standards ranging from 5.6 to 769.5 kDa (Polymer Standards Service, Silver Spring, MD, USA).
Chain Length Distribution
A starch solution (2.5 mg starch/2.45 ml H2O) was prepared according to the procedures described above. The solution was cooled, acetate buffer (0.05 ml, 1.0 M, pH 3.4) and isoamylase solution (10 μl, 5.9 U/μl) was added, and the mixture incubated in a shaker bath at 45°C for 24 h. The solution was neutralized with 0.1 M NaOH, and deionized with Amberlite IR-120-P and Amberlite IR-93 (Sigma, St. Louis, MO, USA) ion exchanger. The solution was diluted to 5 ml, and heated in a boiling water bath for 10 min. Debranched starch solutions were then filtered using a 0.45-μm syringe filter. The filtrate was injected (100 μl) into the HPSEC system. The system was the same as that used for the determination of molecular weight distribution, except the columns used were one G3000PWXL and two G2500PWXL (TSK-Gel, Tosoh, Japan) connected in series. A typical HPSEC profile of debranched starch showed trimodal distribution. The molecular weight of first peak (amylose) was determined by using MALLS and RI signals, and the molecular weight of the second and third peaks (long chain and short chain of amylopectin) were calculated from the RI signal using a calibration curve constructed from a series of Pullulan molecular weight standards ranging from 1.0 to 46.0 kDa (Polymer Standards Service, Silver Spring, MD, USA).[Citation2]
Statistical Analysis
Statistical comparison was conducted using the Duncan's multi-range test in a general linear model procedure of a SAS system (SAS Institute, Cary, NC, USA).
RESULTS AND DISCUSSION
Yield
The yields of the acid-alcohol-modified chickpea starch modified by 0.36% HCl in methanol at 25°C for 4–216 h were high, ranging from 82–91% (). The results are comparable to that obtained for acid-alcohol-modified lentil starches (91.9 to 95.6%) prepared under similar conditions.[Citation4] Lin et al.[Citation2] and Lin et al.[Citation37] reported similar yields (80–100%) for acid-alcohol-treated potato and maize starches. You and Izydorczyk[Citation29] also observed very little solubilization and reported very high recovery (91.5–99.8%) for barley starches with different amylose contents treated with acid-alcohol.
Table 1 Yield, swelling power, and solubility index of chickpea starch.Footnote a
Morphology
Scanning electron microscopy (SEM) was used to observe the morphology of native and modified chickpea starches. SEM of native chickpea starch showed the presence of large oval to small spherical shape granules (). Similar observations for chickpea starches have been reported.[Citation19,Citation38,Citation39] The granule surface of the native starch was smooth without fissures or cavities. With increasing hydrolysis time, no significant configuration changes, such as fragmentation or swelling, were found even after 216 h of modification ( and ), although some surface roughness became evident. However, in an earlier study on acid-alcohol-treated lentil starch,[Citation4] the formation of cavities, surface roughness and development of protuberances became clearly evident upon modification conducted under similar reaction conditions. Such behavior of chickpea starch has appeared similar to that of maize and potato starches as reported by Lin et al.[Citation2] They also reported no significant configuration changes in starch granules of maize and potato starches except for surface roughness after 15 days of modification under similar conditions. So the changes in functional properties of chickpea starch with acid-alcohol-treatment may be attributed and predominantly related to the changes in the molecular structure of the starch granule rather than the changes in granule morphology.
Swelling Power and Solubility Index
When starch is heated in excess of water, hydrogen bonds stabilizing the structure of the double helices in crystallites gets broken and replaced by hydrogen bonds with water,[Citation40] the starch granule swells, and the volume increases. A decrease in swelling power from 10.4 g/g of native chickpea starch to 4.6 g/g of chickpea starch after 216 h of acid-methanol treatment was observed (). A similar decreasing trend was reported on acid-methanol-treated lentil starch.[Citation4] Acid-alcohol-treated potato and maize starches had also shown similar results in their swelling powers.[Citation2] According to Sasaki and Matsuki,[Citation41] swelling volume of starch is affected by amylose content and the structure of amylopectin. Furthermore, the crystallites within the amylopectin molecule and the molecular weight and shape of the amylopectin have been reported to determine the onset of swelling and gelatinization.[Citation42] Thus, degradation of amylopectin molecules to lower molecular weight molecules due to acid-alcohol hydrolysis is inferred to be the reason for such decrease in swelling power.[Citation2,Citation4] The degradation of amylopectin upon acid-alcohol modification was confirmed in the present investigation by carrying out molecular weight distribution analysis.
The solubility of native chickpea starch was observed to be 13.2%, which is similar to previous reports.[Citation19,Citation38] The solubility of chickpea starch increased with the increase in hydrolysis time (). It showed a rapid increasing tendency after 24 h of treatment and the solubility of starch treated for 216 h was observed to be fairly high (46.5%). Solubility provides an evidence of the strength of interaction between starch chains, within the amorphous and crystalline domains. The amylose to amylopectin ratio and the characteristics of amylose and amylopectin in terms of molecular weight distribution, degree of branching, length of branches, and conformations of molecules mainly influence the extent of this interaction.[Citation43] Mukerjea et al.[Citation30] reported that the dissolved starches had generally much higher proportions of amylose to amylopectin ratios than their native granules. So the degradation of amylopectin during acid-alcohol treatment could be attributed to cause this increase in solubility of starches. Lin et al.[Citation2] also termed the increase in solubility to the disruption of granular structure caused by degradation of amylopectin, which consequently increased leaching when starch was heated in water.
Pasting Properties
summarizes the pasting properties of native and acid-alcohol-modified starches analyzed by using a RVA. The pasting temperature of native starch was observed to be 76.0°C. According to Singh et al.,[Citation19] the pasting temperatures for native chickpea starches were observed to be in the range of 75.1–77.1°C, while a pasting temperature of 71.1°C for lentil starch has been reported.[Citation4] The higher pasting temperature of chickpea starch than lentil starch indicates its more resistance to granule swelling and rupture. The observation of lower peak viscosity, breakdown, and higher hot paste viscosity of native chickpea starch than native lentil starch[Citation4] may also be attributed to similar reasoning.
Table 2 Pasting properties and gel hardness of chickpea starch.Footnote a
After acid-alcohol treatment, the pasting viscosity of the modified chickpea starches decreased significantly and showed a regular pattern of decrease with increasing hydrolysis time. This is in accordance with the decreasing swelling power and increasing solubility of acid-modified chickpea starches as reported earlier. Interestingly, although having more resistance to granule rupture, chickpea starch showed a faster decrease in pasting viscosities with an increase in hydrolysis time as compared to lentil starch treated under similar conditions[Citation4] indicating more susceptibility to degradation by acid-methanol treatment. The same could be attributed to the higher disruption of molecular structure inside granules[Citation2] for chickpea starch and was verified by HPSEC analysis conducted in the present investigation. A comparison of scanning electron microscopy observations also substantiated the above findings by revealing the formation of cavities, surface roughness, and development of protuberances for lentil starch, however, no significant configuration changes in chickpea starch granules after similar treatment were observed.
Gel Hardness
Hardness of RVA gels of native and modified chickpea starches evaluated using a TA/XT2 texture analyzer is presented in . The mechanical properties of starch gels depend upon various factors, including the rheological characteristics of the amylose matrix, the volume fraction, and rigidity of the gelatinized starch granules, as well as the interaction between the dispersed and continuous phases of gel.[Citation44] These factors are, in turn, dependent on amylose content and structure of amylopectin.[Citation45] Hardness of native starch gel was found to be 166 g. The hardness of starch gel decreased significantly upon acid-alcohol treatment and was observed to be 23 g at the highest level (216 h) of modification. Long chains in amylopectin play a key factor influencing the texture of starch gels, which has been reported by Lu et al.[Citation46] and that was further corroborated with the analysis presented by Sodhi et al.[Citation47] Hansen et al.[Citation48] also clearly established the importance of longer chains for the formation of a gel network. Hence, the decreased gel strength upon acid-methanol treatment could be attributed to degradation of amylose and long-chain amylopectin[Citation49] in acid-methanol-treated chickpea starches as evidenced by HPSEC results observed in the present study.
Molecular Weight Distribution
Molecular weight distribution profiles of native and acid-alcohol-treated chickpea starch, determined by HPSEC, showed bimodal distribution profiles () as those of lentil starches.[Citation4] The first fraction (F1) in the profiles corresponds to amylopectin, whereas F2 fraction corresponds to low molecular weight molecules. Acid-alcohol treatment caused a decrease in the areas of F1 fractions with an increase in treatment duration, while areas of F2 fractions increased. The weight percentage and weight average degree of polymerization (DPw) of starches are summarized in . These results indicate amylopectin degradation due to hydrolysis caused by acid-alcohol treatment to low molecular weight molecules. The same may be attributed to cause an increase in solubility and decrease in pasting viscosities as observed in the present study upon acid-alcohol treatment. Similar observations of amylopectin degradation upon acid-alcohol treatment have been reported earlier for maize, potato,[Citation2] and lentil starches.[Citation4]
Table 3 Weight percentage and weight average degree of polymerization (DP w ) of chickpea starch
Figure 2 HPSEC profiles of native and acid-alcohol-modified chickpea starch at different levels of modification. (Color figure available online.)
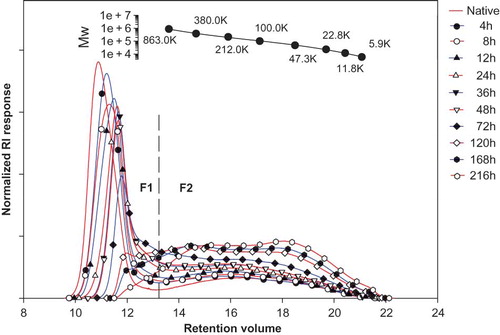
Lin and Chang[Citation31] reported a two-stage degradation pattern explainable by two different straight line equations during acid-methanol treatment of rice and corn starches; however, an exponential degradation pattern of the reciprocal of molecular weight versus duration of acid-methanol treatment for the chickpea starch has been observed in this study. The reduction in molecular weight with an increase in acid-alcohol treatment was observed to be explainable by the following equation:[Citation4]
where k or k' represents the rate constant, t, the reaction time, Mo , the DPw of native starch, Mt , the DPw of starch after being treated for time t, and m, the monomer molecular weight. The results indicated a slow degradation at early stages followed by a very rapid degradation rate (). A similar equation was used earlier to explain the degradation of lentil starch[Citation4] with acid-alcohol treatment. However, the degradation rate constant is observed to be higher for chickpea starch (k′ = 0.0189 h−1) as compared to lentil starch (k′ = 0.0178 h−1) indicating a faster degradation in the former. These differences in degradation patterns may be attributed to the differences in their botanical origins. The influence of botanical source on the degradation rate of starches during acid-alcohol treatment has been reported earlier.[Citation2,Citation6,Citation7,Citation37]
Chain Length Distribution
The HPSEC profiles of native and acid-methanol-treated chickpea starches after debranching with isoamylase were observed to have trimodal distributions () similar to those of lentil starches.[Citation4] summarizes the weight percentage (%) and weight-average chain length (CLw) of each fraction of native and acid-methanol-treated chickpea starches. The P1 fractions in the profiles correspond to amylose, while P2 and P3 fractions consist of long and short chains of amylopectin, respectively.[Citation50] The contents and CLw of P1 fractions showed a significant decrease upon acid-methanol treatment; however, an increase was observed for P2 fractions, which resulted from the degradation of P1 fraction.[Citation2] A slight increase was also observed for P3 fractions with acid-methanol treatment, which could be attributed to the reason that CLw of chains degraded from P2 fraction are similar to that of P3 fraction.[Citation3,Citation51] Hence, it may be inferred from the above discussion that amylose and long chains of amylopectin are preferentially degraded during acid-methanol treatment of chickpea starch. However, in comparison to that of lentil starch[Citation4] treated under similar conditions, a higher degradation rate of long chains of amylopectin was observed in chickpea starch. Long chains of amylopectin are longer branch chains that extend through two or more clusters.[Citation52] As acid hydrolysis preferentially occurs in amorphous regions[Citation2] and branch points passing through can be easily hydrolyzed,[Citation53] hence, it may be inferred that branch chains passing through the amorphous regions had more long chains of amylopectin. Furthermore, Lin and Chang[Citation31] reported a faster degradation rate for starches having a lower S/L ratio (content ratio of P3 to P2 fractions) in their study on acid-alcohol-treated cereal starches. Consequently, lower S/L ratio for chickpea starch (2.35) as compared to lentil starch,[Citation4] which has an S/L ratio of 4.14, may be attributed for faster degradation and, hence, more susceptibility to acid-alcohol-treatment.
Table 4 Weight percentage and chain length parameters of isoamylase-debranched chickpea starch
CONCLUSION
Chickpea starch exhibited recovery values of 82–91% after treatment up to 216 h in methanol containing 0.36% HCl at 25°C. Granule morphology was observed to remain unaltered without having any significant configuration changes with an increase in treatment time. However, their functional properties were significantly affected upon treatment with acid-methanol for various durations. The swelling power, pasting viscosity, and gel strength were observed to be significantly decreased with the acid-methanol treatment indicating that chickpea starch with desired functional properties suitable for a particular application can be achieved by controlling the treatment duration. This was attributed to the degradation of the molecular structure inside the starch granule as revealed by HPSEC studies. Preferential degradation of amylose and long-chain amylopectin was observed during acid-methanol treatment. While comparing the molecular structure studies with lentil starch[Citation4] treated under similar reaction conditions, a faster degradation rate for chickpea starch has been observed and is attributed to lower S/L ratio for chickpea starch. The comparison further led to the inference that long chains of amylopectin mainly consisted of the branch chains passing through the amorphous regions.
REFERENCES
- Wurzburg , O.B. 1986 . “ Converted starches ” . In Modified Starches: Properties and Uses , Edited by: Wurzburg , O.B. 17 – 40 . Boca Raton , FL : CRC Press .
- Lin , J.H. , Lee , S.Y. and Chang , Y.H. 2003 . Effect of acid-alcohol treatment on the molecular structure and physicochemical properties of maize and potato starches . Carbohydrate Polymers , 53 : 475 – 482 .
- Chang , Y.H. , Lin , J.H. and Chang , S.Y. 2006 . Physicochemical properties of waxy and normal corn starches treated in different anhydrous alcohols with hydrochloric acid . Food Hydrocolloids , 20 : 332 – 339 .
- Sodhi , N.S. , Chang , Y.H. , Kaur , N. and Kohyama , K. 2009 . Effect of acid-methanol treatment on the molecular structure and physicochemical properties of Lentil (Lens culinaris Medik) starch . Food Hydrocolloids , 23 : 2219 – 2225 .
- Yiu , P.H. , Loh , S.L. , Rajan , A. , Wong , S.C. and Bong , C.F.J. 2008 . Physicochemical properties of sago starch modified by acid treatment in alcohol . American Journal of Applied Sciences , 5 : 307 – 311 .
- Robyt , J.F. , Choe , J.Y. , Hahn , R.S. and Fuchs , E.B. 1996 . Acid modification of starch granules in alcohols: effects of temperature, acid concentration, and starch concentration . Carbohydrate Research , 281 : 203 – 218 .
- Ma , W.P. and Robyt , J.F. 1987 . Preparation and characterization of soluble starches having different molecular sizes and composition, by acid hydrolysis in different alcohols . Carbohydrate Research , 166 : 283 – 297 .
- Chavan , J.K. , Kadam , S.S. and Salunkhe , D.K. 1986 . Biochemistry and technology of chickpea (Cicer arietinum L.) seeds . CRC Critical Reviews in Food Science and Nutrition , 25 : 107 – 158 .
- Tharanathan , R.N. and Mahadevamma , S. 2003 . Grain legumes—A boon to human nutrition . Trends in Food Science and Technology , 14 : 507 – 518 .
- Almeida Costa , G.E.D. , Queiroz-Monici , K.D.S. , Machado Reis , S.M.P. and Oliveira , A.C.D. 2006 . Chemical composition, dietary fibre and resistant starch contents of raw and cooked pea, common bean, chickpea and lentil legumes . Food Chemistry , 94 : 327 – 330 .
- Kaur , S. , Singh , N. , Sodhi , N.S. and Rana , J.C. 2009 . Diversity in properties of seed and flour of kidney bean germplasm . Food Chemistry , 117 : 282 – 289 .
- Kaur , M. , Singh , N. and Sodhi , N.S. 2005 . Physicochemical, cooking, textural and roasting characteristics of chickpea (Cicer arietinum L.) cultivars . Journal of Food Engineering , 69 : 511 – 517 .
- Singh , N. , Kaur , M. , Sandhu , K.S. and Sodhi , N.S. 2004 . Physicochemical, cooking and textural characteristics of some Indian black gram (Phaseolus mungo L.) varieties . Journal of the Science of Food and Agriculture , 84 : 977 – 982 .
- Ereifej , K.I. , Al-Karaki , G.N. and Hammouri , M.K. 2001 . Seed chemical composition of improved chickpea cultivars grown under semiarid Mediterranean conditions . International Journal of Food Properties , 4 : 239 – 246 .
- Wood , J.A. 2009 . Texture, processing and organoleptic properties of chickpea-fortified spaghetti with insights to the underlying mechanisms of traditional durum pasta quality . Journal of Cereal Science , 49 : 128 – 133 .
- Gill , B.S. , Sodhi , N.S. and Kaur , M. 2005 . Effect of chickpea, ghee, sodium chloride, mixing time and resting time on instrumental texture and sensory quality of chapati . Journal of Food Science and Technology , 42 : 481 – 488 .
- Bhatty , N. , Gilani , A.H. and Nagra , S.A. 2000 . Effect of cooking and supplementation on nutritional value of gram (Cicer arietinum) . Nutrition Research , 20 : 297 – 307 .
- Hoover , R. and Ratnayake , W.S. 2002 . Starch characteristics of black bean, chick pea, lentil, navy bean and pinto bean cultivars grown in Canada . Food Chemistry , 78 : 489 – 498 .
- Singh , N. , Sandhu , K.S. and Kaur , M. 2004 . Characterization of starches separated from Indian chickpea (Cicer arietinum L.) cultivars . Journal of Food Engineering , 63 : 441 – 449 .
- Miao , M. , Zhang , T. and Jiang , B. 2009 . Characterisations of kabuli and desi chickpea starches cultivated in China . Food Chemistry , 113 : 1025 – 1032 .
- Sánchez-Vioque , R. , Clemente , A. , Vioque , J. , Bautista , J. and Millán , F. 1999 . Protein isolates from chickpea (Cicer arietinum L.): Chemical composition, functional properties and protein characterization . Food Chemistry , 64 : 237 – 243 .
- Kaur , M. and Singh , N. 2006 . Relationships between selected properties of seeds, flours, and starches from different chickpea cultivars . International Journal of Food Properties , 9 : 597 – 608 .
- Singh , V. and Ali , S.Z. 2008 . Properties of starches modified by different acids . International Journal of Food Properties , 11 : 495 – 507 .
- International Center for Agricultural Research in the Dry Areas. Completing genotyping of composite set of chickpea. http://www.icarda.org/GenerationCP/cp-1-chickpea.htm (http://www.icarda.org/GenerationCP/cp-1-chickpea.htm) (Accessed: 15 December 2009 ).
- Saini , H.S. and Knights , E.J. 1984 . Chemical constitution of starch and oligosaccharide components of “desi” and “kabuli” chickpea (Cicer arietinum) seed types . Journal of Agricultural and Food Chemistry , 32 : 940 – 944 .
- Botham , R.L. , Cairns , P. , Morris , V.J. , Ring , S.G. , Englyst , H.N. and Cummings , J.H. 1995 . A physicochemical characterization of chick pea starch resistant to digestion in the human small intestine . Carbohydrate Polymers , 26 : 85 – 90 .
- Goni , I. and Valentin-Gamazo , C. 2003 . Chickpea flour ingredient slows glycemic response to pasta in healthy volunteers . Food Chemistry , 81 : 511 – 515 .
- Lin , J.H. , Wang , S.W. and Chang , Y.H. 2008 . Impacts of acid-methanol treatment and annealing on the enzymatic resistance of corn starches . Food Hydrocolloids , 23 : 1465 – 1472 .
- You , S. and Izydorczyk , M.S. 2007 . Comparison of the physicochemical properties of barley starches after partial ∝-amylolysis and acid/alcohol hydrolysis . Carbohydrate Polymers , 69 : 489 – 502 .
- Mukerjea , R. , Slocum , G. and Robyt , J.F. 2007 . Determination of the maximum water solubility of eight native starches and solubility of their acidic-methanol and ethanol modified analogues . Carbohydrate Research , 342 : 103 – 110 .
- Lin , J.H. and Chang , Y.H. 2006 . Molecular degradation rate of rice and corn starches during acid-methanol treatment and its relation to the molecular structure of starch . Journal of Agricultural and Food Chemistry , 54 : 5880 – 5886 .
- Williams , P.C. , Kuzina , F.D. and Hlynka , I. 1970 . A rapid calorimetric procedure for estimating the amylose content of starches and flours . Cereal Chemistry , 47 : 411 – 420 .
- Leach , H.W. , McCowen , L.D. and Schoch , T.J. 1959 . Structure of the starch granules. I. Swelling and solubility patterns of various starches . Cereal Chemistry , 36 : 534 – 544 .
- Newport Scientific . 1998 . Applications Manual for the Rapid Visco™ Analyser , Warriewood , , Australia : Newport Scientific .
- Bhattacharya , M. , Zee , S.Y. and Corke , H. 1999 . Physicochemical properties related to quality of rice noodles . Cereal Chemistry , 76 : 861 – 867 .
- Bourne , M.C. 1978 . Texture profile analysis . Food Technology , 32 : 62 – 66 . 72
- Lin , J.H. , Lii , C.Y. and Chang , Y.H. 2005 . Change of granular and molecular structures of waxy maize and potato starches after treated in alcohols with or without hydrochloric acid . Carbohydrate Polymers , 59 : 507 – 515 .
- El Faki , H.A. , Desikachar , H.S.R. , Paramahans , S.V. and Tharanathan , R.N. 1983 . Physico-chemical characteristics of starches from chick pea, cow pea and horse gram . Starch , 35 : 118 – 122 .
- Lineback , D.R. and Ke , C.H. 1975 . Starches and low-molecular-weight carbohydrates from chick pea and horse bean flours . Cereal Chemistry , 52 : 334 – 347 .
- Tester , R.F. and Karkalas , J. 1996 . Swelling and gelatinization of oat starches . Cereal Chemistry , 73 : 271 – 277 .
- Sasaki , T. and Matsuki , J. 1998 . Effect of wheat starch structure on swelling power . Cereal Chemistry , 75 : 525 – 529 .
- Tester , R.F. and Morrison , W.R. 1990 . Swelling and gelatinization of cereal starches. I. Effects of amylopectin, amylose and lipids . Cereal Chemistry , 67 : 551 – 557 .
- Ratnayake , W.S. , Hoover , R. and Warkentin , T. 2002 . Pea starch: Composition, structure and properties—A review . Starch , 54 : 217 – 234 .
- Biliaderis , C.G. 1982 . Physical characteristics, enzymatic digestibility, and structure of chemically modified smooth pea and waxy maize starches . Journal of Agricultural and Food Chemistry , 30 : 925 – 930 .
- Yamin , F.F. , Lee , M. , Pollak , L.M. and White , P.J. 1999 . Thermal properties of starch in corn variants isolated after chemical mutagenesis of inbred line B73 . Cereal Chemistry , 76 : 175 – 181 .
- Lu , Z.H. , Sasaki , T. , Li , Y.Y. , Yoshihashi , T. , Li , L.T. and Kohyama , K. 2009 . Effect of amylose content and rice type on dynamic viscoelasticity of a composite rice starch gel . Food Hydrocolloids , 23 : 1712 – 1719 .
- Sodhi , N.S. , Sasaki , T. , Lu , Z.H. and Kohyama , K. 2010 . Phenomenological viscoelasticity of some rice starch gels . Food Hydrocolloids , 24 : 512 – 517 .
- Hansen , M.R. , Blennow , A. , Pedersen , S. , Norgaard , L. and Engelsen , S.B. 2008 . Gel texture and chain structure of amylomaltase-modified starches compared to gelatin . Food Hydrocolloids , 22 : 1551 – 1566 .
- Mua , J.P. and Jackson , D.S. 1997 . Relationships between functional attributes and molecular structures of amylose and amylopectin fractions from corn starch . Journal of Agriculture and Food Chemistry , 45 : 3848 – 3854 .
- Hizukuri , S. 1996 . “ Starch: Analytical aspects ” . In Carbohydrates in Food , Edited by: Eliasson , A.C. 347 – 429 . New York : Marcel Dekker .
- Chang , Y.H. , Lin , J.H. and Lii , C.Y. 2004 . Effect of ethanol concentration on the physicochemical properties of waxy corn starch treated by hydrochloric acid . Carbohydrate Polymers , 57 : 89 – 96 .
- Hizukuri , S. 1986 . Polymodal distribution of the chain lengths of amylopectins, and its significance . Carbohydrate Research , 147 : 342 – 347 .
- Gallant , D.J. , Bouchet , B. and Baldwin , P.M. 1997 . Microscopy of starch: Evidence of a new level of granule organization . Carbohydrate Polymers , 32 : 177 – 191 .