Abstract
Textural and rheological properties of raw, dried, and cooked noodles with microbial transglutaminase were evaluated by an instrumental texture analyser. Generally, the strength of the noodles significantly increased with microbial transglutaminase concentration from 0 to 1.0%. The cutting force of raw noodle is a good indicator to predict the strength of dried and cooked noodle products. Mechanical stress relaxation data of cooked noodles were fitted well by both Maxwell and Peleg-Normand models. The fitted parameters, λ1 and k2, were more sensitive to the changes in viscoelastic properties than k1 and %SR. Significant correlations were found between textural characteristics and stress relaxation parameters. Results of this study suggest that dried or cooked noodle with good quality can be produced by incubating with 0.5–1.0% microbial transglutaminase for 30 min at 30 °C.
INTRODUCTION
Noodle is a popular food in East Asian countries. Generally, wheat flour with 10–13% protein is used for preparing oriental noodle and the procedures for producing raw noodle are mixing, resting, compounding, sheeting, and cutting.[Citation1] Dried noodle is prepared from the raw noodle dehydrated by either natural drying or hot-air drying, and its advantages are long shelf-life, low cost, and easy to cook. The eating quality of cooked noodle depends largely on textural characteristics, such as hardness, elasticity, and surface smoothness.
Transglutaminase is an enzyme that catalyzes an acyl transfer reaction between the γ-carboxyamide of peptide–bound glutaminyl residue and a variety of primary amines. Industrially, microbial transglutaminase (MTGase) is mainly used to improve the texture, stability, water holding capacity, and other functional properties of various food products, such as wheat, soybean, seafood, and dairy products.[Citation2 − Citation4] Sakamoto et al.[Citation5] and Wu and Corke[Citation6] found that treatment of noodles with MTGase prevented the deterioration of texture upon cooking and increased the strength of the products. TGase improved the quality of cooked spaghetti[Citation7 − Citation9] and increased the hardness and tensile strength of cooked yellow noodle.[Citation10, Citation11] However, there is a lack of information regarding the effects of concentration and incubation time of MTGase on the quality of raw, dried, and cooked noodle products simultaneously.
Stress relaxation has traditionally been employed as one of the principal means for measuring the viscoelastic behavior of foods. Stress relaxation data are very important since they supply information about food products, such as fine structure of potato products,[Citation12] staling of corn products,[Citation13] and the quality of cheese[Citation14, Citation15] and puffed seeds.[Citation16] Generally, experimental force decay curve has been presented in the form of a discrete Maxwell model[Citation17] containing 2–4 elements. Peleg and Normand[Citation18] suggested that stress relaxation data can be recalculated to a normalized stress (or force) and next fitted with time. Peleg-Normand model has less constants than Maxwell model, thus the former is a simple, quick, and effective method to handle stress relaxation data. Singh et al.[Citation19] reported that k 2 value in the Peleg-Normand model was more suitable for differentiating various food products than k 1 value. Hatcher et al.[Citation20, Citation21] reported that damaged starch and alkali reagent affected the stress relaxation parameters in the Peleg-Normand model of yellow alkali noodles made from durum and common wheat varieties. Sozer et al.[Citation22] found that the stress relaxation data of cooked spaghetti were fitted well by both three-element Maxwell and Peleg-Normand models. As mentioned, the addition of TGase strengthened the structure of food products by covalent crosslinks between protein molecules. However, study on stress relaxation of noodle products incorporating with MTGase is rare so far.
The purpose of this study was to investigate the effects of the concentration and incubation time of MTGase on the quality of raw, dried, and cooked noodles, as well as to observe the correlations between empirical textural characteristics (cutting force, breaking strength, tensile strength, etc.) and fundamentally mechanical stress relaxation parameters of the noodles.
MATERIALS AND METHODS
Materials
Wheat flour with medium strength was purchased from Uni-President Co. (Tainan, Taiwan). The proximate composition of the flour was analyzed by the AACC methods.[Citation23] Commercial microbial transglutaminase ACTIVA® STG-M (MTGase) powder was obtained from Ajinomoto Taiwan Inc. (Taipei, Taiwan) and its activity was 25 Ug−1, according to the manufacturer's data.
Preparation of Doughs
MTGase (0–3.75 g) and salt (2.5 g) were completely dissolved in 90 mL of distilled water. The solution was mixed with wheat flour (250 g) in a mixer (Model TS-108, Tian Shuai Food Machine Co., Taichung, Taiwan) using slow and medium speeds, respectively, for 2 min to form dough with 36% moisture content (wet basis). The concentrations of MTGase used were 0–1.5% (flour basis). Then the dough was placed in a covered plastic box and rested for 5 min at ambient temperature (about 28°C) and hereafter is referred to as the dough.
Preparation of Raw, Dried, and Cooked Noodles
Dough sheets were produced by passing the dough between two rollers. First, the dough was sheeted and folded in half three times through a pair of rollers with a 10 mm gap at 20 rpm; then the resulted dough sheet was rested for 2 min in a plastic bag. The dough sheet was gradually reduced to a final thickness of 1.8 mm by consecutively passing through roll gaps of 5.2, 3.0, and 1.7 mm. For each gap, the dough sheet was rolled three times and rested for 2 min. Then the dough sheet was cut into three rectangular pieces (25 × 20 cm per piece). The rectangular dough sheets were sealed in plastic bags and incubated for different durations of time (30–90 min) in an oven at 30°C. Finally, raw noodles (25 cm long and 3 mm wide) were produced by cutting the incubated dough sheet. Parts of raw noodles were dried at 40°C and 50% RH for 17 h in an air oven, and the dried noodles were stored in sealable plastic bags before testing. About 20 g of dried noodles were cooked in boiling water (400 mL) for 18 min until the white core disappeared. The cooked noodles were collected by a 20-mesh screen and cooled for 8 min at ambient temperature. The cool cooked noodles were stored in sealable plastic bags, and their textural properties were measured as soon as possible.
Cutting Force and Tensile Strength of Raw and Cooked Noodles
The cutting forces of three strands of raw or cooked noodle were measured by using a Texture Analyser (TA-XT2i, Stable Micro Systems, Surrey, UK) fitted with a probe knife (A/LKB-F). The probe speed was 0.5 mm/sec and compression depth was 1.2 mm. Data were collected automatically by the Texture Expert software program. The hardness (N) and adhesiveness (N*s) of the noodles were recorded from the maximum force and from the area under X-axis on compression graph curve, respectively.[Citation24]
For tensile strength analysis, the Texture Analyser was equipped with a probe of Kieffer cell/dough extensibility rig and operated in tension mode. Both pretest and test speeds were set at 2.0 mm/sec to avoid vibrations that might occur at high speed. The tensile strength and extensibility of one strand of cooked noodle (8 cm long) were determined by recording the maximum force and the distance at the extension limit.[Citation25]
Breaking Force of Dried Noodle
The breaking force (N) of one strand of dried noodle (8 cm long) was measured using the Texture Analyser equipped with a three point bend rig (HDP/3PB). A strand of the dried noodle was horizontally placed onto the platform of the 3PB rig and test speed was set at 1.0 mm/sec. The breaking force of the dried noodle was recorded as the maximum force of compression curve.
Stress Relaxation of Cooked Noodle
The stress relaxation of cooked noodle was measured according to the method proposed by Sozer et al.[Citation22] with some modifications. The stress relaxation test was executed by using the Texture Analyser equipped with a P10 probe (10 mm diameter). Five strands of cooked noodle were placed beside each other without leaving any space among strands, and were cut into the required size for fitting the probe dimension. The samples were deformed in compression to a constant strain of 20% with a test speed of 0.5 mm/s. The data acquisition rate was 10 points per second. The residual force was continuously recorded as a function of time for 480 sec. The stress relaxation data were analyzed by using a Maxwell model as well as a Peleg-Normand model. Equation (Equation1) is a Maxwell model with three elements:
Cooking Properties
The cooking properties of noodle were determined using the method of Shiau.[Citation26] About 20 g of dried noodles (W 1), cut into 8 cm length, were cooked in boiling water (400 mL) for 18 min. The cooked noodles were collected by a 20-mesh screen and cooled for 8 min in a covered box at room temperature. The samples of the cooled noodles (W 2) were placed in a beaker (W 3), and dried at 105°C to a constant weight (W 4). Moisture content of cooked noodle was calculated as:
The beaker for cooking and screen were washed with distilled water. The combined filtrates were cooled to room temperature and diluted to 400 mL with distilled water. Then 10 mL of the cooled diluted solution were placed into a beaker (W 5) and dried at 105°C to a constant weight (W 6). The cooking loss of dried noodle was calculated as:
Statistical Analysis
The data in triplicate for different treatments were analyzed by one-way ANOVA and by Duncan's new multiple range test to determine the statistical significance of differences among the values, using the SAS System (SAS Institute, Cary, NC, USA). Pearson's simple correlation analysis was also conducted for observing the relations between textural characteristics and stress relaxation parameters.
RESULTS AND DISCUSSION
Effect of MTGase on Raw Noodle
The proximate composition of wheat flour used was 12.50% crude protein, 0.35% crude fat, and 0.40% ash contents (dry weight basis). shows the effects of MTGase concentration and incubation time on the cutting forces of raw noodles. Results showed that the cutting forces significantly increased with the enzyme concentration from 0 to 1.0% (0–25 U/g flour) for 30 min of incubation, and increased from 0 to 0.5% for both 60 and 90 min of incubation. The increase of cutting forces after adding MTGase can be attributed to the formations of intra- and intermolecular ϵ-(γ-glutamyl)-lysine crosslinks in gluten proteins caused by the MTGase reaction, and the higher MTGase concentration used the more crosslinks formed. However, the cutting forces of raw noodles incubated for 30 min with MTGase at 1.0 and 1.5% levels did not show a significant difference. This might be due to the limit content of lysine in gluten,[Citation27] which restricted the cross-linking reactions at high MTGase dose.
Figure 1 Effects of concentration and incubation time of MTGase on the cutting force of raw noodle. The incubation time was 30 (▲), 60 (о), and 90 min (X), respectively.
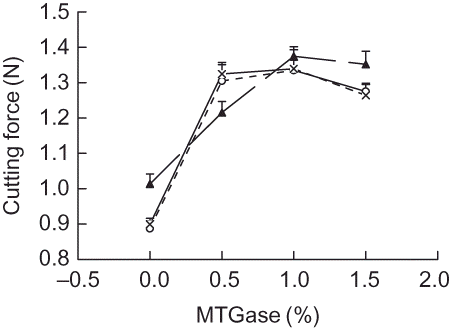
The cutting forces of raw noodles with 0.5% MTGase gradually increased with the incubation time of MTGase from 30 to 90 min. The cutting forces of raw noodles with 1.5% MTGase significantly decreased with increasing the incubation time from 30 to 60 min. This might be related to the secondary reaction of deamidation[Citation28] catalyzed by MTGase incubated for a long time at high concentrations. However, the cutting forces of control noodle decreased, and this effect could result from native protease reaction and/or SH-SS interchange reactions in protein molecules. Thus, it is important to select an appropriate MTG concentration and incubation time for preparing noodles.
Effect of MTGase on Dried Noodle
Since gluten protein is the main contribution to the strength of dried noodle, cross-linking of the protein by MTGase was expected to affect this parameter. shows the effects of MTGase concentration and incubation time on the breaking forces of dried noodles. The breaking force of dried noodle incubated for 30 min at 30°C significantly increased with the enzyme concentration (0–1.5%). This indicated that MTGase treatment was beneficial to avoid breaking the dried noodles during packaging and shipping. Aalami and Leelavathi[Citation8] reported that MTGase increased the breaking strength of dried spaghetti and improved the quality of spaghetti.
Figure 3 Effects of concentration (a) and incubation time (b) of MTGase on the cutting force (О), tensile strength (▲), extensibility (X), and adhesiveness (▪) of cooked noodle, respectively. The incubation time and concentration used for (a) and (b) were 30 min and 0.5%, respectively.
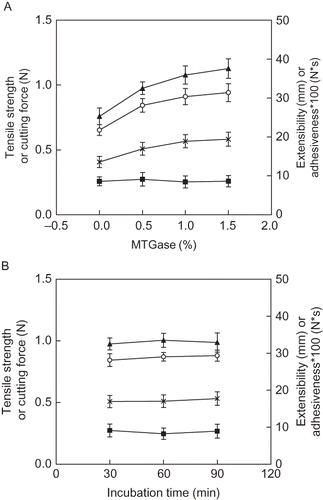
The breaking forces of dried noodles with 0.5% MTGase significantly increased with the incubation time of MTGase from 30 to 90 min (). However, breaking forces of dried noodles incubated with 1.0 and 1.5% MTGase did not show a significant difference after being treated for various incubation times. This indicates that the noodles with high dose of MTGase can be produced by short incubation time.
Effect of MTGase on Cooked Noodle
shows the effect of MTGase concentration on the empirical textural characteristics of cooked noodle incubated for 30 min at 30°C. Results showed that the cutting force, tensile strength, and extensibility of cooked noodles significantly increased with the enzyme concentration from 0 to 1.5%. However, these textural characteristics of the cooked noodles incubated with 0.5–1.5% MTGase were not significantly different. It is desirable that the stickiness and adhesiveness of cooked noodle are low. Although the adhesiveness of raw noodle was not detectable, cooked noodle had somewhat adhesive properties (84–91 mN.s) related to the gelatinization of starch. The adhesiveness of cooked noodle was not affected by MTGase concentration. The results are in line with the reports that MTGase increased the hardness of white salted noodle and instant noodle,[Citation6, Citation11] and the tensile strength of yellow noodle.[Citation10] The increase in hardness with MTGase treatment was related to a stronger and tighter protein network between the starch granules which was responsible for limiting excessive water uptake during cooking.[Citation29]
Figure 2 Effects of concentration and incubation time of MTGase on the breaking force of dried noodle. The incubation time was 30 (▲), 60 (О), and 90 min (X), respectively.
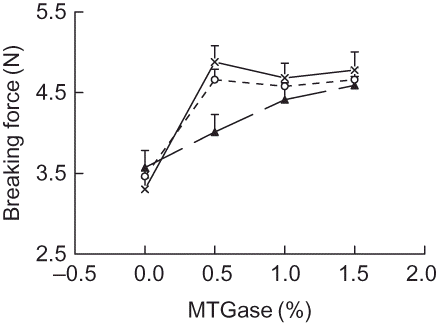
The cutting force, tensile strength, extensibility, and adhesiveness of cooked noodle were not affected by incubation time of MTGase either at 0.5% () or at higher dose levels (data not shown). Results in showed different trends from those in and . This is related to the cooking treatment, which caused the gelatinization of starch in cooked noodle. The quality and quantity of gluten protein are major factors affecting the strength of raw and dried noodles. However, the strength of cooked noodle is contributed by both denatured gluten protein and gelatinized starch. Thus, it is suitable to produce cooked noodle with good quality by incubating with 0.5–1.0% MTGase in spite of incubation time (from 30 to 90 min).
Fundamental viscoelastic properties of foods have frequently been measured by stress relaxation. The stress relaxation data of cooked noodles in this study were analyzed by the Maxwell model, Peleg-Normand model, and %SR. lists the fitted parameters of the three-element Maxwell model of cooked noodles with and without MTGase. Results showed that all stress relaxation data of cooked noodles were fitted well by the Maxwell model with three elements (R 2 > 0.99). For the two-element Maxwell model, R 2 values were approximately 0.96. Hence, the three-element Maxwell model fitted to the data better than the two-element Maxwell model did. A three-element Maxwell model was found to be adequate for demonstrating the stress relaxation data of cooked spaghetti.[Citation22]
Table 1 Fitted parameters of three-term Maxwell model using stress relaxation data of cooked noodles
The elastic component of the Maxwell element can be represented by the decay force (F 1, F 2, or F 3), which indirectly measures the rigidity of the material being tested. Relaxation time (λ1, λ2, or λ3) was defined as the time it took for a macromolecule to be stretched out when deformed.[Citation30] Both F 1 and λ1 parameters of cooked noodles with 0.5–1.5% MTGase were significantly higher than those without MTGase (). Higher decay force and longer relaxation time of cooked noodles with MTGase indicated that the noodles had a more rigid and elastic behavior. However, differences in F 3, λ2, and λ3 parameters were insignificant among the cooked noodles tested. Transglutaminase was found to increase the semirelaxation time of wheat dough.[Citation25]
Peleg-Normand model is a good alternative to the Maxwell model since it is easy to execute for analyzing the results. lists the fitted parameters of the Peleg-Normand model for cooked noodles with and without MTGase. Results showed that the stress relaxation data of cooked noodles were fitted well by the Peleg-Normand model (R 2 > 0.998). The k 2 parameter in the Peleg-Normand model indicates the extent of relaxation with values equal to one for a pure liquid and to infinite for a perfect elastic body. Results in indicated that the F 0 (initial force) parameters of cooked noodles without MTGase were the lowest among all treatments tested, while the k 2 value of noodle incubated with 1.0% MTGase showed significantly different from that of the control. Therefore, cooked noodle with 1.0% MTGase had higher elasticity than noodle without MTGase.
Table 2 Percent SR and fitted parameters of Peleg and Normand model using stress relaxation data of cooked noodles
The reciprocal of k 1 value represents the initial decay rate. A high k 1 value is associated with a low decay rate, indicating a pronounced elastic behavior. The addition of MTGase was not significantly affected the k 1 values of cooked noodles (). Singh et al.[Citation19] concluded that k 1 value was not a good indicator for differentiating various food products based on their viscoelastic behavior. But the additions of both resistant starch and wheat bran increased the k 1 values of optimally cooked spaghetti.[Citation22]
Percent stress relaxation (%SR) has been used to realize viscoelastic properties of food products,[Citation19] and is obtained directly from the plot of stress relaxation versus time plot at an arbitrary time. For the ideal elastic solid, %SR is equal to 0, while for the ideal liquid, %SR is 100. %SR of cooked noodle tested ranged from 31.8 to 33.8 (), thus the noodle was classified as a viscoelastic solid. Results in indicated that addition of MTGase did not significantly affect the %SR of cooked noodles. Among the parameters derived from stress relaxation data, %SR was not a good indicator for differentiating viscoelastic behavior of cooked noodles in this study. Both λ1 and F 1 in the Maxwell model were the best parameters to differentiate the viscoelastic behavior of the noodles. k 2 in the Peleg-Normand model was the next best parameter. However, both k 1 in the Peleg-Normand model and %SR were the least sensitive to the viscoelastic nature of the noodles.
Compared with the control sample, the magnitudes of change in cutting force, tensile strength, and extensibility of cooked noodles incubated with MTGase were up to 44, 48, and 43%, respectively (), and the magnitudes of change in F 1 and λ1 () as well as k 2 () were up to 23, 9, and 7%, respectively. Hence, the change in empirical textural characteristics in this study corresponded to the change in fundamental mechanical parameters of stress relaxation. Moreover, the magnitudes of change in empirical textural characteristics of cooked noodles were more than those in fundamental stress relaxation parameters.
lists the effect of MTGase concentration and incubation time on the cooking quality of noodles. Results showed that cooking loss (4.98%) of noodle without MTGase was significantly (p < 0.05) lower than cooking losses (5.84–6.12%) of noodles with the enzyme. However, there was not a significant difference in cooking loss among the noodles incubated with various MTGase concentrations and incubation times. This result implied that cross-linking of gluten protein by MTGase could decrease the protein-starch interactions, leading to an increase in the leaching of starch components into the cooking water. Cooking loss is the quantity of component released from the noodle during cooking. High value of cooking loss indicates cooked noodles with sticky surface and is not desirable. Cooking loss (4.98–6.12%) of noodle in this study was low and acceptable. The cooking loss of MTGase-treated spaghetti increased to 5.49–6.60% as compared to that of the control (5.35%).[Citation8] Wu and Corke[Citation6] concluded that MTGase did not significantly influence the cooking loss of white salted noodle. However, Takacs et al.[Citation9] reported that the addition of MTGase decreased cooking loss of pasta from 14.0 to 7.6%.
Table 3 Effects of MTGase concentration and incubation time on the cooking loss and moisture content of cooked noodle
Table 4 Correlation coefficients between textural and rheological characteristics of raw, dried, and cooked noodles
Moisture contents of cooked noodle with 0.5–1.5% MTGase were significantly lower than that of cooked noodle without MTGase (). Similar to the results of cooking loss, moisture contents of MTGase-treated noodles were not significantly different. Significant decreasing in moisture content or cooking yield could be associated with the tighter network structure resulted from MTGase reaction. Since the formation of ϵ-(γ-glutamyl)-lysine crosslink by MTGase could decrease the number of hydrophilic amino acids, fewer water molecules would be absorbed. This result is in agreement with the data that the yield and water uptake of cooked noodle and spaghetti were significantly decreased by MTGase.[Citation6, Citation8, Citation9]
Correlations Between Textural and Rheological Parameters
lists the correlation coefficients of textural and rheological parameters of raw, dried, and cooked noodles. The cutting force of raw noodle (CF1) was found to be positively correlated to the breaking force (BF) of dried noodle as well as the cutting force (CF2), tensile strength (TS), extensibility (E), and the fitted parameters (k 2 and λ1) of cooked noodle (p < 0.05). Therefore, the cutting force of raw noodle is a good indicator to predict the strength of dried and cooked noodles. BF of dried noodle was found to be positively correlated to CF2 and λ1 of cooked noodle. For cooked noodle, CF2 was found to be positively correlated to TS, E, F 0, k 2, and λ1, and TS was positively correlated to E, k 2, and λ1 (p < 0.05). This demonstrates that empirical textural characteristics (CF2, TS, and E) were consistent with fundamental mechanical properties (k 2 and λ1) of cooked noodle. Hatcher et al.[Citation20] reported that significant correlations were found among textural characteristics (maximum cutting stress, resistance to compression, and recovery) and stress relaxation parametrs (%SR, k 1, and k 2) of cooked yellow alkali noodles. Among the fitted parameters of stress relaxation, λ1 in the Maxwell model had positive correlation with k 2 and F 0 in the Peleg-Normand model (), while k 2 was negatively correlated to %SR.
CONCLUSION
Addition of MTGase significantly increased the strength of raw, dried, and cooked noodles and reduced the moisture content of cooked noodles. The cutting force of raw noodle is a good indicator to predict the strength of dried and cooked noodles. Generally, cutting force of raw noodle; breaking force of dried noodle; and cutting force, tensile strength, and extensibility of cooked noodle increased with the concentration of MTGase from 0 to 1.0%. The stress relaxation data of cooked noodles were fitted well by the Maxwell as well as the Peleg-Normand models. Compared empirical textural characteristics with fundamental stress relaxation parameters, the former was sensitive to the change by MTGase action. Significant correlations were found among empirical textural characteristics and fundamentally mechanical stress relaxation parameters. Results of this study suggest that dried or cooked noodle with good quality can be prepared by incubating with 0.5–1.0% MTGase for 30 min at 30°C.
ACKNOWLEDGMENTS
This study was financially supported by the National Science Council, Taiwan, ROC (NSC 94-2214-E-127-001). The authors are grateful to Ms. Wei-Jie Yang and Man-Yu Chang for assistance in the preparation and analysis of samples.
REFERENCES
- Oh , N.H. , Seib , P.A. , Deyoe , C.W. and Ward , A.B . 1983 . Noodles. 1. Measuring the textural characteristics of cooked noodles. Cereal Chemistry , 60 ( 6 ) : 433 – 438 .
- Kuraishi , C. , Yamazaki , K. and Susa , Y. 2001 . Transglutaminase: Its utilization in the food industry . Food Reviews International , 17 ( 2 ) : 221 – 246 .
- Dube , M. , Schaefer , C. , Neidhart , S. and Carle , R. 2007 . Texturization and modification of vegetable proteins for food applications using microbial transglutaminase . European Food Research and Technology , 225 ( 2 ) : 287 – 299 .
- Oner , Z. , Karahan , A.G. , Aydemir , S. and Sanlidere Aloglu , H. 2008 . Effect of transglutaminase on physicochemical properties of set-style yogurt . International Journal of Food Properties , 11 ( 1 ) : 196 – 205 .
- Sakamoto , H. , Yamazaki , K. , Kaga , C. , Yamamoto , Y. , Ito , R. and Kurosawa , Y. 1996 . Strength enhancement by addition of microbial transglutaminase during Chinese noodle processing . Nippon Shokuhin Kagaku Kaishi , 43 : 598 – 602 .
- Wu , J.P. and Corke , H. 2005 . Quality of dried white salted noodles affected by microbial transglutaminase . Journal of the Science of Food and Agriculture , 85 ( 15 ) : 2587 – 2594 .
- Basman , A. , Koksel , H. and Atli , A. 2006 . Effects of increasing levels of transglutaminase on cooking quality of bran supplemented spaghetti . European Food Research and Technology , 223 ( 4 ) : 547 – 551 .
- Aalami , M. and Leelavathi , K. 2008 . Effect of microbial transglutaminase on spaghetti quality . Journal of Food Science , 73 ( 5 ) : 306 – 312 .
- Takacs , K. , Gelencser , E. and Kovacs , E.T. 2008 . Effect of transglutaminase on the quality of wheat-based pasta . European Food Research and Technology , 226 ( 3 ) : 603 – 611 .
- Gan , C.Y. , Ong , W.H. , Wong , L.M. and Easa , A.M. 2009 . Effects of ribose, microbial transglutaminase and soy protein isolate on physical properties and in-vitro starch digestibility of yellow noodles . LWT–Food Science and Technology , 42 ( 1 ) : 174 – 179 .
- Choy , A.L. , Hughes , J.G. and Small , D.M. 2010 . The effects of microbial transglutaminase, sodium stearoyl lactylate and water on the quality of instant fried noodles . Food Chemistry , 122 ( 4 ) : 957 – 964 .
- Blahovec , J. 2003 . Activation volume from stress relaxation curves in raw and cooked potato . International Journal of Food Properties , 6 ( 2 ) : 183 – 193 .
- Limanond , B. , Castell-Perez , M.E. and Moreira , R.G. 2002 . Modeling the kinetics of corn tortilla staling using stress relaxation data . Journal of Food Engineering , 53 ( 3 ) : 237 – 247 .
- Venugopal , V. and Muthukumarappan , K. 2002 . Stress relaxation characteristics of Cheddar cheese . International Journal of Food Properties , 4 ( 3 ) : 469 – 484 .
- Joshi , N.S. , Jhala , R.P. , Muthukumarappan , K. , Acharya , M.R. and Mistry , V.V. 2004 . Textural and rheological properties of processed cheese . International Journal of Food Properties , 7 ( 3 ) : 519 – 530 .
- Markowski , M. , Ratajski , A. , Konopko , H. , Zapotoczny , P. and Majewska , K. 2006 . Rheological behavior of hot-air-puffed amaranth seeds . International Journal of Food Properties , 9 ( 2 ) : 195 – 203 .
- Mohsenin , N.N. 1970 . Physical Properties of Plant and Animal Materials , 88 – 173 . New York : 1st Ed.; Gordon and Breach Science Publishers .
- Peleg , M. and Normand , M.D. 1983 . Comparison of two methods for stress relaxation data presentation of solid foods . Rheologica Acta , 22 ( 1 ) : 108 – 113 .
- Singh , H. , Rockall , A. , Martin , C.R. , Chung , O.K. and Lookhart , G.L. 2006 . The analysis of stress relaxation data of some viscoelastic foods using a texture analyzer . Journal of Texture Studies , 37 ( 4 ) : 383 – 392 .
- Hatcher , D.W. , Bellido , G.G. , Dexter , J.E. , Anderson , M.J. and Fu , B.X. 2008 . Investigation of uniaxial stress relaxation parameters to characterize the texture of yellow alkaline noodles made from durum and common wheats . Journal of Texture Studies , 39 ( 6 ) : 695 – 708 .
- Hatcher , D.W. , Bellido , G.G. , Dexter , J.E. and Anderson , M.J. 2009 . Flour particle size, starch damage, and alkali reagent: Impact on uniaxial stress relaxation parameters of yellow alkaline noodles . Cereal Chemistry , 86 ( 3 ) : 361 – 368 .
- Sozer , N. , Kaya , A. and Dalgic , A.C. 2008 . The effect of resistant starch addition on viscoelastic properties of cooked spaghetti . Journal of Texture Studies , 39 ( 1 ) : 1 – 16 .
- AACC . 2000 . Approved Method of the AACC , 10th , St. Paul , MN : AACC International .
- Bourne , M.C. 1978 . Texture profile analysis . Food Technology , 32 ( 7 ) : 62 – 66 . 72
- Bollain , C. and Collar , C. 2004 . Dough viscoelastic response of hydrocolloid/enzyme/surfactant blends assessed by uni- and bi-axial extension measurements . Food Hydrocolloids , 18 ( 3 ) : 499 – 507 .
- Shiau , S.Y. 2004 . Effects of emulsifiers on dough rheological properties and the texture of extruded noodles . Journal of Texture Studies , 35 ( 1 ) : 93 – 110 .
- Rakowska , M. , Ochodzki , P. and Sikorski , Z.E. 2001 . “ Nutritive role of food proteins ” . In Chemical and Functional Properties of Food Proteins , 217 – 232 . Pennsylvania : CRC Press . InEd
- Ohtsuka , T. , Umezawa , Y. , Nio , N. and Kubota , K. 2001 . Comparison of deamidase activity of transglutaminase . Journal of Food Science , 66 ( 1 ) : 25 – 29 .
- Kovacs , M.I.P. , Fu , B.X. , Woods , S.M. and Khan , K. 2004 . Thermal stability of wheat gluten protein: Its effect on dough properties and noodle texture . Journal of Cereal Science , 39 ( 1 ) : 9 – 19 .
- Cuq , B. , Gonçalves , F. , Mas , J.F. , Vareille , L. and Abecassis , J. 2003 . Effects of moisture content and temperature of spaghetti on their mechanical properties . Journal of Food Engineering , 59 ( 1 ) : 51 – 60 .