Abstract
A polyphenol oxidase was isolated from Agaricus bisporus using ammonium sulfate precipitation, combined with DEAE–Sepharose Fast Flow chromatography and Phenyl Sepharose CL-4B chromatography. The molecular weight of the purified polyphenol oxidase was determined to be about 43 kDa with N-terminal sequence Ala-Thr-Asn-Ser-Gly-Thr-Leu-Ile-Ile-Phe by Edman degradation. The optimum temperature and pH for the polyphenol oxidase activity was 20°C and a pH of 6.5–7.0. Moreover, it was stable at 30 and 40°C over a 60 min pre-incubation time period. The Km and Vmax values of this polyphenol oxidase for catechol were 0.67 mM and 3333 U/ml min−1, respectively. In addition, following the cross linking with polyphenol oxidase, the emulsifying activity index, foam capacity, and foam stability of the whey proteins were evaluated to be modified. This work was useful to better understand polyphenol oxidase from A. bisporus, and may lead to its practical use in the future.
INTRODUCTION
Polyphenol oxidase (EC 1.14.18.1, PPO), also often called tyrosinase, usually occurring in plants, fruits, and vegetables, is an enzyme that initiates an enzymatic browning reaction. This enzyme catalyzes both the oxygen-dependent hydroxylation of monophenols to their corresponding o-diphenols and the oxidation of o-diphenols to their cognate o-quinones.Citation[1] Quinones are highly reactive electrophilic molecules that can polymerize, leading to the formation of brown or black pigments.Citation[2] The undesirable enzymatic browning in food processing and post-harvest physiology can reduce nutritional and commercial values.
Currently, PPO has been investigated in many kinds of plants and fungi, such as butter lettuce,Citation[2] Napoleon grape,Citation[3] potato,Citation[4] coffee,Citation[5] marula fruit,Citation[6] artichoke heads,Citation[7] longan fruit,Citation[8] tobacco,Citation[9] banana,Citation[10] Rosmarinus Officinalis,Citation[11] wheat flour,Citation[12] Portabella mushrooms,Citation[13] Ferula sp.,Citation[14] Trametes sp.,Citation[15] and pholiota nameko.Citation[16] Tyrosinase from Agaricus bisporus is liable to progress to enzymatic browning and pigmentation during development and post-harvest storage, which particularly decreases the commercial value of the product.Citation[17] Otherwise, in the last years, enzymatic cross-linking of proteins had gained increasing interest in food technology. PPO had been used for in vitro cross-linking of several proteins, including lysozyme, α-lactalbumin, and β-lactoglobulin;Citation[18] mussel polyphenolic proteins;Citation[19] pork meat;Citation[20] gliadin;Citation[21] and tropocollagen.Citation[22] The aim of this work was to purify and characterize PPO from A. bisporus, including optimum temperature and thermal stability, optimum pH, metal ions effect, and kinetic parameters (Km and V max values), and to apply PPO in cross linking of whey proteins. It will be useful to better understand PPO from A. bisporus, and may lead to its practical use in the future.
MATERIALS AND METHODS
Materials
A. bisporus was purchased at commercial maturity from a local market. DEAE Sepharose Fast Flow and Phenyl Sepharose CL-4B were obtained from GE Healthcare (Bucks, United Kingdom). Caffeic acid was purchased from Sigma-Aldrich (MO, USA). All other chemicals were of analytical grade.
Enzyme Extraction and Isolation
All procedures were carried out at 4°C unless otherwise stated. The fruit bodies of A. bisporus were frozen in liquid nitrogen and ground in mortar. The powder was extracted with cold acetone(−20°C), then vacuum filtered and lyophilized. The acetone powder was homogenized in 0.05 M sodium phosphate buffer, pH 6.5 for 20 min. After centrifugation at 7000 g for 20 min, the supernatant was fractionated with solid ammonium sulfate (between 30 and 70% saturation) and the pellet was collected by centrifugation at 7000 g for 20 min, followed by being re-dissolved in 0.05 M sodium phosphate buffer (pH 6.5) and dialyzed against the same buffer overnight.
The dialysate was transferred to a DEAE–Sepharose Fast Flow column (2.0 × 34 cm), equilibrated with 0.05 M sodium phosphate buffer, pH 6.5. Then, the column was eluted with the equilibration buffer at a flow rate of 30 ml h−1 with a linear gradient of 0 to 0.2 M NaCl dissolved in a total volume of 500 ml equilibration buffer. The eluate was collected in a tube as 5 ml volume. The active fractions were pooled, followed by adding NaCl to 0.5 M and was loaded onto a Phenyl Sepharose CL-4B column (2.0 × 25 cm) equilibrated with 0.5 M NaCl in sodium phosphate buffer (pH 6.5). The adsorbed enzyme was eluted at a flow rate of 30 ml h−1 with a linear gradient of 0.5 M to 0 M NaCl in sodium phosphate buffer (pH 6.5) in a total volume of 500 ml. Finally, the eluate was fractioned into 5 ml volume, and the active fractions were pooled and concentrated for further use.
N-Terminal Amino Acid Sequencing
Purified PPO was electrophoresed in SDS-PAGE gel and then transferred from the gel to a polyvinylidene fluoride membrane by electroblotting at 70 mA of constant current for 1 h. After the membranes were stained with Coomassie brilliant blue R-250 and destained with 50% methanol, the target band was submitted to Peking University for N-terminal amino acid sequencing by Edman degradation using a ABI Procise 491 protein sequencer. Subsequently, sequence data were compared with the protein databases using the BLAST program (http://www.ncbi.nlm.nih.gov/blast/).
Effect of Temperature on PPO Activity and Stability
For determination of optimum temperature of PPO activity, the substrate solution was incubated over a temperature range of 10–40°C. After equilibration of the substrate solution at the temperature, the enzyme was added for the enzyme activity measurement. The thermal stability was determined by pre-incubating the enzyme in a temperature range of 30–60°C with a variation of incubation time from 10 to 60 min, followed by the samples being submitted for determination of residual activity.
Effect of pH and Metal Ions on Enzyme Activity
The PPO activity was assayed on the basis of the standard assay method modified by changing the buffer to obtain the desired pH. The buffers used were as follows: 0.05 M citrate buffer in a pH range of 3.0–5.5; 0.05 M phosphate buffer in a pH range of 6.0–8.0; and 0.05 M Tris buffer in a pH range of 8.5–10.0. PPO activity was measured in the presence of various metal ions (final concentration 5 mM), including K+, Na+, Mg2+, Cu2+, Mn2+, Zn2+, Al3+, and Ba2+. Activity of control was measured at the absence of metal ions and regarded as 100%. In this part, 0.04 M catechol solution in distilled water was used as the substrate.
Enzyme Kinetics
In order to obtain Michaelis constant (Km ) and maximum velocity (V max), the enzyme activity was measured using catechol as the substrate under various concentrations, at pH 7.0 and 20°C. Accordingly, the Km and V max values of the enzyme were measured following the method of Lineweaver and Burk.Citation[23]
Cross Linking of Whey Proteins with PPO from A. bisporus
Concentrated whey proteins were obtained from skim milk by acid method with the pH at 4.6Citation[24] and then adjusted the acidity to pH 4 using 0.1 N HCl. Caffeic acid was dissolved in ethanol as a 0.1 M solution. Then, the caffeic acid and 200–1400 U/ml PPO were added to whey proteins solution, followed by adding distilled water to the mixture for adjusting the final concentration of whey proteins to 10 mg/ml. Finally, the mixture was incubated at 40°C for 4 h. In control samples, caffeic acid and PPO solutions were substituted by distilled water.
The conditions of cross linking were as follows: 1% whey proteins, pH 4, PPO activity 600 U/ml, caffeic acid 1 mM, 40°C for 4 h. Emulsifying activity index (EAI) and emulsifying stability index (ESI) were determined by the method of Liu et al. with a little modification.Citation[25] A volume of 3 ml soybean oil was added to 10 ml cross linking whey proteins, followed by homogenization at a speed of 10,000 g with an omnimixer for 2 min. Then, 10 μl of emulsion was pipeted from the bottom of the container into 5 ml of 0.1% sodium dodecyl sulfate (SDS) (w/v) solution immediately (0 min) and 10 min after homogenization. Finally, absorbance of the SDS solution was measured at 500 nm. EAI and ESI were calculated by the equation:
where T = 2.303, dilution factor = 501, C is the weight of protein per unit volume (g/ml), and ϕ is the oil volumetric fraction.
Foam capacity and foam stability of protein were tested according to the method of Giménez et al. with a little modification.Citation[26] Ten milliliters of cross linking whey proteins were homogenized at a speed of 10,000 g with an omnimixer for 2 min. The total volume was measured at 0, 30, 60, 90, and 120 min, respectively, after whipping. Foam capacity was expressed as foam expansion at 0 min, which was calculated with the following equation:
where A and B is the volume (ml) after or before whipping, respectively. Foam stability was calculated as follows:
where A is the volume (ml) at 30, 60, 90, and 120 min after whipping and B is the volume (ml) before whipping.
Assay of Enzyme Activity
PPO activity was determined by measuring the initial rate of quinone formation, as indicated by an increase in absorbance at 420 nm.Citation[2] One unit of enzyme activity was defined as the amount of enzyme that caused a change in absorbance of 0.001 per min. In regards to the practical testing, the sample cuvette contained 2.8 ml of 0.04 M catechol solution in 0.05 M phosphate buffer (pH 7.0) and 0.2 ml of the enzyme solution, while the blank sample contained only 3 ml of substrate solution. All the measurements for PPO activity were assayed in triplicate.
Protein Determination and Electrophoresis
Protein content was determined according to the method of Lowry using bovine serum albumin as a standard.Citation[27] Sodium dodecylsulfate polyacrylamide gel electrophoresis (SDS-PAGE) was performed following the method of Laemmli with 5% acrylamide stacking gels and 15% acrylamide separating gels, respectively, and protein bands were stained with Coomassie brilliant blue R-250.Citation[28]
Statistical Analysis
Data were analyzed using Microcal Origin 6.0 software (OriginLab Corporation, MA, USA). Every experiment was repeated three times.
RESULTS AND DISCUSSION
Extraction and Isolation of PPO
Purification of PPO from A. bisporus is somewhat difficult due to the small amount of PPO in it. Furthermore, the enzyme is liable to activity loss during isolation at room temperature. Thus, all of the procedures were carried out at 4°C. In order to extract PPO from A. bisporus, (NH4)2SO4 with various salt concentrations was used. PPO was rarely precipitated at 30% of (NH4)2SO4, whereas some other proteins were precipitated. The main PPO activity (about 70%) was recovered in the 70% (NH4)2SO4 fraction. The protein obtained after precipitation with 30–70% ammonium sulphate was further separated by ion-exchange chromatography using DEAE–Sepharose Fast Flow. In order to remove other contaminated proteins, Phenyl Sepharose CL-4B was used. Finally, a 41.9-fold purification of PPO relative to protein with a yield of 6.5% was achieved ().
Table 1 Purification of polyphenol oxidase from Agaricus bisporus
The molecular weight of the purified PPO was determined to be approximately 43 kDa by SDS-PAGE (). It was in accordance with the report of Wichers et al.,[29] who observed that the molecular weights of tyrosinases from A. bisporus were 43 kDa under reducing and denaturing conditions and 47 kDa under native conditions. Experimentally, the molecular weights of most of PPOs were about 40–70 kDa. For example, Kawamura-Konishi et al. reported that the molecular weight of the tyrosinase from Pholiota nameko was estimated to be 42 kDa by SDS-PAGE.Citation[16] In addition, the molecular weight of PPO from other species had been reported as follows: portabella mushrooms, 70 kDa;Citation[13] butter lettuce, 60 kDa;Citation[2] Indian tea leaf, 72 kDa;Citation[30] and mulberry, 65 kDa.Citation[31] Additionally, the N-terminal sequence of the enzyme was determined to be ATNSGTLIIF. Then the sequence data were compared with the protein databases using the BLAST program (http://www.ncbi.nlm.nih.gov/blast/). It indicated that there was no matching with the sequence in the reported PPOs, and we think the enzyme should be a new PPO. The further identification is ongoing.
Effect of Temperature on PPO Activity and Stability
To study the influence of temperature on PPO activity, a temperature range from 10 to 40°C was selected (). The optimum temperature for PPO activity was 20°C, and the enzyme still possessed good activity at 15–30°C (more than 90% of that at 20°C). While the activity of PPO from butter lettuce,Citation[2] longan fruit,Citation[8] artichoke heads,Citation[7] and tobaccoCitation[9] was optimal at 35, 35, 25, and 40°C, respectively. Moreover, it has been reported that the optimum temperature for PPO activity from both tissues and fruit endosperm of coffee was 30°C,Citation[5] while the optimum temperature for melon PPO was as high as 60°C in both ‘Charentais’ and ‘Amarillo’ cultivars.Citation[32] Accordingly, it is clear that the optimum temperatures for PPO are quite species- and substrate-dependent.Citation[33]
Figure 2 Characterization of PPO activity from Agaricus bisporus. (a) Effect of temperature on the PPO activity of Agaricus bisporus. (b) Heat inactivation of PPO at different temperatures using catechol as substrate. (c) Determination of the optimum pH of the PPO activity from Agaricus bisporus measured using catechol as substrate. (d) Effect of metal ions on the activity of PPO from Agaricus bisporus. (Color figure available online.)
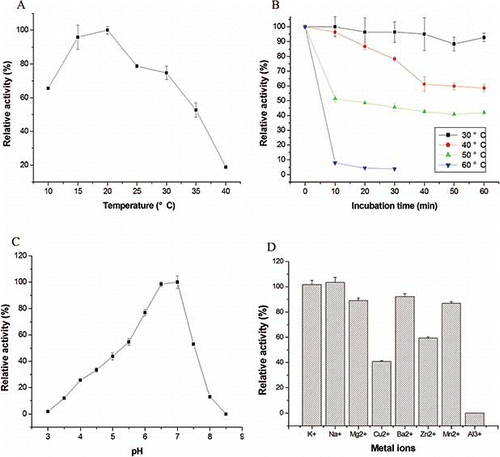
The thermal stability profiles of the PPO, presented as residue activity after pre-incubation at different temperatures for various periods of time, were shown in . The profiles were characterized by a rapid decline in activity on exposure to heat, followed by a more gradual decrease in activity on continued exposure in high temperatures. The enzyme was stable at 30 and 40°C over a 60 min pre-incubation time period. When pre-incubated at 50°C for 60 min, about 42% activity was preserved. Interestingly, the enzyme activity was almost lost (7.7%) when the temperature was up to 60°C; whereas marula fruit PPO was relatively heat stable at 60°C,Citation[6] and PPO of artichoke heads was excellently thermal stable. About 20% PPO activity of artichoke heads was preserved when pre-incubated at 70°C for 10 min.Citation[7] To our surprise, PPO from Napoleon grape was highly heat stable, 80% of activity remaining at 70°C for 5 min.Citation[3] Upon the analysis, it is concluded that A. bisporus PPO was relatively less thermal stable than PPOs from other sources.
Effect of pH and Metal Ions on PPO Activity
The purified PPO showed a maximum activity between pH 6.5–7.0 (). However, Gouzi and Benmansour indicated that PPO activity from Agaricus bisporus Imbach showed two pH optima, at pH 5.3 and 7.0.Citation[34] This may be the reason that the enzyme extract in this work contained more than one enzyme. Actually, most plants showed maximum PPO activity at or near neutral pH values. From the literature, we could find that the optimum pH values were 6.0 for purified marula PPOCitation[6] and Napoleon grape PPO,Citation[3] 6.5 for longan fruit PPO.Citation[8] Aydemir observed that the optimum pH for artichoke PPO was very broad (5.0–7.0).Citation[7] Besides, the optimum pH for PPO activity varies depending on the extraction method, the substrates used for assay, and the localization of the enzyme in the plant cell.Citation[35]
In respect to metal irons, showed that K+ and Na+ had slight stimulating effects on the enzyme (1.02- and 1.05-fold of the control). Ba2+, Mn2+, and Mg2+ showed slight inhibition on PPO activity (93.8, 89.2, and 86.8% of the control, respectively), while Cu2+ and Zn2+ showed strong inhibition on PPO activity (40.7 and 59.3% of the control, respectively). Interestingly, the enzyme was completely inactivated when Al3+ was added. In contrast, 30% decrease in artichoke heads’ PPO activity was observed at 10 mM concentration of MnCl2, CoCl2, CaCl2, and NiCl2.Citation[7] Furthermore, PPO activity from longan fruit was markedly inhibited by FeSO4 and SnCl2, while promoted by MnSO4 and CaCl2.Citation[8] In addition, Motoda reported that Hg2+ and Sn2+ inhibited both PPOs from Trametes sp. MS39401 by about 70%, while Cu+, Cd2+, and A13+ slightly inhibited PPO-1.Citation[15] In regard to PPO-2, Cu+ could slightly inhibit its activity, while Cu2+ had no effect on PPO-1.
Enzyme Kinetics of PPO
Km and V max values of the PPO were obtained from the Lineweaver-Burk plots, using catechol as substrate (). The Km and V max values of the PPO for catechol were 0.67 mM and 3333 U/ml min−1, respectively. However, the kinetic parameters of PPO from A. bisporus imbach were: Km = 1.4 mM, V max = 78 U/ml min−1 for pyrogallol and Km = 0.4 mM, V max = 168 U/ml min−1 for pyrocatechol.Citation[34] In addition, the Km values and V max obtained for PPO towards catechol from butter lettuce were 3.2 mM and 4081 U/ml min−1.Citation[2] It indicated that the enzyme kinetics of PPO were different among species.
Figure 3 The Lineweaver–Burk plots of PPO activity measured with catechol as substrate. The concentration of catechol was 1, 2, 3, 4, 5, and 6 mM, respectively. (Color figure available online.)
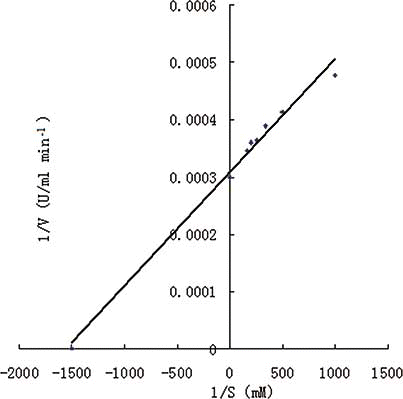
Furthermore, the difference can exist also between substrates. For example, the kinetic parameters of PPO from artichoke heads were: Km = 10.2 mM, V max = 19,662 U/ml min−1 for catechol; Km = 12.4 mM, V max = 12,500 U/ml min− for 4-methylcatechol; Km = 43.3 mM, V max =4620 U/ml min−1 for gallic acid.Citation[7] All in all, enzyme kinetics of PPO are individual dependent.
Cross Linking of Whey Proteins with PPO
To elucidate the effect of PPO activity on cross linking of whey proteins, the PPO activity of 200–1400 U/ml in the reaction mixture was added progressively. The intensity of oligomer was up to the highest at 600 U/ml, while the quantity of polymers did not increase accordingly when the PPO activity was above 600 U/ml in the reaction mixture (). After cross linking, the emulsifying activity index (EAI) of whey proteins reached 20.2 m2/g, whereas the EAI of whey proteins without cross linking was just 18.2 m2/g. In addition, the emulsion stability index (ESI) of cross linked whey proteins was 11.3 min, which was similar to that of whey proteins without cross linking (11.1 min).
Figure 4 Effect of PPO activity on the cross linking of whey proteins in the presence of caffeic acid. The conditions of cross linking were as follows: caffeic acid 1 mM, pH 4, 40°C for 4 h, PPO activity 200–1400 U/ml. M: marker; Lane 1: control sample; Lane 2: 200 U/ml; Lane 3: 400 U/ml; Lane 4: 600 U/ml; Lane 5: 800 U/ml; Lane 6: 1000 U/ml; Lane 7: 1200 U/ml; Lane 8: 1400 U/ml.
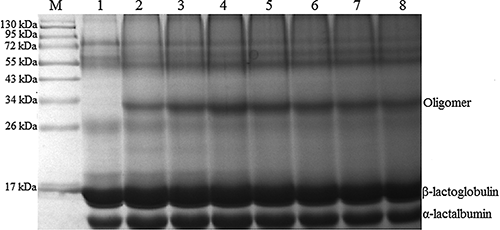
As mentioned for foaming properties, the foam capacity and foam stability of cross linked whey proteins were increased obviously (). After cross linking, the foam capacity of whey proteins was 144.5%, which was higher than that of whey proteins without cross linking (134.2%), and the foam stability was also very high. The foam stability still reached to 99.5% at 120 min late after whipping, but that of the control was just 49%. Comparing the cross linking whey protein and the control sample, the percentage of cross linking protein is very low by judging the amount of oligomer in the SDS-PAGE profile (). However, the functional properties of whey proteins changed greatly. All the changes will contribute to the modified characteristics of whey protein, maybe leading to a novel ingredient. Consequently, whey protein can be modified by the purified PPO, resulting in variation in properties. Moreover, the PPO has the potential to be applied to the modification of whey protein in the food industry.
CONCLUSIONS
Polyphenol oxidase can be derived from many plants and fungi, and also several kinds of PPO are commercially available. However, there is still much variation of PPO existing naturally including in A. bisporus because of the diversity of PPO genes. Fortunately, we purify PPO successfully from A. bisporus by ammonium sulfate precipitation combined with DEAE–Sepharose Fast Flow chromatography and Phenyl Sepharose CL-4B chromatography, and a 41.9-fold purification of PPO with a yield of 6.5%, on the basis of protein analysis, was achieved. Since the identified N-terminal sequence of this PPO cannot match totally with the existed date within the protein databases of GenBank, it should concluded that the purified PPO from A. bisporus was a new one. The enzymatic characterization of the purified PPO was defined well, and it may help to better understand the PPO. Moreover, the emulsifying activity index, foam capacity, and foam stability of the enzymatic cross-linked whey proteins by the PPO have significant changes, and it implicated that the purified PPO might be used for the modification of whey protein in the future.
ACKNOWLEDGMENTS
International Science & Technology Cooperation Program of China (No. 2013DFG31380), National Science and Technology. Support Project, China (No. 2012BAK17B02, 2011BAK10B03), National High Technology Research and Development Program of China (863 Program, No. 2013AA102205), and National Natural Science Foundation of China (No. 31060215).
REFERENCES
- Mayer , A.M. and Harel , E. 1979 . Polyphenol oxidase in plants . Phytochemistry , 18 : 193 – 215 .
- Gawlik-Dziki , U. , Zlotek , U. and Swieca , M. 2008 . Characterization of polyphenol oxidase from butter lettuce (Lactuca sativa var. capitata L.) . Food Chemistry , 107 : 129 – 135 .
- Nunez-Delicado , E. , Serrano-Megas , M. , Perez-Lopez , A.J. and Lopez-Nicolas , J.M. 2007 . Characterization of polyphenol oxidase from Napoleon grape . Food Chemistry , 100 : 108 – 114 .
- Marri , C. , Frazzoli , A. , Hochkoeppler , A. and Poggi , V. 2003 . Purification of a polyphenol oxidase isoform from potato (Solanum tuberosum) tubers . Phytochemistry , 63 : 745 – 752 .
- Mazzafera , P. and Robinson , S.P. 2000 . Characterization of polyphenol oxidase in coffee . Phytochemistry , 55 : 285 – 296 .
- Mdluli , K.M. 2005 . Partial purification and characterisation of polyphenol oxidase and peroxidase from marula fruit (Sclerocarya birrea subsp. Caffra) . Food Chemistry , 92 : 311 – 323 .
- Aydemir , T. 2004 . Partial purification and characterization of polyphenol oxidase from artichoke (Cynara scolymus L.) heads . Food Chemistry , 87 : 59 – 67 .
- Jiang , Y.M. 1999 . Purification and some properties of polyphenol oxidase of longan fruit . Food Chemistry , 66 : 75 – 79 .
- Shi , C. , Dai , Y. , Xu , X. , Xie , Y. and Liu , Q. 2002 . The purification of polyphenol oxidase from tobacco . Protein Expression and Purification , 24 : 51 – 55 .
- Wuyts , N. , De Waele , D. and Swennen , R. 2006 . Extraction and partial characterization of polyphenol oxidase from banana (Musa acuminata Grande naine) roots . Plant Physiology and Biochemistry , 44 : 308 – 314 .
- Aydemira , T. 2010 . Selected kinetic properties of polyphenol oxidase extracted from Rosmarinus officinalis L . International Journal of Food Properties , 13 : 475 – 485 .
- Yadava , D.N. , Patkia , P.E. , Sriharia , S.P. , Sharmaa , G.K. and Bawaa , A.S. 2010 . Studies on polyphenol oxidase activity of heat stabilized whole wheat flour and its chapatti making quality . International Journal of Food Properties , 13 : 142 – 154 .
- Fan , Y. and Flurkey , W.H. 2004 . Purification and characterization of tyrosinase from gill tissue of Portabella mushrooms . Phytochemistry , 65 : 671 – 678 .
- Erat , M. , Sakiroglu , H. and Kufrevioglu , O.I. 2006 . Purification and characterization of polyphenol oxidase from Ferula sp . Food Chemistry , 95 : 503 – 508 .
- Motoda , S. 1999 . Purification and characterization of polyphenol oxidase from Trametes sp. MS39401 . Journal of Bioscience and Bioengineering , 87 ( 2 ) : 137 – 143 .
- Kawamura-Konishi , Y. , Tsuji , M. , Hatana , S. , Asanuma , M. , Kakuta , D. , Kawano , T. , Mukouyama , E.B. , Goto , H. and Suzuki , H. 2007 . Purification, characterization, and molecular cloning of tyrosinase from Pholiota nameko . Bioscience Biotechnology and Biochemistry , 71 ( 7 ) : 1752 – 1760 .
- Seo , S.Y. , Sharma , V.K. and Sharma , N. 2003 . Mushroom tyrosinase: Recent prospects . Journal of Agricultural and Food Chemistry , 51 ( 10 ) : 2837 – 2853 .
- Thalmann , C. and Lötzbeyer , T. 2002 . Enzymatic cross-linking of proteins with tyrosinase . European Food Research and Technology , 214 ( 4 ) : 276 – 281 .
- Burzio , L.A. , Burzio , V.A. , Pardo , J. and Burzio , L.O. 2000 . In vitro polymerization of mussel polyphenolic proteins catalyzed by mushroom tyrosinase . Comparative Biochemistry and Physiology, Part B , 126 ( 3 ) : 383 – 389 .
- Lantto , R. , Plathin , P. , Niemist , M. , Buchert , J. and Autio , K. 2006 . Effects of transglutaminase, tyrosinase and freeze-dried apple pomace powder on gel forming and structure of pork meat . LWT–Food Science and Technology , 39 ( 10 ) : 1117 – 1124 .
- Takasaki , S. , Kawakishi , S. , Murata , M. and Homma , S. 2001 . Polymerisation of gliadin mediated by mushroom tyrosinase . LWT–Food Science and Technology , 34 ( 8 ) : 507 – 512 .
- Dabbous , M.K. 1966 . Inter- and intramolecular cross-linking in tyrosinase-treated tropocollagen . Journal of Biological Chemistry , 241 ( 22 ) : 5307 – 5312 .
- Lineweaver , H. and Burk , D. 1934 . The determination of enzyme dissociation constant . Journal of the American Chemical Society , 56 : 658 – 661 .
- Li , X. , Luo , Z. , Chen , H. and Cao , Y. 2008 . Isolation and antigenicity evualution of β-lactoglobulin from buffalo milk . African Journal of Biotechnology , 7 : 2258 – 2264 .
- Liu , C. , Wang , X. , Ma , H. , Zhang , Z. , Gao , W. and Xiao , L. 2008 . Functional properties of protein isolates from soybeans stored under various conditions . Food Chemistry , 111 : 29 – 37 .
- Giménez , B. , Alemán , A. , Montero , P. and Gómez-Guillén , M.C. 2009 . Antioxidant and functional properties of gelatin hydrolysates obtained from skin of sole and squid . Food Chemistry , 114 : 976 – 983 .
- Lowry , O.H. , Rosebrough , N.J. , Farr , A.L. and Randall , R.J. 1951 . Protein measurement with the Folin phenol reagent . Journal of Biological Chemistry , 193 : 265 – 275 .
- Laemmli , U.K. 1970 . Cleavage of structural proteins during the assembly of the head Bacteiofage T4 . Nature , 227 : 680 – 685 .
- Wichers , H.J. , Gerritsen , Y.A.M. and Chapelon , C.G.J. 1996 . Tyrosinase isoforms from the fruit bodies of Agaricus bisporus . Phytochemistry , 43 ( 2 ) : 333 – 337 .
- Halder , J. , Tamuli , P. and Bhaduri , A.N. 1998 . Isolation and characterization of polyphenol oxidase from Indian tea leaf (Camellia sinensis) . Journal of Nutritional Biochemistry , 9 ( 2 ) : 75 – 80 .
- Arslan , O. , Erzengin , M. , Sinan , S. and Ozensoy , O. 2004 . Purification of mulberry (Morus alba L.) polyphenol oxidase by affinity chromatography and investigation of its kinetic and electrophoretic properties . Food Chemistry , 88 : 479 – 484 .
- Chisari , M. , Barbagallo , R.N. and Spagna , G. 2008 . Characterization and role of polyphenol oxidase and peroxidase in browning of fresh-cut melon . Journal of Agricultural and Food Chemistry , 56 ( 1 ) : 132 – 138 .
- Özen , A. , Colak , A. , Dincer , B. and Güner , S. 2004 . A diphenolase from persimmon fruits (Diospyros kaki L., Ebenaceae) . Food Chemistry , 85 ( 3 ) : 431 – 437 .
- Gouzi , H. and Benmansour , A. 2007 . Partial purification and characterization of polyphenol oxidase extracted from Agaricus bisporus (J. E. Lange) Imbach . International Journal of Chemical Reactor Engineering , 5 ( 1 ) : A76
- Alyward , F. and Haisman , D.R. 1969 . Oxidation system in fruits and vegetables—Their relation to the quality of pressured products . Advances in Food Research , 17 : 1 – 7 .