Abstract
Starch is the main carbohydrate in human nutrition. Starch digestibility can vary from a rapid digestion to indigestibility. Therefore, postprandial glycaemic control in type 2 diabetics is of great interest in the context of worldwide health concerns. Although powerful synthetic inhibitors of starch digestive enzymes, such as acarbose, are available to control postprandial hyperglycemia, plant-based enzyme inhibitors are potentially safer. Natural enzyme inhibitors, such as wheat albumin, the Phaseolus vulgaris α-amylase inhibitor, and several phenolic compounds, have the potential to serve as a remedy against hyperglycemia-induced chronic diseases. The inhibition of α-amylase and α-glucosidase is mediated by different phenolics found in varieties of raspberry. Maltase inhibitory activities of chebulagic acid and chebulinic acid from fruit of Terminalia chebula are comparable to that of acarbose. The Nepalese herb Pakhanbhed (Bergenia ciliata) phenolics, (-)-3-O-galloylepicatechin and (-)-3-O-galloylcatechin, showed effective inhibition against starch digesting enzymes. In separate studies, oral administration of starch and maltose with persimmon (Diospyros kaki) leaf tea proanthocyanidins [containing (-)-epigallocatechin-3-O-gallate] and black/bitter cumin (Centratherum anthelminticum) seed phenolics, respectively, resulted in a significant and dose-dependent decrease in the blood glucose level in Wistar rats. Co-application of phenolics with synthetic enzyme inhibitors may reduce the effective dose of synthetic inhibitors required in the regulation of starch digestion. Several phenolic compounds might be useful functional food components and could contribute to manage both hyperglycemia and proper cellular redox status. Human dose-selecting studies and well-controlled long-term human studies would help to optimize the beneficial effects of phenolic compounds.
INTRODUCTION
Diabetes mellitus (DM) is one of the most serious and chronic diseases due to an improper balance of glucose homeostasis. Currently, an estimated more than 220 million people worldwide have diabetes.[Citation1] The death toll from diabetes will increase to double between 2005 and 2030.[Citation1] Two types of DM are presently known, one being type 1, which is characterized by insufficient insulin production and the other being type 2, that results from ineffectiveness of insulin.[Citation1,Citation2] Worldwide, type 2 diabetes accounts for approximately 90% of the total cases.[Citation1] A condition characterized by an abnormal postprandial increase of blood glucose level, known as hyperglycemia or raised blood sugar, has been linked to the onset of type 2 diabetes[Citation2] and is the main cause of complications related with cardiovascular disease, renal failure, blindness, neurological complications, etc.[Citation3] Recently, type 2 diabetes is also being found in children.[Citation1] Thus, the increasing trend in type 2 diabetes mellitus has become a serious medical concern worldwide.
Rapidly digested carbohydrates result in a quick increase in the post-meal blood glucose level.[Citation4] Regular consumption of rapidly digestible carbohydrates is the main cause for the development of hyperglycemia-induced chronic diseases.[Citation5] Chronic postprandial hyperglycemia induces the glycosylation of proteins;[Citation6] hyperglycemia triggers the generation of free radicals leading to oxidative stress when excessive free radicals react with proteins and DNA[Citation7,Citation8] and increases plasma insulin and lipid concentrations along with cardiovascular disease risk factors[Citation9] ().
Flow Chart 1 Potential connections between meal-associated hyperglycemia and the increase in cardiovascular disease risk.
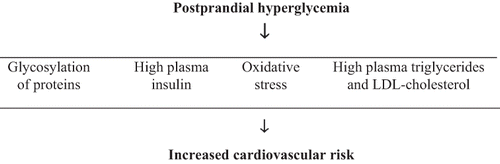
Starch is the most abundant storage polysaccharide in plants. Starch consists of amylose (linear polymer of α-D-glucose units linked by α-1,4 glycosidic linkages) and amylopectin (branched polymer of α-D-glucose units linked by α-1,4 and α-1,6 glycosidic linkages). Pancreatic α-amylase and intestinal α-glucosidase are the two key enzymes involved in starch digestion.[Citation5] Starch is hydrolyzed by amylases to α-dextrins or oligosaccharides (maltose, maltotriose) that are further hydrolyzed to glucose by intestinal α-glucosidase before being absorbed in the duodenum and upper jejunum. Starch and starchy food products can be classified based on their digestibility.[Citation10] Post-meal glycaemic control in type 2 diabetics due to ingestion of starchy food is of great interest in the context of worldwide health concerns.
An important therapeutic approach for treating type 2 diabetes is to decrease the post-prandial hyperglycemia by retarding the absorption of glucose through the inhibition of the enzymes, α-amylase and α-glucosidase, in the digestive tract. Enzyme inhibitors delay the rate of glucose absorption by preventing carbohydrate digestion and consequently dulling the postprandial plasma glucose rise.[Citation11] The inhibition of starch digestive enzymes by synthetic agents, such as acarbose, is an important clinical strategy for controlling postprandial glycemia.[Citation4, Citation10, Citation12, Citation13] Though acarbose (), which is approved by the Food and Drug Administration, reduces blood glucose levels, this inhibitor is reported to cause critical side effects, such as liver disorders, while plant-based enzyme inhibitors are potentially safer.[Citation6–12] The natural enzyme inhibitors, the Phaseolus vulgaris α-amylase inhibitor (α-AI) and wheat albumin (WA), have been known for a long time and have been well-discussed previously.[Citation12, Citation14, Citation15] Some dietary supplements used to control obesity are based on protein concentrates from kidney bean (Phaseolus vulgaris), which are known to contain significant levels of α-amylase inhibitor also known as phaseolamin (α-AI). α-Amylase inhibitor (α-AI) is known to be effective in preventing postprandial hyperglycemia by blocking access to the active site of the starch digestive enzyme.[Citation12] WA can inhibit starch digestion, resulting in improved overall glycemic status in type 2 diabetic patients with mild hyperglycemia without the occurrence of critically adverse effects, such as hepatic disorders.[Citation14]
More than 80% of diabetes deaths occur in developing countries.[Citation1] Plants continue to play a vital role in the treatment of type 2 diabetes, particularly in developing countries where most people do not have an access to modern treatment. The use of plant-based enzyme inhibitors is also encouraged in developed countries because there is concern about the critical side effects of synthetic pharmaceutical agents. To either avoid or decrease the adverse effects of currently used synthetic agents, it is necessary to utilize naturally occurring α-amylase and α-glucosidase inhibitors. Keeping this in view, the present review mainly focuses on the possibilities of phenolic compounds as α-amylase and α-glucosidase inhibitors from some underutilized plants (or only localized in a particular region) for overcoming hyperglycemia-induced chronic diseases, such as type 2 diabetes.
METABOLIC HETEROGENEITY OF STARCH
Based on kinetics of in vitro digestion of starch (by simulating stomach and intestinal conditions), different starch fractions are defined as:[Citation16] (i) Rapidly digestible starch (RDS): amount of glucose release after 20 min of in vitro digestion; (ii) Slowly digestible starch (SDS): amount of glucose released between 20 and 120 min; (iii) Resistant starch (RS): RS is the starch not hydrolyzed after120 min of incubation. RS is defined as that fraction of dietary starch, which escapes digestion in the small intestine, and passes to the large intestine for fermentation. It is measured chemically as the difference between total starch (TS) and the sum of RDS and SDS:
Of relevance to the postprandial glycemic control in type 2 diabetes, the nature of the starch in the dietary foods is important. Structural characteristics of starch vary among different botanical sources. Raw starches high in amylose have been shown to digest more slowly than those high in amylopectin.[Citation10] The ratio of amylose to amylopectin molecules in the starchy food plays a vital role to control postprandial glucose and insulin concentrations.[Citation17] It was found that high amylose products induced low blood glucose and insulin responses when compared with similar products high in amylopectin.[Citation18] Amylopectin is a much larger molecule than amylose. The average molecular weight of amylopectin is between 105 and 106, whereas the average molecular weight for amylose is 104. The large surface area of amylopectin makes it a suitable substrate for amylolytic attack.[Citation10] In addition, lower availability of amylose for amylolytic attack than amylopectin is due to more bound glucose chains (to each other by hydrogen bonds) in amylose polymers.[Citation10]
High-amylose starches require high temperatures (up to 150°C) in the presence of water to become fully gelatinized[Citation17] and making it more easily available for enzymatic attack.[Citation10] It is usually not possible to reach this temperature under normal cooking. A higher content of amylose lowers the digestibility of starch because there is a positive correlation between amylose content and the formation of RS.[Citation19] Granfeldt et al.[Citation20] reported that high amylose (70%) corn flour had RS of 20 g/100 g dry matter than ordinary corn flour (25% amylose) that had RS of 3 g/100 g dry matter. RS is highly resistant to mammalian enzymes.
PHENOLIC COMPOUNDS
Recently, phenolic compounds have been receiving much attention for controlling the digestibility of starch and for having a high antioxidant activity. They are widely distributed in plant foods, and their biological activities depend on their chemical structures, doses, and time of consumption. Phenolic compounds belong to the families of phenolic acids, flavonoids, and others.[Citation21] Among them, flavonoids represent the widely distributed group of plant phenolics. The flavonoids include the anthocyanin pigments, flavonols, flavones, flavanols, and isoflavones. The flavanols tend to polymerize to condensed tannins, whereas other flavonoids occur mainly as glycosides.[Citation21]
Plant tannins are natural polyphenolic compounds of high molecular weight. Tannins are subdivided into hydrolyzable and condensed tannins. Hydrolyzable tannins are molecules with a polyhydroxy component.[Citation22] It is found that the hydroxyl groups of these molecules are esterified with phenolics, for example, ellagic acid (ellagitannins). Condensed tannins are formed through the condensation of flavanols and are also known as proanthocyanidins. Catechin, epicatechin, and gallocatechin are the monomeric constituents of the condensed tannins.[Citation21] Polyphenols, especially tannins, have a high antioxidant activity.[Citation8, Citation23, Citation24] Improved body antioxidant status can protect against degenerative diseases.
ENZYME INHIBITORS FROM PLANT ORIGINS
Pakhanbhed (Bergenia ciliata)
The Nepalese herb Bergenia ciliata is known as ‘‘Pakhanbhed” in the Nepali language, and is used in traditional ayurvedic medicine as a remedy against various diseases in an indigenous community in Nepal.[Citation25,Citation26] Bhandari et al.[Citation27] have investigated the anti-diabetic activity of Bergenia ciliata using an in vitro model. Investigation of the ethyl acetate soluble extract of B. ciliata revealed that two active compounds, (-)-3-O-galloylepicatechin and (-)-3-O-galloylcatechin, are responsible for the strong inhibition of porcine pancreatic α-amylase and rat intestinal maltase activity in a dose-dependent manner[Citation27] (). In addition to their antioxidant activity, catechins are also shown to be inhibitors of starch digestive enzymes.[Citation28,Citation29]
Table 1 Key enzyme inhibitors from plant origins involved in starch digestion
The IC50 value is defined as the concentration of an inhibitor that is required for 50% inhibition of the enzyme activity and it is commonly used for the measurement of inhibitor potency. The IC50 value for rat intestinal maltase and porcine pancreatic α-amylase were 334 and 739 μM, respectively, for (-)-3-O-galloylepicatechin and 150 and 401 μM, respectively, for (-)-3-O-galloylcatechin.[Citation27] In vivo and in vitro models, the α-glucosidase and α-amylase inhibitory activities of these compounds revealed that they have a high potential to serve as a remedy against type 2 diabetes.[Citation28–31 Citation Citation Citation−31 For catechins, their epi forms exhibited significant inhibitory effects and the galloyl moiety also served an important role.[Citation5]
It is found that non-covalent interactions occur between polyphenols and enzymes (proteins).[Citation32] Hydroxyl groups and galloyl groups are present in the molecular structure of polyphenols.[Citation28] The phenolic groups can form hydrogen bonds with the polar groups of enzyme. In contrast, there are many hydrophobic amino acids found in enzymes (protein). Galloyl groups in polyphenols show hydrophobicity[Citation33] and, therefore, polyphenols can bind enzymes through hydrophobic association.[Citation28] The galloyl moiety may play an important role to interact with mammalian α-amylase and α-glucosidase[Citation5, Citation34] and their positions mostly affect the effectiveness.[Citation35] The cooperative effects of hydrophobic association and hydrogen bond formation between polyphenols and the starch digestive enzymes could contribute to control postprandial hyperglycemia in type 2 diabetic patients.
Persimmon (Diospyros kaki)
In addition to green tea, different kinds of tea are prepared from leaves of dietary plants, such as persimmon, guava, and mulberry, by drying and are commercially available as herbal teas in Japan. Based on the degree of astringency at the mature stage, Japanese persimmon cultivars (Diospyros kaki) are classified into two groups, an astringent and a non-astringent type.[Citation36] Kawakami et al.[Citation37] identified polyphenols from an astringent cultivar of persimmon leaf tea as effective an α-amylase inhibitor. Thiolysis and subsequent analysis revealed that the major components were proanthocyanidin, consisting of (+)-catechin, (–)-epigallocatechin, (–)-epigallocatechin-3-O-gallate, (–)-epicatechin, and (–)-epicatechin-3-O-gallate[Citation37] (). Similar proanthocyanidins with the same units were also found in persimmon leaves. Like persimmon tea, green tea is also derived from drying the fresh tea leaves and so no oxidation takes place, resulting in excellent levels of catechins. The (–)-epigallocatechin-3-O-gallate (EGCG) has been recognized as the most beneficial component among the four other catechins isolated from green tea.[Citation38]
The polyphenol concentrate of the persimmon leaf tea had significant α-amylase inhibitory activity in a concentration-dependent manner, but had no considerable effect on maltase. The percentage of inhibition ratios of persimmon leaf tea against porcine pancreas α-amylase were 24.6 ± 3.2, and 45.2 ± 2.8 at the concentrations 24 and 48 μg/ml of polyphenol concentrate powders, respectively.[Citation37] The α-amylase inhibitory strength of persimmon leaves is comparable to that of green tea,[Citation5, Citation37] whereas, compared to green tea,[Citation5] maltase inhibitory potency of persimmon leaves was much less,[Citation37] indicating that the inhibition of α-amylase by persimmon leaves proanthocyanidin appeared to be more relatively specific than green tea proanthocyanidin. Proanthocyanidins are flavan-3-ol polymers with high structural diversity and complexity, and the biological activities of proanthocyanidins depend on their structural features and doses.
When polyphenol concentrates of persimmon leaf tea were orally administered with soluble potato starch, blood glucose levels in Wistar rats decreased in a dose-dependent manner.[Citation37] Eighteen overnight-starved male rats (190–230 g) were divided into three groups. Oral administration of starch (2.0 g/kg of body weight) with polyphenol concentrate of persimmon leaf tea at three different dosages (0, 100, and 300 mg/kg of body weight) resulted in an increase in the blood glucose level from the values of 94 ± 12, 112 ± 9, and 105 ± 15 mg/dl at 0 min to peaks of 155 ± 24, 150 ± 14, and 136 ± 13 mg/dl at 30 min, respectively, and then decreased.[Citation37] Kao et al.[Citation39] suggested that tea catechins, particularly (–)-epigallocatechin-3-O-gallate have the potential for the treatment of hyperglycemia-induced chronic diseases, including type 2 diabetes and obesity.[Citation39] Human studies show anti-diabetic effects of green tea catechins, especially (–)-epigallocatechin-3-O-gallate (EGCG).[Citation40] An epidemiological study conducted in Japan revealed that subjects with an average consumption of more than 6 cups of green tea per day was inversely associated with the risk for type 2 diabetes, compared to those who drank <1 cup per week.[Citation41] In this study, a total of 17,413 subjects were followed up for 5 years. Although, the optimal dose of EGCG for controlling postprandial hyperglycemia has not yet been established, a dose range of 84–386 mg EGCG/day may be sufficient to support the beneficial effects.[Citation42,Citation43]
Proanthocyanidins in rowanberry may contribute greatly for managing hyperglycemia by the inhibition of α-amylase.[Citation44] The inhibition of starch digestive enzymes by acarbose is an important clinical strategy for controlling postprandial glycemia, but it has adverse effects. The effect of rowanberry proanthocyanidins and acarbose were investigated at different combinations of their IC50 values by Grussu et al.[Citation44] Co-addition of rowanberry proanthocyanidins with acarbose reduced the effective concentration of acarbose required for α-amylase inhibition. Although the combined effect of persimmon proanthocyanidins and acarbose were not tested, such synergistic interactions may have implications for the current clinical use of synthetic inhibitors for controlling starch digestion.
Finger Millet (Eleusine coracana L.)
Finger millet (Eleusine coracana L.) or ragi is cultivated in India and many of the African countries. The millet seed coat matter is an excellent source of phenolic compounds (10% w/w). Shobana et al.[Citation6] characterized phenolic compounds from the millet seed coat by HPLC and electrospray ionization mass spectrometry after extraction with acidified methanol and then investigated the ability of phenolics in inhibiting the activities of porcine pancreatic α-amylase and rat intestinal α-glucosidase.[Citation6]
Different types of phenolic compounds, including phenolic acids and polyphenols, such as gallic acid, protocatechuic acid, gentisic acid, caffeic acid, syringic acid, ferulic acid, naringenin, kaempferol, luteolin glycoside, phloroglucinol, apigenin, (+)-catechin/(–)-epicatechin, trans-feruloyl-malic acid, dimer of prodelphinidin (epi/gallocatechins; 2GC), daidzein, catechin gallates, and trimers and tetramers of catechin were identified from the millet seed coat phenolics extract[Citation6] (). The IC50 values for α-glucosidase and pancreatic amylase were 16.9 and 23.5 μg of finger millet seed coat phenolics, respectively,[Citation6] indicating that these phenolics have the potential for managing hyperglycemia. Tadera et al.[Citation45] reported that the starch digestive enzymes were inhibited by naringenin, kaempferol, luteolin, apigenin, (+)-catechin/(–)-epicatechin, daidzein, and epigallocatechin gallate and showed that there is a correlation between the potency of inhibition and the number of hydroxyl groups of flavonoids. Kinetic studies showed that the millet seed coat phenolics non-competitively inhibited the two key enzymes involved in starch digestion.[Citation6] A Korean herb (Rhus chinensis) extract used as a remedy against type 2 diabetes in Korea, exhibited the same type of inhibition on α-glucosidase and pancreatic amylase.[Citation46]
Raspberries (Rubus idaeus L.)
Raspberries (Rubus idaeus L.) are known as an excellent source of antioxidants mainly because of their high levels of phenolics. Wolfe et al.[Citation47] reported that a raspberry extract has the highest antioxidant activity among common fruits in the USA. Zhang et al.[Citation48] assessed the inhibitory activities of phenolics-rich extracts from seven primocane fall-bearing raspberry (Rubus idaeus L.) cultivars Nova, Dinkum, Heritage, Autumn Britten, Josephine, Anne, and Fall Gold, against yeast α-glucosidase, porcine pancreatic α-amylase, and porcine pancreatic lipase. All the raspberry extracts potently inhibit α-glucosidase but no inhibition occurred against α-amylase and lipase. Among the tested raspberry extracts, the ‘Dinkum’ raspberry extract exhibited the highest α-glucosidase inhibitory activity.
Zhang et al.[Citation48] identified four major active phenolic compounds, ellagic acid (phenolic acids), cyanidin diglucoside (anthocyanins), pelargonidin-3-rutinoside (anthocyanins), and catechin (flavonoids), as the α-glucosidase inhibitors by HPLC and liquid chromatography-mass spectroscopy (LC-MS) analysis on the ‘Dinkum’ raspberry extract (). These phenolic compounds exhibited potent inhibition of α-glucosidase with IC50 from 14.7 to 64.5 μg/mL.[Citation48] McDougall et al.[Citation49] reported that fruits rich in anthocyanin strongly inhibited α-glucosidase activity, but the inhibitory potency of fruits' extracts against both salivary and pancreatic amylase was related to their tannin content.[Citation49] Diacylated anthocyanins inhibited α-glucosidase activity and induced an anti-diabetic activity in animal models.[Citation50] Red currants inhibit α-amylase more efficiently than black currants,[Citation51] which possess higher anthocyanin content, indicating that anthocyanins are not crucial for amylase inhibition.[Citation44]
It was found that the phenolic extracts of a specific raspberry (variety ‘Glen Ample’) effectively inhibited activities of α-amylase and α-glucosidase.[Citation49] The inhibitory potency of raspberry extracts against α-glucosidase was associated with their anthocyanin content. The α-amylase inhibitory components in raspberry (variety ‘Glen Ample’) were identified as ellagitannins using LC-MS.[Citation49] Grussu et al.[Citation44] also reported that in vitro, extract from raspberry variety (‘Glen Ample’) was able to inhibit porcine pancreatic α-amylase (IC50 values of 21.0 μg/mL) due to the presence of ellagitannins as major active components.[Citation44] Purified ellagitannins from strawberry also possess α-amylase inhibitory activity.[Citation52] Ellagitannins in raspberry (‘Glen Ample’) have been reported to undergo breakdown partially under simulated gastrointestinal conditions,[Citation53] although a very recent study[Citation44] suggests that raspberry extracts retain amylase inhibitory activity to a high level when subjected to in vitro digestion. The extracts of seven raspberry cultivars (Nova, Dinkum, Heritage, Autumn Britten, Josephine, Anne, and Fall Gold) did not inhibit the activity of pancreatic α-amylase possibly because they effectively lacked ellagitannins in these cultivars. It was found that the concentrations of the major phenolic components in 14 raspberry cultivars varied approximately 20-fold.[Citation54,Citation55]
In vitro results suggest that raspberry phenolics inhibit enzymes involved in starch digestion, such as α-amylase or α-glucosidase, at levels easily achieved in the gastrointestinal tract through normal dietary intake of fruits. Ellagitannins in raspberry (variety ‘Glen Ample’) were the main active components for effective inhibition of mammalian α-amylase and capable of retarding starch digestion. Although it is still not clear whether raspberry α-glucosidase inhibitors can suppress postprandial hyperglycemia, raspberry phenolics greatly inhibited yeast α-glucosidase.
Terminalia chebula Retz
The dried fruit of T. chebula Retz is known as black myrobalan in English and Xi-Qin-Ge or Zhang-Qin-Ge in China. Gao et al.[Citation35] investigated the inhibitory effect of the fruits of T. chebula on mammalian α-glucosidase activity. Three active ellagitannins were identified as chebulanin, chebulagic acid, and chebulinic acid from aqueous methanolic extract of the dried T. chebula fruits and were found to possess strong rat intestinal maltase inhibitory activity (), with the IC50 values of 690, 97, and 36 μM, respectively, but exhibited no inhibitory effects on rat intestinal isomaltase and sucrase activities.[Citation35] These compounds inhibited maltase activity in a dose-dependent manner. The clove ellagitannins were also shown to have maltase inhibitory activity.[Citation34] The rat intestinal maltase inhibitory activity of 1,2,3,4,6-penta-O-galloyl-β-D-glucose (PGG) (IC50 = 140 μM) was lower than that of chebulagic acid and chebulinic acid.[Citation35] PGG is a potent maltase inhibitor and inhibits the activity of maltase in response to the numbers of the galloyl group attached to a glucose core.[Citation34] The IC50 value of acarbose was 0.08 mM for rat intestinal maltase,[Citation56] indicating that chebulagic acid and chebulinic acid from dried T. chebula fruits are effective inhibitors of maltase as acarbose. Research on the bioavailability of dietary tannins is still limited. Binding of tannins to proteins in the gastrointestinal tract may reduce their effective concentration. Nevertheless, tannic acid and the tannin-rich nonalcoholic components of red wine have been found to reduce post-meal blood glucose levels in a study of patients with type 2 diabetes mellitus.[Citation57]
One of the therapeutic approaches for preventing type 2 diabetes is to delay absorption of glucose via inhibition of α-glucosidase activity. The strong enzymatic inhibitory activity against mammalian α-glucosidases shown by T. chebula fruits phenolics could help to control blood glucose level in type 2 diabetes without any critical side effects. The inhibition of α-glucosidase by T. chebula fruits extracts appears to be specific as they did not inhibit other digestive enzymes, such as intestinal sucrase and isomaltase activity, although the inhibitory effect of the fruits of T. chebula on mammalian α-amylase was not tested. Ellagitannins from raspberry (variety ‘Glen Ample’) extract were able to inhibit α-amylase activity in vitro at low concentrations. Further studies are needed to investigate T. chebulafruits phenolics activity against α-amylase and to explore their potential therapeutic effects on post-meal glycemic response by limiting carbohydrate digestion and absorption.
Black/Bitter Cumin (C. anthelminticum)
Cumin is one of the most popular spices used in chicken, beef, or mutton curry preparations in India and Bangladesh. Centratherum anthelminticum (L.) Kuntze is black in color and bitter in taste, which possesses antioxidant and antibacterial activities.[Citation58] Ani et al.[Citation59] tested the effect of phenolics of C. anthelminticum seeds extract (CA) on rat intestinal α-glucosidases, human salivary α-amylase activity, and postprandial glycaemic control in rats. Phenolic compounds of CA showed a dose dependent inhibition of rat intestinal sucrase and maltase with an IC50 value of 34.1 ± 3.8 and 62.2 ± 4.5 μg, respectively. The phenolic compounds of C. anthelminticum seeds extracts were separated by HPLC and their structures were identified by LC-MS. Gallic acid, protocatechuic acid, caffeic acid, ellagic acid, ferulic acid, quercetin, and kaempferol were the major inhibitory components[Citation59] ().
The IC50 value of phenolics of CA was 185.5 ± 4.9 μg for human salivary α-amylase.[Citation59] The inhibitory potency against human salivary α-amylase are expected to be comparable to that of human pancreatic α-amylase because these enzymes share a high degree of amino acid sequence similarity with 97% identical residues overall and 92% in catalytic domains.[Citation60,Citation61]
Certain naturally occurring flavonoids, such as quercetin, kaempferol, luteolin, quercetagetin, and scutellarein act as inhibitors of human α-amylase, which makes them promising candidates for controlling the digestion of starch.[Citation4, Citation45] Flavonoids are integral parts of the human diet providing potential antioxidant benefits for managing diabetes by also preventing the progressive impairment of pancreatic beta-cell function due to oxidative stress.[Citation2, Citation24, Citation62] They cannot be synthesized by the human body, and play beneficial roles in human life.[Citation62,Citation63]
Oral administration of maltose with phenolics of CA resulted in a dose-dependent decrease in the blood glucose level in Wistar rats.[Citation59] Male Wistar albino rats were starved overnight (12 h). When CA phenolics were orally administered at different dosages (0, 50, 100, and 200 mg/kg of body weight) in maltose (2.0 g/kg of body weight), blood glucose levels increased from baseline values of 64.57 ± 1.65, 62.82 ± 4.04, 63.39 ± 3.83, and 64.50 ± 3.94 mg/dl at 0 min to 180.50 ± 3.83, 144.62 ± 6.49, 141.23 ± 5.13, 139.55 ± 2.88 mg/dl at 60 min, respectively (values are mean ± SEM of 5 rats in each group), and then decreased.[Citation59]
Mild inhibition of α-amylase and strong inhibition of intestinal α-glucosidase activity would be an effective strategy for type 2 diabetes management.[Citation64] The IC50 value of CA was lower for α-glucosidase than α-amylase. The inhibitory potency of CA phenolics against α-amylase and α-glucosidase suggested that the potentially safer phenolic compounds from C. anthelminticum seeds extract therefore may be preferred alternatives for controlling postprandial glycemia in type 2 diabetes.
CONCLUSION
The elevated blood glucose level after a meal in type 2 diabetic patients presents a challenge for controlling meal-associated hyperglycemia. Of relevance to the postprandial glycemic control in type 2 diabetes, the nature of the starch in the dietary foods is important. Postprandial glycaemic control in type 2 diabetics due to ingestion of starchy food is of great interest in the context of worldwide health concerns. Type 2 diabetes represents an emerging health burden for governments and health care providers, particularly in the developing world. Although type 2 diabetes and relevant complications are on the increase worldwide, several phenolic compounds have the potential to serve as a remedy against these conditions. Phenolics may provide a protective effect against hyperglycemia-induced chronic diseases through dual protection of antioxidant and inhibition of starch digestion. Co-application of phenolics with synthetic enzyme inhibitors may reduce the effective dose of synthetic inhibitors required for controlling prosprandial glycemia.
Human studies demonstrated the anti-diabetic effects of green tea catechins. Nevertheless, there are several aspects of natural enzyme inhibitors that require further research. For example, the optimal dose of phenolic compounds, including green tea catechins, has not yet been established. In addition, the presence of other dietary components, such as proteins, may reduce the inhibitory potency of polyphenols in the gastrointestinal tract, although some phenolics appear to be enzyme specific. The number of human studies in this field is still very limited. Well-controlled, long-term human studies would help to determine the efficacy, optimal dose, and safety of these inhibitors and ingredient functionality of phenolics in the presence of other dietary components.
Based on the ingredient functionality of phenolic compounds in various food systems and its safer and efficacious dose for humans, it is possible to develop nutraceuticals or functional foods for type 2 diabetic patients. Preserving the native structure of the active molecules during functional food preparation is important. Keeping a balance between α-amylase and α-glucosidase inhibitors would be useful to limit the gastrointestinal adverse effects related to undigested starch reaching the colon. For the development of nutraceuticals or functional foods, knowledge of the interaction between phenolic compounds and digestive enzymes is desirable.
REFERENCES
- WHO (World Health Organization). Diabetes. 2011 http://www.who.int/mediacentre/factsheets/fs312/en/index.html (http://www.who.int/mediacentre/factsheets/fs312/en/index.html) (Accessed: 27 January 2011 ).
- Kwon , Y.-I. , Apostolidis , E. and Shetty , K. 2008 . In vitro studies of eggplant (Solanum melongena) phenolics as inhibitors of key enzymes relevant for type 2 diabetes and hypertension . Bioresource Technology , 99 : 2981 – 2988 .
- Lopez-Candales , A. 2001 . Metabolic syndrome X: A comprehensive review of the pathophisiology and recommended therapy . Journal do Medico , 32 : 283 – 300 .
- Lo Piparo , E. , Scheib , H. , Frei , N. , Williamson , G. , Martin Grigorov , M. and Chou , C. J. 2008 . Flavonoids for controlling starch digestion: Structural requirements for inhibiting human α-amylase . Journal of Medicinal Chemistry , 51 : 3555 – 3561 .
- Koh , L.W. , Wong , L.L. , Loo , Y.Y. , Kasapis , S. and Huang , D. 2010 . Evaluation of different teas against starch digestibility by mammalian glycosidases . Journal of Agricultural and Food Chemistry , 58 : 148 – 154 .
- Shobana , S. , Sreerama , Y.N. and Malleshi , N.G. 2009 . Composition and enzyme inhibitory properties of finger millet (Eleusine coracana L.) seed coat phenolics: Mode of inhibition of α-glucosidase and pancreatic amylase . Food Chemistry , 115 : 1268 – 1273 .
- Brownlee , M. 2005 . The pathobiology of diabetic complications . Diabetes , 54 : 1615 – 1625 .
- Oboh , G. , Ademiluyi , A.O. and Akindahunsi , A.A. 2009 . Changes in polyphenols distribution and antioxidant activity during fermentation of some underutilized legumes . Food Science and Technology International , 15 : 41 – 46 .
- Riccardi , G. , Rivellese , A.A. and Giacco , R. 2008 . Role of glycemic index and glycemic load in the healthy state, in prediabetes, and in diabetes . The American Journal of Clinical Nutrition , 87 ( suppl ) : 269S – 274S .
- Singh , J. , Dartois , A. and Kaur , L. 2010 . Starch digestibility in food matrix: A review . Trends in Food Science & Technology , 21 : 168 – 180 .
- Rhabasa-Lhoret , R. and Chiasson , J.L. 2004 . “ α-Glucosidase inhibitors ” . In International Textbook of Diabetes Mellitus , Edited by: Defronzo , R.A. , Ferrannini , E. , Keen , H. and Zimmet , P. Volume 1 , 901 – 914 . UK : John Wiley & Sons Ltd .
- Obiro , W.C. , Zhang , T. and Jiang , B. 2008 . The nutraceutical role of the Phaseolus vulgaris α-amylase inhibitor . British Journal of Nutrition , 100 : 1 – 12 .
- Park , H. , Hwang , K.Y. , Kim , Y.H. , Oh , K.H. , Lee , J.Y. and Kim , K. 2008 . Discovery and biological evaluation of novel α-glucosidase inhibitors with in vivo antidiabetic effect . Bioorganic & Medicinal Chemistry Letters , 18 : 3711 – 3715 .
- Kodama , T. , Miyazaki , T. , Kitamura , I. , Suzuki , Y. , Namba , Y. , Sakurai , J. , Torikai , Y. and Inoue , S. 2005 . Effects of single and long-term administration of wheat albumin on blood glucose control: Randomized controlled clinical trials . European Journal of Clinical Nutrition , 59 : 384 – 392 .
- Juge , N. and Svensson , B. 2006 . Proteinaceous inhibitors of carbohydrate-active enzymes in cereals: Implication in agriculture, cereal processing and nutrition . Journal of the Science of Food and Agriculture , 86 : 1573 – 1586 .
- Englyst , H.N. , Kingman , S.M. and Cummings , J.H. 1992 . Classification and measurement of nutritionally important starch fractions . European Journal of Clinical Nutrition , 46 ( Suppl 2 ) : S33 – S50 .
- van Amelsvoort , J.M.M. and Weststrate , J.A. 1992 . Amylose-amylopectin ratio in a meal affects postprandial variables in male volunteers . The American Journal of Clinical Nutrition , 55 : 712 – 718 .
- Kabir , M. , Rizkalla , S.W. , Champ , M. , Luo , J. , Boillot , J. , Bruzzo , F. and Slama , G. 1998 . Dietary amylose-amylopectin starch content affects glucose and lipid metabolism in adipocytes of normal and diabetic rats . Journal of Nutrition , 128 : 35 – 43 .
- Berry , C.S. 1986 . Resistant starch: Formation and measurement of starch that survives exhaustive digestion with amylolytic enzymes during the determination of dietary fiber . Journal of Cereal Science , 4 : 301 – 314 .
- Granfeldt , Y. , Drews , A. and Björck , I. 1995 . Arepas made from high amylose corn flour produce favorably low glucose and insulin responses in healthy humans . Journal of Nutrition , 125 : 459 – 465 .
- Thompson , L.U. 1993 . Potential health benefits and problems associated with antinutrients in foods . Food Research International , 26 : 131 – 149 .
- Zajacz , A. , Gyemant , G. , Vittori , N. and Kandra , L. 2007 . Aleppo tannin: Structural analysis and salivary amylase inhibition . Carbohydrate Research , 342 : 717 – 723 .
- Troszynska , A. , Amarowicz , R. , Lamparski , G. , Wolejszo , A. and Barylko-Pikielna , N. 2006 . Investigation of astringency of extracts obtained from selected tannins-rich legume seeds . Food Quality and Preference , 17 : 31 – 35 .
- Song , Y. , Manson , J.E. , Buring , J.E. , Sesso , H.D. and Liu , S. 2005 . Association of dietary flavonoids with risk of type 2 diabetes, and markers of insulin resistance and systemic inflammation in women: A prospective study and cross sectional analysis . Journal of the American College of Nutrition , 24 : 376 – 384 .
- Shrestha , I. and Joshi , N. 1993 . Medicinal plants of the Lele village of Lalitpur district, Nepal . Pharmaceutical Biology , 31 : 130 – 134 .
- Manandhar , N.P. 1995 . A survey of medicinal plants of Jaarkot district, Nepal . Journal of Ethnopharmacology , 48 : 1 – 6 .
- Bhandari , M.R. , Jong-Anurakkun , N. , Hong , G. and Kawabata , J. 2008 . α-Glucosidase and α-amylase inhibitory activities of Nepalese medicinal herb Pakhanbhed (Bergenia ciliata, Haw.) . Food Chemistry , 106 : 247 – 252 .
- He , Q. , Lv , Y. and Yao , K. 2006 . Effects of tea polyphenols on the activities of α-amylase, pepsin, trypsin and lipase . Food Chemistry , 101 : 1178 – 1182 .
- Matsui , T. , Tanaka , T. , Tamura , S. , Toshima , A. , Tamaya , K. , Miyata , Y. , Tanaka , K. and Matsumoto , K. 2007 . α-Glucosidase inhibitory profile of catechins and theaflavins . Journal of Agricultural and Food Chemistry , 55 : 99 – 105 .
- Braca , A. , Politi , M. , Sanogo , R. , Sanou , H. , Morelli , I. , Pizza , C. and De Tommasi , N. 2003 . Chemical composition and antioxidant activity of phenolic compounds from wild and cultivated Sclerocarya birrea (Anacardiaceae) leaves . Journal of Agricultural and Food Chemistry , 51 : 6689 – 6695 .
- Wan , S.B. , Chen , D. , Dou , Q.P. and Chan , T.H. 2004 . Study of the green tea polyphenols catechin-3-gallate (CG) and epicatechin-3-gallate (ECG) as proteaseome inhibitors . Bioorganic & Medicinal Chemistry , 12 : 3521 – 3527 .
- Siebert , K.J. , Troukhanova , N.V. and Lynn , P.Y. 1996 . Nature of polyphenols-protein interactions . Journal of Agricultural and Food Chemistry , 44 : 80 – 85 .
- He , Q. , Shi , B. and Yao , K. 2006 . Interactions of gallotannins with proteins, amino acids, phospholipids and sugars . Food Chemistry , 95 : 250 – 254 .
- Toda , M. , Kawabata , J. and Kasai , T. 2001 . Inhibitory effects of ellagiand gallotannins on rat intestinal α-glucosidase complexes . Bioscience, Biotechnology, and Biochemistry , 65 : 542 – 547 .
- Gao , H. , Huang , Y.-N. , Xu , P.-Y. and Kawabata , J. 2007 . Inhibitory effect on α-glucosidase by the fruits of Terminalia chebula Retz . Food Chemistry , 105 : 628 – 634 .
- Matsuo , T. 1998 . Chemistry, metabolism and biological functions of fruit tannins and relative compounds . Recent Research Developments in Phytochemistry , 2 : 269 – 285 .
- Kawakami , K. , Aketa , S. , Nakanami , M. , Iizuka , S. and Hirayama , M. 2010 . Major water-soluble polyphenols, proanthocyanidins, in leaves of persimmon (Diospyros kaki) and their α-amylase inhibitory activity . Bioscience, Biotechnology, and Biochemistry , 74 : 1380 – 1385 .
- Tachibana , H. 2009 . “ Molecular basis for cancer chemoprevention by green tea polyphenol EGCG ” . In Food Factors for Health Promotion. Forum of Nutrition , Edited by: Yoshikawa , T. 156 – 169 . Basel : Karger .
- Kao , Y.H. , Chang , H.H. , Lee , M.J. and Cheng , C.L. 2006 . Tea, obesity, and diabetes . Molecular Nutrition & Food Research , 50 : 188 – 210 .
- Thielecke , F. and Boschmann , M. 2009 . The potential role of green tea catechins in the prevention of the metabolic syndrome—A review . Phtochemistry , 70 : 11 – 24 .
- Iso , H. , Date , C. , Wakai , K. , Fukui , M. and Tamakoshi , A. 2006 . The relationship between green tea and total caffeine intake and risk for self-reported type-2 diabetes among Japanese adults . Annals of Internal Medicine , 144 : 554 – 562 .
- Hosoda , K. , Wang , M.F. , Liao , M.L. , Chuang , C.K. , Iha , M. , Clevidence , B. and Yamamoto , S. 2003 . Antihyperglycemic effect of oolong tea in type-2 diabetes . Diabetes Care , 26 : 1714 – 1718 .
- Tsuneki , H. , Ishizuka , M. , Terasawa , M. , Wu , J.B. , Sasaoka , T. and Kimura , I. 2004 . Effect of green tea on blood glucose levels and serum proteomic patterns in diabetic (db/db) mice and on glucose metabolism in healthy humans . BMC Pharmacology , 4 : 18
- Grussu , D. , Stewart , D. and McDougall , G.J. 2011 . Berry polyphenols inhibit α-amylase in vitro: Identifying active components in rowanberry and raspberry . Journal of Agricultural and Food Chemistry , 59 : 2324 – 2331 .
- Tadera , K. , Minami , Y. , Takamatsu , K. and Matsuoka , T. 2006 . Inhibition of α-glucosidase and α-amylase by flavonoids . Journal of Nutritional Science and Vitaminology , 52 : 149 – 153 .
- Shim , Y.J. , Doo , H.K. , Ahn , S.Y. , Kim , Y.S. , Seong , J.-K. , Park , I.-S. and Min , B.-H. 2003 . Inhibitory effect of aqueous extract from the gall of Rhus chinensis on alpha-glucosidase activity and postprandial blood glucose . Journal of Ethnopharmacology , 85 : 283 – 287 .
- Wolfe , K.L. , Kang , X. , He , X. , Dong , M. , Zhang , Q. and Liu , R.H. 2008 . Cellular antioxidant activity of common fruits . Journal of Agricultural and Food Chemistry , 56 : 8418 – 8426 .
- Zhang , L. , Li , J. , Hogan , S. , Chung , H. , Welbaum , G.E. and Zhou , K. 2009 . Inhibitory effect of raspberries on starch digestive enzyme and their antioxidant properties and phenolic composition . Food Chemistry , 119 : 592 – 599 .
- McDougall , G.J. , Shpiro , F. , Dobson , P. , Smith , P. , Blake , A. and Stewart , D. 2005 . Different polyphenolic components of soft fruits inhibit α-amylase and α-glucosidase . Journal of Agricultural and Food Chemistry , 53 : 2760 – 2766 .
- Matsui , T. , Ebuchi , S. , Kobayashi , M. , Fukui , K. , Sugita , K. , Terahara , N. and Matsumoto , K. 2002 . Anti-hyperglycemic effect of diacylated anthocyanin derived from Ipomoea batatas cultivar Ayamurasaki can be achieved through the alpha-glucosidase inhibitory action . Journal of Agricultural and Food Chemistry , 50 : 7244 – 7248 .
- Da Silva Pinto , M. , Kwon , Y.I. , Apostolidis , E. , Lajolo , F.M. , Genovese , M.I. and Shetty , K. 2010 . Evaluation of red currants (Ribes rubrum L.), black currants (Ribes nigrum L.), red and green gooseberries (Ribes uva-crispa) for potential management of type 2 diabetes and hypertension using in vitro models . Journal of Food Biochemistry , 34 : 639 – 660 .
- Pinto , M.D. , de Carvalho , J.E. , Lajolo , F.M. , Genovese , M.I. and Shetty , K. 2010 . Evaluation of anti-proliferative, anti-type 2 diabetes, and antihypertension potentials of ellagitannins from strawberries (Fragaria × ananassa Duch.) using in vitro models . Journal of Medicinal Food , 13 : 1027 – 1035 .
- McDougall , G. , Dobson , P. , Shapiro , F. , Smith , P. , Stewart , D. and Fyffe , S. 2007 . Assessing bioavailability of soft fruit polyphenols in vitro . Acta Horticulturae , 744 : 135 – 148 .
- Beekwilder , J. , Hall , R.D. and de Vos , C.H. 2005 . Identification and dietary relevance of antioxidants from raspberry . Biofactors , 23 : 197 – 205 .
- Beekwilder , J. , Jonker , H. , Meesters , P. , Hall , R.D. , van der Meer , I.M. and Ric de Vos , C.H. 2005 . Antioxidants in raspberry: On-line analysis links antioxidant activity to a diversity of individual metabolites . Journal of Agricultural and Food Chemistry , 53 : 3313 – 3320 .
- Kim , K.Y. , Nam , K.A. , Kurihara , H. and Kim , S.M. 2008 . Potent α-glucosidase inhibitors purified from the red alga Grateloupia elliptica . Phytochemistry , 69 : 2820 – 2825 .
- Gin , H. , Rigalleau , V. , Caubet , O. and Aubertin , J. 1999 . Effects of red wine, tannic acid, or ethanol on glucose tolerance in non-insulin dependent diabetic patients and on starch digestibility in vitro . Metabolism , 48 : 1179 – 1183 .
- Ani , V. , Varadarajan , M.C. and Akhilender Naidu , K. 2006 . Antioxidant and antibacterial activities of polyphenolic compounds from bitter cumin (Cuminum nigrum L.) . European Food Research and Technology , 224 : 109 – 115 .
- Ani , V. and Akhilender Naidu , K. 2008 . Antihyperglycemic activity of polyphenolic components of black/bitter cumin Centratherum anthelminticum (L.) . Kuntze seeds. European Food Research and Technology , 226 : 897 – 903 .
- Brayer , G.D. , Luo , Y. and Withers , S.G. 1995 . The structure of human pancreatic α-amylase at 1.8 Å resolution and comparisons with related enzymes . Protein Science , 4 : 1730 – 1742 .
- Ramasubbu , N. , Paloth , V. , Luo , Y. , Brayer , G.D. and Levine , M.J. 1996 . Structure of human salivary alpha-amylase at 1.6 A resolution: Implications for its role in the oral cavity. Acta Crystallographica. Section D . Biological Crystallography , 52 : 435 – 446 .
- Zujko , M.E. and Witkowska , A.M. 2011 . Antioxidant potential and polyphenol content of selected food . International Journal of Food Properties , 14 : 300 – 308 .
- Yildiz , G. , Vatan , O. , Celikler , S. and Dere , S. 2011 . Determination of the phenolic compounds and antioxidative capacity in red algae Gracilaria bursa-pastoris . International Journal of Food Properties , 14 : 496 – 502 .
- Krentz , A.J. and Bailey , C.J. 2005 . Oral antidiabetic agents: Current role in type 2 diabetes mellitus . Drugs , 65 : 385 – 411 .