Abstract
A β-glucosidase from Lycoperdon pyriforme, a wild edible mushroom, was characterized biochemically. The enzyme showed a maximum activity at pH 4.0 and 50°C when p-nitrophenyl-β-D-glucoside was used as a substrate. Km and Vmax values were calculated as 0.81 mM and 1.62 U/mg protein, respectively. The enzyme activity was conserved about 85% over a broad range of pH (3.0–9.0) at 4°C after 24 h incubation. The activity was fully retained after 60 min incubation at 20–40°C. Na+, Li+, Mg2+, Mn2+, Zn2+, Co2+, Ca2+, and Cu2+ did not affect the enzyme activity and 0.25% sodium dodecylsulfate inhibited the enzyme activity approximately 76%. Ethylenediamine tetra-acetic acid, phenylmethanesulfonylfluoride, and dithiothreitol showed no or a little negative effect on the enzyme activity. The resistance of the enzyme to some metal ions, chemicals, and ethanol along with the pH stability, can make it attractive for future applications in industry.
INTRODUCTION
β-Glucosidases (EC 3.2.1.21) occur widely in animals, fungi, bacteria, and plants.[Citation1] They hydrolyze aryl- and alkyl-β-D-glucosides to release glucose and aglycones.[Citation2] β-Glucosidases are widely distributed in the living world and play pivotal roles in many biological processes, such as degradation of cellulosic biomass, hydrolysis of glycolipids, cyanogenesis, and modification of secondary metabolites.[Citation3] In plants, β-glucosidase activity is involved in processes, such as the compartmentalization and activity of phytohormones;[Citation4] defense mechanisms against microbes, insects, or parasitic plants;[Citation5] floral development and pigmentation;[Citation6, Citation7] and they are also thought to have roles in lignification and cell wall decomposition.[Citation8]
β-Glucosidases as part of the cellulase enzyme complex hydrolyze cellobiose and cello-oligosaccharides to yield glucose, which is fermentable by yeasts into fuel ethanol. The conversion of cellulosic biomass into fermentable sugars is a very attractive way of ethanol production.[Citation9] Using β-glucosidases as additives in cellulose-based feeds is beneficial for single-stomach animals, such as pigs and chickens, by enhancing the digestibility of the feed.[Citation10, Citation11] In winemaking, β-glucosidases play a key role in the enzymatic release of aromatic compounds from glycosidic precursors present in fruit juices, musts, and wines. The natural process by endogenous plant β-glucosidases is very time consuming. Supplementation with external enzymes may enhance aroma release.[Citation12] In tea beverages treated with immobilized β-glucosidase, the essential oil content has been increased by 6.79–20.69%.[Citation13] In citrus fruit juices, the hydrolysis of naringenin to prunin reduced the bitterness of the juices.[Citation14]
Isoflavones found in soybean have phytoestrogenic properties, so they can relieve menopausal symptoms and can help prevent several chronic diseases and certain cancers. However, in soy-based foods the isoflavons are mainly in the inactive form of glycosides. Production of aglycons by the hydrolysis via β-glucosidases is highly desired.[Citation15, Citation16] Detoxification of cassava (a staple food in tropical countries) was achieved by the addition of β-glucosidase of Mucor circinelloides. After 24 h of fermentation, all cyanogenic glycosides were hydrolyzed to remove the toxic-CN moiety.[Citation17] There are some examples for the use of the synthetic activity of β-glucosidases in the literature. Arbutin-β-glycosides were synthesized via the transglycosylation reaction of Thermotoga neapolitana β-glucosidase to develop a new skin whitening agent, and the products were evaluated for their melanogenesis inhibitory activities.[Citation18]
The scopes of this study were to extract a β-glucosidase from the fruiting body of Lycoperdon pyriforme and characterize the enzyme to determine optimum reaction conditions (pH and temperature), thermal and pH stability, kinetic parameters, and the effects of various chemical compounds on the activity. Because β-glucosidase has many applications in industry, especially the food processing industry, it is interesting to study β-glucosidase activity from different organisms. There are few studies about the biochemical characterization of β-glucosidases from edible mushroom species. From this point of view, it can be said that the present study is important for its contribution to the literature.
MATERIALS AND METHODS
Materials and Chemicals
Lycoperdon pyriforme Schaeff. was collected from Hidirnebi High Plateau (Trabzon, Turkey), carried into the laboratory in liquid nitrogen, and stored in deep freeze at -34°C. All chemicals used in the study were reagent grade and purchased from Sigma (St. Louis, MO, USA).
Preparation of Crude Enzyme Extract
Crude enzyme extract was prepared as reported previously with some modifications.[Citation19– Citation21] Mushrooms (50 g) were placed in a dewar flask under liquid nitrogen for 10 min in order to decompose cell membranes. The cold mushrooms were homogenized in 100 mL of 50 mM cold phosphate buffer (pH 7.0) containing 2 mM EDTA, 1 mM MgCl2, and 1 mM phenylmethylsulfonyl fluoride (PMSF) by using a porcelain mortar. After the homogenate was filtered through four layers of muslin, the filtrate was centrifuged at 20,000 rpm for 30 min at 4°C. The supernatant was used as crude enzyme extract.
Acetone Precipitation of L. pyriforme β-Glucosidase
The equal volume of cold acetone (−30°C) was added to the crude enzyme extract and the mixture was incubated at 4°C for 2 h for the precipitation of proteins. After centrifugation at 20000 rpm for 10 min at 4°C, the precipitate was re-dissolved in 5 mL of 50 mM phosphate buffer (pH 7.0).
Determination of Protein Concentration
Protein concentration was determined according to the Lowry method[Citation22, Citation23] with bovine serum albumin as a standard. The values were obtained by graphic interpolation on a calibration curve at 650 nm.
Determination of β-Glucosidase Activity
β-Glucosidase activity was assayed by using p-nitrophenyl-β-D-glucoside (pNPG) as a substrate. Then 200 μL of enzyme solution and 200 μL of pNPG (4 mM stock) were incubated for 15 min at 37°C. The reaction was stopped by the addition of 1.2 mL Na2CO3 (0.1 M). The amount of p-nitrophenol (pNP) liberated was estimated at 410 nm using a Perkin Elmer Lambda 25 (Perkin Elmer, UK) as spectrophotometer. One unit of enzyme activity was defined as the amount of enzyme producing 1 nmole of p-nitrophenol per minute under the assay conditions.[Citation21, Citation24]
Activity Staining
Non-denaturing polyacrylamide gel electrophoresis was performed at 4°C by using a 10% separating gel containing 4-methylumbelliferyl-β-D-glucopyranoside (MUG) in the final concentration of 0.02% (w/v). After the electrophoresis was run, the gel was washed three times with 50 mM acetate buffer (pH 5.0) and incubated at 37°C for 30 min in 50 mM acetate buffer (pH 5.0) containing 0.1% MUG. β-Glucosidase bands were then visualized under UV light.[Citation25]
Effect of pH and Temperature on L. pyriforme β-Glucosidase Activity
The activity of L. pyriforme β-glucosidase as a function of pH was assayed at 37°C by using pNPG as a substrate and 50 mM buffer systems: Glycine-HCl (pH 3.0), acetate (pH 4.0–5.0), phosphate (pH 6.0–8.0), and Tris-HCl (pH 9.0). The activity was expressed as percent relative activity with respect to maximum activity, which was considered as 100%.[Citation26] The optimum temperature of the enzyme was determined at optimum pH value by measuring the activity at different temperatures in the range of 20–80°C with 10°C increments by using pNPG as a substrate. The activity was expressed as percent relative activity in relation to the temperature optimum, which was considered as 100%.[Citation25]
Enzyme Kinetics
Enzyme kinetic parameters of L. pyriforme β-glucosidase were obtained by measuring the rate of pNPG hydrolysis at various substrate concentrations ranging from 10 to 1000 μM in the standard reaction mixture (at 50°C in 50 mM acetate buffer, pH 4.0). The Michaelis-Menten constant (K m) and maximum velocity (V max) values were determined from the Lineweaver-Burk plot using Microsoft Excel software (Microsoft Corporation, USA).
pH and Thermal Stability of the Enzyme
The effect of pH on the enzyme stability was determined by incubating the partially purified enzyme at 4 and 25°C for 24 h in the buffer solutions of different pH values: Glycine-HCl (pH 3.0), acetate (pH 4.0–5.0), phosphate (pH 6.0–8.0), and Tris-HCl (pH 9.0). At the end of the storage period, the β-glucosidase activity was assayed under standard reaction conditions. The percentage of residual enzyme activity was calculated by comparison with a non-incubated enzyme.[Citation25, Citation27] In order to determine the thermal stability of the L. pyriforme β-glucosidase, the enzyme solutions in Eppendorf tubes were incubated at temperatures over the range of 20–80°C with 10°C increments. Aliquots were withdrawn at times of 15, 30, 45, and 60 min, rapidly cooled in an ice bath for 5 min, and then brought to 25°C. After reaching room temperature, the enzyme activity was determined at standard assay conditions. Control with non-incubated enzyme was used to determine the 100% activity value.[Citation26– Citation28]
Effect of Some Metal Ions and Chemicals on the Enzyme Activity
The effect of metal ions on the enzyme activity was separately investigated by adding chloride salts of Na+, Li+, Mg2+, Mn2+, Zn2+, Co2+, Ca2+, and Cu2+ directly to the standard reaction mixture in a final concentration of 1 and 5 mM. Enzyme activity determined in the absence of metal ion was defined as 100%.[Citation26] To study the effect of some chemicals on the enzyme activity, ethylenediamine tetra-acetic acid (EDTA), phenylmethanesulfonylfluoride (PMSF), and dithiothreitol (DTT) were separately added to the standard reaction mixture in the final concentration of 10 and 25 mM. In addition, the effect of sodium dodecylsulfate (SDS) on the enzyme activity was investigated in the final concentration of 0.25 and 0.75%. The percentage of residual activities were expressed by comparison with a standard assay mixture with no chemical added.[Citation26]
Effect of Sugars and Ethanol on β-Glucosidase Activity
Effects of sugar (glucose, fructose, and sucrose) on enzyme activities were conducted by using sugar concentrations over a range of 0–20% w/v. Stock solutions of glucose, fructose, and sucrose were prepared in a 50-mM acetate buffer (pH 4.0) and were added to the reaction mixture resulting in final concentrations from 0 to 20% (w/v).[Citation29] The enzyme assay was carried out by standard assay conditions as previously described. Effect of ethanol on enzyme activity was conducted by using ethanol concentration over a range of 0–20% v/v. Ethanol was added to the reaction mixture resulting in final concentrations from 0 to 20% (v/v).[Citation29] The enzyme assay was carried out by standard assay conditions as previously described.
RESULTS AND DISCUSSION
In this study, a β-glucosidase from the fruiting body of L. pyriforme was partially purified 10.0-fold with acetone precipitation. It was previously reported that a β-glucosidase from the fruiting body of Lepista flaccida was partially purified 5.0-fold with 30–40% ammonium sulfate saturation.[Citation30]
Activity Staining
Non-denaturing polyacrylamide gel electrophoresis was performed at 4°C by using a 10% separating gel containing MUG in the final concentration of 0.02% (w/v). After the electrophoresis, β-glucosidase bands were visualized under UV light. While the presence of two β-glucosidase bands was observed in the crude enzyme extract, one activity band was seen in the enzyme solution obtained with acetone precipitation (). Two activity bands observed in the crude enzyme extract could be attributed to the presence of isoenzymes. The existence of isoenzymes for β-glucosidases has been previously reported for other organisms, such as oat seeds and Lepista flaccida.[Citation30, Citation31]
Effect of pH and Temperature on L. pyriforme β-Glucosidase Activity
β-Glucosidase activity of the L. pyriforme was analyzed at 37°C between pH values of 3.0 and 9.0 using 50 mM buffer systems. Maximum activity was measured at pH 4.0 (). The enzyme showed approximately 55, 90, and 80% of its maximum activity at pH 3.0, 8.0, and 9.0, respectively. In addition, it was almost fully inhibited at pH 8.0 and 9.0. Similar optimum pH results were also reported for Stachybotrys strain, Aspergillus niger strain 322, and Metschnikowia pulcherrima.[Citation32– Citation34] The trend for higher specific activity at pH values lower than the optimum of 4.0 reported for most of the β-glucosidases might be due to an acid-base catalytic mechanism.[Citation35] Activity of the L. pyriforme β-glucosidase was investigated over a temperature range from 20 to 80°C at pH 4.0. The temperature optimum for the enzyme was 50°C and at least 90% activity was observed between 40 and 60°C (). It was previously reported that Volvariella volvacea, Stachybotrys strain, A. niger strain 322, and Achatina fulica β-glucosidases had an optimum temperature of 50°C.[Citation25, Citation32, Citation33, Citation36]
Figure 3 Temperature-activity profile of L. pyriforme β-glucosidase. The background hydrolysis of the substrate was deducted by using a reference sample of identical composition to the incubation mixture except for the enzyme. The activity was expressed as percent relative activity in relation to the temperature optimum, which was considered as 100%.
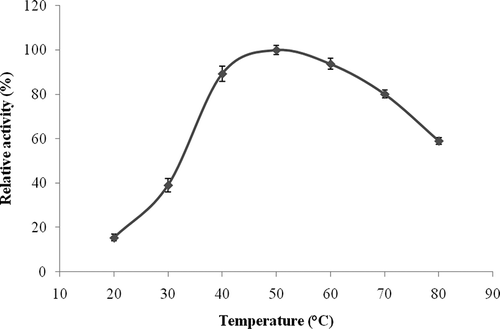
Enzyme Kinetics
Enzyme kinetic parameters of L. pyriforme β-glucosidase were obtained by measuring the rate of pNPG hydrolysis at various substrate concentrations ranging from 0.01 to 2 mM in the standard reaction mixture. The values of K m and V max were calculated from the Lineweaver-Burk plot as 0.81 mM and 1.62 U/mg protein, respectively (). K m values of β-glucosidases from other organisms for pNPG range from 0.2 to 21.7 mM.[Citation37] It was also reported that K m values of L. flaccida, M. pulcherrima, Pichia pastoris, Stachybotrys strain, and A. fulica β-glucosidases were 1.06, 1.5, 0.12, 0.3, and 0.224 mM, respectively.[Citation25, Citation30, Citation32, Citation34, Citation38] Thus, L. pyriforme β-glucosidase had a comparable K m value for pNPG. K m is a measure of the affinity of an enzyme for a particular substrate and depends on environmental conditions, such as pH, temperature, and ionic strength.[Citation39] For this reason, the K m values of β-glucosidases from different organisms could be different.
pH and Thermal Stability of the Enzyme
pH stability of L. pyriforme β-glucosidase was determined by incubating the enzyme for 24 h at 4 and 25°C in the buffers having different pH values between 3.0 and 9.0. After incubation, the activity was assayed under standard reaction conditions. The enzyme was quite stable at all pH values and retained over 85% of its original activity at 4°C after 24 h incubation (). It was reported that L. flaccida β-glucosidase retained over 90% of its original activity at pH 4.0 and 5.0 after 24 h incubation at 4°C. It was also quite stable at pH 2.0–3.0 and 6.0–9.0.[Citation30] The activity of L. pyriforme β-glucosidase was fully retained at 25°C after 24 h incubation (). It was reported that β-glucosidase from Periconia sp. was highly stable at basic pH, but was unstable at acidic pH after 4 h at 25°C. It retained more than 85% of its maximal activity after being incubated at pH 7–10.[Citation40]
Thermal stability tests showed that L. pyriforme β-glucosidase retained its full activity between 20 and 40°C after 1 h, but it fully lost its original activity at 50–80 °C (). L. pyriforme β-glucosidase conserved approximately 75 ± 2.5% and 70 ± 3.8% of its original activity after 15 min at 60 and 70°C, respectively. It was reported that β-glucosidase from V. volvacea lost 70% of its original activity after only 5 min incubation at 60°C.[Citation41] Activity of A. oryzae β-glucosidase significantly decreased at temperatures above 40°C when the enzyme was incubated for 4 h.[Citation42] It was also previously reported that storage of A. niger strain 322 β-glucosidase for 30 min at temperatures higher than 50°C resulted in a gradual loss in relative activity, reaching as low as 9–8% at 80°C.[Citation33] When L. flaccida β-glucosidase was incubated at temperatures between 10 and 40°C for 30 min, it retained almost 80% of its original activity, but it lost 35 and 37% of its original activity at 50 and 60°C, respectively.[Citation30]
Table 1 Effect of some metal ions on the β-glucosidase activity of L. pyriforme. Enzyme activity determined in the absence of metal ion was defined as 100%
Effect of Some Metal Ions and Chemicals on the Enzyme Activity
The effect of Na+, Li+, Mg2+, Mn2+, Zn2+, Co2+, Ca2+, and Cu2+ on the β-glucosidase activity of L. pyriforme was tested at 1 and 5 mM concentration of each metal ion. All had no or a little negative/positive effect on the enzyme activity, demonstrating that the enzyme did not require these metal ions to function (). It was reported that β-glucosidase activity of M. pulcherrima was not affected by 10 mM NaCl.[Citation34] Also, it was reported that 1 mM of Mn2+, Co2+, Ca2+, and Cu2+ did not significantly affect the activity of a soybean β-glucosidase.[Citation43] β-Glucosidase activity of V. volvacea was unaffected by 1 mM of Mn2+, Mg2+, and Co2+.[Citation36] To study the effect of EDTA, PMSF, and DTT on the enzyme activity, they were separately added to the standard reaction in the final concentration of 10 and 25 mM. They had no effect on the β-glucosidase activity of L. pyriforme. SDS inhibited the enzyme 76 and 100% in the final concentration of 0.25 and 0.75%, respectively (). It was reported that β-glucosidase activity of Volvariella volvacea was completely inhibited by 0.1% SDS (w/v).[Citation36] The inhibition of the enzyme by SDS indicates that the integrity of its three-dimensional structure is critical for its catalytic activity. Since EDTA had no effect on the activity, it appears that the enzyme does not depend on a metallic co-factor.
Table 2 Effect of some chemicals on the β-glucosidase activity of L. flaccida. The percentage residual activities were expressed by comparison with standard assay mixture with no metal ion or chemicals added
Effect of Sugars on β-Glucosidase Activity
Effects of glucose, fructose, and sucrose on the enzyme activity were measured by using sugar concentrations over a range of 0–20% w/v. The results are shown in . An amount of 2.5% (w/v) glucose inhibited the β-glucosidase approximately 75%. In the presence of 20% (w/v) glucose, the enzyme activity was strongly inhibited. Whereas L. pyriforme β-glucosidase conserved approximately all of its original activity in the presence of 2.5 and 5.0% fructose, it was inhibited about 15% in higher concentrations. L. pyriforme β-glucosidase did not become inhibited until the sucrose concentration reached 15% (w/v). The enzyme activity was inhibited approximately 30% by 30% (w/v) sucrose. These results are consistent with the literature. It was reported that β-glucosidase of Pichia anomala was strongly inhibited by 20% (w/v) glucose and was slightly inhibited by fructose and sucrose.[Citation29] Commercial β-glucosidase enzymes from fungi, such as Aspergillus niger, were also inhibited by glucose. In addition, β-glucosidases from Hanseniaspora uvarum and A. niger was slightly inhibited by fructose.[Citation44] But, an intracellular β-glucosidase from Debaryomyces hansenii was highly tolerant to glucose, and only 20% inhibited in 500 mM glucose.[Citation45]
Effect of Ethanol on β-Glucosidase Activity
Effect of ethanol on enzyme activity was determined by using ethanol concentration over a range of 0–20% (v/v) (). β-Glucosidase activity was almost fully conserved at the concentrations of 5 and 10% (v/v) ethanol. It can be said that the enzyme was tolerant to ethanol, because its activity was only slightly reduced by 10% in the presence of 15% (v/v) ethanol. These results imply that β-glucosidase from L. pyriforme would be useful if directly added during the final stages of alcoholic fermentation (ethanol concentration <15%).[Citation29] It was reported that β-glucosidase from D. hansenii was tolerant to ethanol.[Citation45] L. pyriforme β-glucosidase was inhibited about 40% in the presence of 20% (v/v) ethanol. The inhibitory effect of alcohol at this concentration is probably due to effects in the enzyme structure brought on by changes in the polarity of the medium.[Citation39] It was earlier reported that β-glucosidase from Hanseniaspora sp. was substantially inhibited in the presence of ethanol.[Citation29]
CONCLUSION
In conclusion, the present study has revealed the isolation and characterization of a β-glucosidase for the first time from L. pyriforme. It can be concluded from the results that the resistance of L. pyriforme β-glucosidase to some metal ions, chemicals, and ethanol along with the pH stability, can make the enzyme useful for future application in industry as well as in biotechnology.
ACKNOWLEDGMENTS
The author wishes to thank KTU-BAP for financial support, and also Dr. Nagihan Saglam Ertunga and Dr. Ahmet Colak for their proofreading and technical support.
REFERENCES
- Woodward , J. and Wiseman . 1982 . A. Fungal and other β-glucosidases . Their properties and applications. Enzyme and Microbial Technology , 4 : 73 – 79 .
- Reese , E.T. 1977 . Degradation of polymeric carbohydrates by microbial enzymes . Recent Advances in Phytochemistry , 11 : 311 – 364 .
- Esen , A. 1992 . Purification and partial characterization of maize (Zea mays L.) β-glucosidase . Plant Physiology , 98 : 174 – 182 .
- Kleczkowski , K. and Schell , J. 1995 . Phytohormone conjugates: Nature and function . Critical Reviews in Plant Sciences , 14 : 283 – 298 .
- Phillips , D.A. and Streit , W. 1996 . “ Legume signals to rhizobial symbionts: A new approach for defining rhizosphere colonization ” . In Plant-Microbe Interactions , Edited by: Stacey , G. and Keen , N. 236 – 271 . New York : Chapman & Hall . InEds.
- Harborne , J.B. and Mabry , T.J. 1982 . The Flavonoids: Advances in Research , London : Chapman and Hall .
- Koes , R.E. , Quattrocchino , F. and Mol , J.N.M. 1994 . The flavonoid biosynthetic pathway in plants: Function and evolution . BioEssays , 16 : 123 – 132 .
- Leah , R. , Kigel , J. , Svendsen , I. and Mundy . 1995 . J. Biochemical and molecular characterization of a barley seed β-glucosidase . The Journal of Biological Chemistry , 270 : 15789 – 15797 .
- Krisch , J. , Tako , M. , Papp , T. and Vagvölgyi , C. 2010 . “ Characteristics and potential use of β-glucosidases from Zygomycetes ” . In Current Research, Technology and Education Topics in Applied Microbiology and Microbial Biotechnology Edited by: Mendez-Vilas , A. InEd. Formatex: Badajaz, Spain
- Zhang , Z. , Marquardt , R.R. , Wang , G. , Guenter , W. , Crow , G.H. , Han , Z. and Bedford , M.R. 1996 . A simple model for predicting the response of chicks to dietary enzyme supplementation . Journal of Animal Sciences , 74 : 394 – 402 .
- Coenen , T.M. , Schoenmakers , A.E. and Verhagen , H. 1995 . Safety evaluation of β-glucanase derived from Trichoderma reesei: Summary of toxicological data. Food Chemistry and Toxicology , 33 : 859 – 866 .
- Gueguen , Y. , Chemardin , S.P. , Arnaud , A. and Galzy , P. 1998 . “ Investigation of β-glucosidases potentialities of yeast strains and application to bound aromatic terpenols liberation ” . In New Frontiers in Screening for Microbial Biocatalysts , Edited by: Kieslich , K. , van der Beek , C.P. , de Bont , J.A.M. and van den Tweel , W.J.J. 149 – 157 . Amsterdam : Elsevier . InEds
- Sub , E. , Xiaa , T. , Gaoa , L. , Daia , O. and Zhanga , Z. 2010 . Immobilization of β-glucosidase and its aroma-increasing effect on tea beverage . Food and Bioproducts Processing , 88 : 83 – 89 .
- Roitner , M. , Schalkhammer , T. and Pittner , F. 1982 . Characterization of naringinase from Aspergillus niger . Monatshefte für Chemie–Chemical Monthly , 115 : 1255 – 1267 .
- Hu , S.C. , Hong , K. , Song , Y.C. , Liu , J.Y. and Tan , R.X. 2009 . Biotransformation of soybean isoflavones by a marine Streptomyces sp. 060524 and cytotoxicity of the products . World Journal of Microbiology and Biotechnology , 25 : 115 – 121 .
- Pandjaitan , N. , Hettiarachchy , N. and Ju , Z.Y. 2000 . Enrichment of genistein in soy protein concentrate with β-glucosidase . Journal of Food Science , 65 : 403 – 407 .
- Petruccioli , M. , Brimer , L. , Cicalini , A.R. and Federici , F. 1996 . The linamarase of Mucor circinelloides LU M40 and its detoxifying activity on cassava . Journal of Applied Microbiology , 86 : 302 – 310 .
- Jun , S.-Y. , Park , K.-M. , Choi , K.-W. , Kyung Jang , M. , Kang , H.Y. , Lee , S.H. , Park , K.H. and Cha , J. 2008 . Inhibitory effects of arbutin-β-glycosides synthesized from enzymatic transglycosylation for melanogenesis . Biotechnology Letters , 30 : 743 – 748 .
- Colak , A. , Sahin , E. , Yildirim , M. and Sesli , E. 2007 . Polyphenol oxidase potentials of three wild edible mushroom species harvested from Lişer High Plateau, Trabzon . Food Chemistry , 103 : 1426 – 1433 .
- Özel , A. , Colak , A. , Arslan , O. and Yildirim , M. 2010 . Purification and characterisation of a polyphenol oxidase from Boletus erythropus and investigation of its catalytic efficiency in selected organic solvents . Food Chemistry , 119 : 1044 – 1049 .
- Elvan , H. , Saglam Ertunga , N. , Yildirim , M. and Colak , A. 2010 . Partial purification and characterization of endoglucanase from an edible mushroom, Lepista flaccida . Food Chemistry , 123 : 291 – 295 .
- Lowry , O.H. , Rosebrough , N.J. , Farr , A.L. and Randall , R.J. 1951 . Protein measurement with the folin phenol reagent . The Journal of Biological Chemistry , 19 : 265 – 275 .
- Nagaia , T. , Inoueb , R. , Suzukic , N. and Nagashimaa , T. 2009 . Alpha-amylase from persimmon honey: Purification and characterization . International Journal of Food Properties , 12 : 512 – 521 .
- Günata , Z. and Vallier , M.-J. 1999 . Production of a highly glucose-tolerant extracellular β-glucosidase by three Aspergillus strains . Biotechnology Letters , 21 : 219 – 223 .
- Hu , Y. , Luan , H. , Hao , D. , Xiao , H. , Yang , S. and Yang , L. 2007 . Purification and characterization of a novel ginsenoside-hydrolyzing β-D-glucosidase from the China white jade snail (Achatina fulica). Enzyme and Microbial Technology , 40 : 1358 – 1366 .
- Yildirim , M. , Colak , A. , Col , M. and Canakci , S. 2009 . A new recombinant phosphotriesterase homology protein from Geobacillus caldoxylosilyticus TK4: An extremely thermo-pH-stable esterase . Process Biochemistry , 44 : 1366 – 1373 .
- Yildirim , M. , Col , M. , Colak , A. , Güner , S. , Dülger , S. and Beldüz , A.O. 2005 . Diphenolases from Anoxybacillus kestanbolensis strains K1 and K4T . World Journal of Microbiology and Biotechnology , 21 : 501 – 507 .
- Tuncay , D. and Yagar , H. 2011 . Comparison of polyphenol oxidases prepared from different parts of artichoke (Cynara scolymus L.) . International Journal of Food Properties , 14 : 809 – 821 .
- Swangkeaw , J. , Vichitphan , S. , Butzke , C.E. and Vichitphan , K. 2011 . Characterization of β-glucosidases from Hanseniaspora sp. and Pichia anomala with potentially aroma-enhancing capabilities in juice and wine . World Journal of Microbiology and Biotechnology , 27 : 423 – 430 .
- Elvan , H. , Saglam Ertunga , N. , Colak , A. and Yildirim , M. 2011 . β-Glucosidase from an edible mushroom, Lepista flaccida: Partial purification and characterization . Asian Journal of Chemistry , 23 : 1107 – 1111 .
- Kim , Y.-W. and Kim , I.-S. 1998 . Subunit composition and oligomer stability of oat β-glucosidase isozymes. Biochimica et Biophysica Acta (BBA)—Protein Structure and Molecular Enzymology , 1388 : 457 – 464 .
- Amouri , B. and Gargouri , A. 2006 . Characterization of a novel β-glucosidase from a Stachybotrys strain . Biochemical Engineering Journal , 32 : 191 – 197 .
- Peshin , A. and Mathur , J.M.S. 1999 . Purification and characterization of β-glucosidase from Aspergillus niger strain 32 . Letters in Applied Microbiology , 28 : 401 – 404 .
- Gonzalez-Pombo , P. , Perez , G. , Carrau , F. , Guisan , J.M. , Batista-Viera , F. and Brena , B.M. 2008 . One-step purification and characterization of an intracellular β-glucosidase from Metschnikowia pulcherrima . Biotechnology Letters , 30 : 1469 – 1475 .
- Legler , G. 1990 . Glycoside hydrolases: Mechanistic information from studies with reversible and irreversible inhibitors . Advances in Carbohydrate Chemistry & Biochemistry , 48 : 319 – 323 .
- Li , X. , Pei , J. , Wu , G. and Shao , W. Expression . 2005 . purification and characterization of a recombinant β-glucosidase from Volvariella volvacea . Biotechnology Letters , 27 : 1369 – 1373 .
- Joo , A.-R. , Jeya , M. , Lee , K.-M. , Sim , W.-I. , Kim , J.-S. , Kim , I.-W. , Kim , Y.-S. , Oh , D.-K. , Gunasekaran , P. and Lee , J.-K. 2009 . Purification and characterization of a β-1, 4-glucosidase from a newly isolated strain of Fomitopsis pinicola . Applied Microbiology and Biotechnology , 83 : 285 – 294 .
- Turan , Y. and Zheng , M. 2005 . Purification and characterization of an intracellular β-glucosidase from the methylotrophic yeast Pichia pastoris . Biochemistry (Moskow) , 70 : 1363 – 1368 .
- Berg , J.M. , Tymoczko , J.L. and Stryer , L. 2002 . “ The Michaelis-Menten model accounts for the kinetic properties of many enzymes ” . In Biochemistry Edited by: Freeman , W. H. New York In
- Harnpicharnchai , P. , Champreda , V. , Sornlake , W. and Eurwilaichitr , L. 2009 . A thermotolerant β-glucosidase isolated from an endophytic fungi, Periconia sp., with a possible use for biomass conversion to sugars . Protein Expression and Purification , 67 : 61 – 69 .
- Cai , Y.J. , Buswell , J.A. and Chang , S.T. 1998 . β-Glucosidase components of the cellulolytic system of the edible straw mushroom, Volvariella volvacea . Enzyme and Microbial Technology , 22 : 122 – 129 .
- Horii , K. , Adachi , T. , Matsuda , T. , Tanaka , T. , Sahara , H. , Shibasaki , S. , Ogino , C. , Hata , Y. , Uedaf , M. and Kondo , A. 2009 . Improvement of isoflavone aglycones production using β-glucosidase secretory produced in recombinant Aspergillus oryzae . Journal of Molecular Catalysis B: Enzymatic , 59 : 297 – 301 .
- Hsieh , M.-C. and Graham , T.L. 2001 . Partial purification and characterization of a soybean β-glucosidase with high specific activity towards isoflavone conjugates . Phytochemistry , 58 : 995 – 1005 .
- Barbagallo , R.N. , Spagna , G. , Palmeri , R. , Restuccia , C. and Giudici , P. Selection . 2004 . characterization and comparison of β-glucosidase from mould and yeasts employable for enological applications . Enzyme and Microbial Technology , 35 : 58 – 66 .
- Yanai , T. and Sato , M. 1999 . Isolation and properties of β-glucosidase produced by Debaryomyces hansenii and its application in winemaking . American Journal of Enology and Viticulture , 50 : 231 – 235 .