Abstract
Protein fractions from Silybum marianum seeds were sequentially extracted based on solubility and then compared with protein isolate in terms of the yields and physicochemical properties. Molecular weights of protein fractions were in the range of 16.3–112.1 kDa. Secondary structure of the protein fractions had a high amount of β-types. Albumin fraction was the dominant seed protein with a characteristic profile of tryptophan residues in most hydrophilic environments. Solubility of protein fractions was lowest at pH 4–5. Besides high levels of essential amino acids favorably compared with recommended levels for pre-school children, S. marianum seed proteins could also be used as protein extenders and acid formulations.
INTRODUCTION
Milk thistle Silybum marianum L. Gaernt (family Compositae) is an annual or biennial plant, native to the Mediterranean area, which has now spread to other warmer and dryer regions. Various preparations of the plant, especially the fruits, have been medicinally used to treat liver disorders for over 2000 years.[Citation1] Besides this cytoprotective activity, there is a growing interest in its anticancer and chemopreventive activity as well as hypocholesterolemic, cardioprotective, neuroactive, and neuroprotective activities.[Citation2] Silymarin is the pharmacological active component of the fruit, which is composed of an isomeric mixture of the flavonolignans silychristin, silydianin, and the diastereoisomers silybin and isosilybin.[Citation3] Thus, S. marianum is also a crop for silymarin industrial production. Recently, the increased consumption of silymarin has finally made farmers expand the harvest area of S. marianum. In China, the growing area in Qinghai, Shanxi, Hubei, Jiangsu, and Guangdong provinces has increased year by year.[Citation4]
The seeds of S. marianum also contain a relatively high amount of oil and crude proteins (approximately 19.58%, dry weights of the seeds).[Citation5] This oil contains phospholipids, relatively high amounts of vitamin E,[Citation6] and a great quantity of unsaturated fatty acids, such as linoleic acid (C18:2) and oleic acid (C18:1). For plant proteins to be useful and successful in food application, they should ideally possess several desirable characteristics, referred to as functional properties, as well as providing essential amino acids.[Citation7] These properties are intrinsic physicochemical characteristics that affect the behavior of proteins in food systems during processing, manufacturing, storage, and preparation.[Citation7] Therefore, it is necessary to investigate these properties to utilize the dietary protein from the seeds in the food industry. However, there is little information on the properties of protein fractions of the S. marianum seeds. Thus, the present study was to evaluate some basic physicochemical properties of protein fractions from S. marianum seeds; the protein isolate of S. marianum seeds was also used as a comparison. These properties include molecular weights of major fractions, amino acid compositions, secondary and tertiary conformations, and protein solubility. It was also of interest to determine some functional properties of the seed proteins, as it is essential for their potential use as protein extenders and as acid formulations.
MATERIALS AND METHODS
Materials
S. marianum seeds were obtained from Zhongxing Yaoyue Co., Ltd. (Zhenjiang, Jiangsu, China), and identified by Chen Li (Chief Pharmacist, Food and Drug Administration Bureau of Zhenjiang). Low molecular weight protein markers (Code number: D530S) were purchased from DINGUO Biotech. Co., Ltd. (Shanghai, China). All solvents and chemicals were of analytical reagent grade or better grade and obtained from Sinopharm Chemical Reagent Co., Ltd. (Shanghai, China).
Proximate Analysis
The recommended methods of the Association of Official Analytical Chemists[Citation8] were adopted to determine the content of crude protein, moisture, and total ash of S. marianum seed powders (SSP). The crude fiber content was determined by the ceramic fiber filter method. The carbohydrate contents of the samples were determined as nitrogen free extract (NFE) by the following formula: NFE % = 100 − (moisture + crude fat + crude protein + crude fiber + total ash).
Preparation of Defatted Powders of S. marianum Seed (DSSP)
Seeds were removed from the S. marianum fruit, husked, and air-dried at 25°C, and then crashed to the average particle size of 300 μm. SSP were defatted with hexane at 25°C, air-dried in the fume hood, and stored in bottle desiccators at room temperature.
Preparation of Protein Fractions and Protein Isolate
Protein fractions of DSSP were fractionated according to the Osborne methods[Citation9] with modifications as follows. For albumin fraction (SA), suspensions of DSSP/de-ionized water (1:10, w/v) were stirred for 1 h at 25°C and centrifuged at 13,500 g for 15 min. After dialyzing extensively with de-ionized water for 24 h, the supernatant was centrifuged again, and lyophilized to obtain the SA. To obtain the globulin fraction (SG), the precipitate obtained by the first centrifugation was re-suspended in a solution of 0.5 mol/L NaCl (1:5, w/v), and the resulting suspension stirred for 1 h at 25°C. After centrifugation under the same conditions as described earlier, the supernatant was dialyzed and centrifuged again. The corresponding precipitate was dissolved in de-ionized water and then lyophilized to obtain the SG. The solid residue after SG extraction was used for the sequential extraction of prolamin fraction (SP) and glutelin fraction (SGL) by using 75% ethanol solution and 0.05 mol/L NaOH stirred for 1 h at 25°C, respectively. Ethanol in the SP was removed by evaporate at 45°C. For SGL, the extract was adjusted to pH 4.5 with 0.5 mol/L HCl, and then centrifuged at 13,500 g for 15 min. The precipitate was then re-suspended in five volumes of de-ionized water and adjusted to pH 7.0 with 0.5 mol/L NaOH. After centrifugation under the same conditions, the supernatant was dialyzed with de-ionized water for 24 h then lyophilized to produce SGL. The molecular weight (MW) cut-off of dialysis membrane was above 3500 Da.
Protein isolate from S. marianum seeds (SPI) was prepared from the DSSP according to the method described by Tang[Citation10] with necessary modifications. Briefly, the powders were fully dispersed in a 10-fold volume of de-ionized water for 2 h at room temperature, and then the dispersion was adjusted to pH 8.0 with with NaOH (0.1 mol/L). After centrifugation at 13,500 g for 15 min, the resultant supernatant was adjusted to pH 4.5 using HCl (0.5 mol/L) to precipitate the proteins. The precipitate was re-dispersed in de-ionized water, then dialyzed and lyophilized to obtain the SPI.
Quantification of Total, Free, and Protein-Bound Polyphenols
The contents of total, free, and protein-bound polyphenols were determined according to the method described by Tang et al.[Citation11] with slight modifications. The free polyphenols content was determined by the absorbance of the supernatant after protein precipitation with 8% trichloroacetic acid and centrifugation. All other steps and calculations were done exactly as described in the original method. Rutin was used as a standard compound for the standard curve. The contents of the polyphenols were expressed as g rutin equivalent/100 g samples.
Amino Acid Analysis
The amino acid compositions of the samples were determined by an L-8800 automatic amino acid analyzer (Hitachi, Tokyo, Japan). The samples were hydrolyzed by using 6 mol/L HCl at 110°C in a sealed tube for 24 h. The amino acid compositions were expressed in proportion of the single amino acid to total amino acids. The amino acid tryptophan was not determined.
Sodium Dodecyl Sulfate-Polyacrylamide Gel Electrophoresis (SDS-PAGE)
SDS-PAGE was performed on a discontinuous buffered system, according to the method described by Laemmli,[Citation12] using 10% separating gel and 6% stacking gel with slight modifications. The samples were dissolved in 0.06 mol/L Tris-HCl buffer (pH 6.8), containing 2% (w/v) SDS, 2.5% (v/v) 2-mercaptoethanol (2-ME), 25% (v/v) glycerol, and 0.1% (w/v) bromophenol blue, and then heated for 5 min in boiling water before electrophoresis. An aliquot of each sample (15 μL) was applied to each lane to the electrophoresis, after that the gel was stained in 0.1% Coomassie blue (R-250) and 10% acetic acid (methanol:acetic acid:water, 9:2:9, v/v/v) and de-stained in 10% acetic acid (methanol:acetic acid:water, 1:1:8, v/v/v). The molecular weights of protein subunits for each sample were determined using low molecular weight protein markers.
Circular Dichroism (CD) Spectroscopy
CD spectra were recorded at 25°C on a MOS-450 spectropolarimeter (BioLogic Science Instruments, Claix, France). The CD spectroscopy measurements were performed in quartz cuvette of 2 mm with an approximate protein concentration of about 0.1 mg/mL in 10 mmol/L phosphate buffer (pH 7.0). The samples were scanned from the wavelengths of 190 to 250 nm. The following parameters were used: step resolution, 1 nm; acquisition duration, 1 s; bandwidth, 0.5 nm. The CD spectra of the samples were corrected by subtracting of the spectrum of the protein-free buffer.
Intrinsic Fluorescence Spectroscopy
Emission spectra of intrinsic fluorescence of the samples were determined on a Cary Eclipse Fluorophotometer (Varian, Palo Alto, CA, USA). Intrinsic fluorescence spectroscopy measurements were performed in quartz cuvette of 1 cm with a protein concentration of 0.5 mg/mL in 10 mmol/L phosphate buffer (pH 7.0). Protein solutions were excited at 275 nm, and emission spectra were recorded from 300 to 400 nm at a constant slit of 5 nm for both excitation and emission.
Protein Solubility (PS)
The pH of aqueous solution (1%, w/v) of the protein sample was adjusted to the desired value (pH 2.0–10.0) with either HCl (0.4 mol/L) or NaOH (0.4 mol/L). The pH was readjusted if necessary after being stirred for 30 min, and then the solution was centrifuged at 8000 g for 20 min. After an appropriate dilution of the supernatant, the protein content of the supernatant was determined. The PS was expressed as the proportion of soluble protein to total proteins and the protein content of the dispersion (1%, w/v) in 0.1 mol/L NaOH was used as the total protein content.
Functionality Tests
Foaming and emulsifying properties
Foam capacity (FC) and foam stability (FS%) of DSSP were determined according to the method described by Hojilla-Evangelista et al.[Citation13] The foam volume was measured after 1 min and the increase in volume of the foam was expressed as foam capacity. Foam stability was measured by the decrease of the foam at 30 min. Emulsification activity (EA) and emulsification stability (ES) of DSSP were tested following the method of Wang et al.[Citation14] EA and ES were calculated as the ratio of emulsion layer height to the total height.
Water holding and fat absorption capacities (WHC and FAC)
WHC and FAC of DSSP were determined using the method described by Tomotake et al.[Citation15] with slight modifications. For WHC, briefly, accurately each 0.25 g of the DSSP was weighed into 10-mL pre-weighed centrifuge tubes, and then 5 mL de-ionized water was added to the tubes. For FAC, accurately 0.50 g of the DSSP was weighed into 10-mL centrifuge tubes pre-weighed and mixed with 3 mL of peanut oil thoroughly. All other steps and calculations were done exactly as described in the original method.
Determination of Protein Contents
Total proteins of the SSP and DSSP were determined using the micro-Kjeldahl method with nitrogen conversion factor of 6.25. Whenever necessary, the contents of soluble proteins were analyzed by using bovine serum albumin as the standard protein following the method described by Bradford.[Citation16] Appropriate solvent and reagent blanks were included in each assay.
Statistical Analysis
Data are reported as mean ± SD (standard deviation) of triplicate determinations. One-way analysis of variance (ANOVA) of the data was performed, and a least significant difference (LSD) test with confidence interval of 95% was used to compare the means.
RESULTS AND DISCUSSION
Proximate Composition
The crude fat of SSP had been determined by our previous study,[Citation17] with the content of 46.84%. The proximate analysis showed that of the SSP contained 5.50% moisture, 6.30% ash, 6.46% crude fiber, 14.87% NFE, and crude fat. The crude protein in SSP was similar to that obtained under similar conditions (19.58%).[Citation5] It is possible to produce value-added byproducts (dietary oil or protein) resulting from the high fat and protein content of the S. marianum seeds.
Protein Fractions and Protein Content
The obtained protein fractions are presented in . The yields of protein fractions indicated that albumin was the dominant fractions, followed by globulins with a small amount of glutelins and prolamins. Conforti and Lupano also found that about 70% of the proteins of the gametophyte of araucaria angustifolia and araucaria araucana were soluble in distilled water (albumin proteins).[Citation18] The protein contents of protein fractions varied considerably. SPI had the highest protein content compared with the protein fractions. However, albumins play an essential role in seeds because they are responsible for both enzymatic and metabolic activities in the seeds.[Citation19] In addition, albumins are of nutritional importance because of their high contents of essential amino acids and sulphur amino acids.
Table 1 Protein contents of protein fractions as well as protein isolate from S. marianu m seeds
Polyphenol Contents
Polyphenols contents of DSSP, proteins fractions, as well as SPI are presented in . The polyphenols contents of individual protein fractions and SPI were significantly different. SGL had the highest total polyphenols content, followed by SA, SG, and SPI. The difference of total polyphenols contents among individual protein fractions and SPI may be attributed to the different extent of interactions between the polyphenols and the proteins by different preparation. In these protein fractions, the form of the polyphenols was also diverse. Around half of the polyphenols were present in the free form in the DSSP, SA, and SPI, whereas in SG and SGL were only 36.15 and 12.85%, respectively. The result indicated that SG and SGL more preferably interacted with the polyphenols to form polyphenol–protein complexes, through covalent linkage bonds. The interaction form of the polyphenols in DSSP and SPI was similar with that in SA, which was the dominant fraction. Most of polyphenols in the SGL (87.15%) were in the protein-bound form. The covalent interactions between polyphenols and proteins may be favored by the alkali conditions in the presence of the polyphenols,[Citation20] which presumably account for this phenomenon.
Table 2 Polyphenol contents of defatted powders of S. marianu m seed, protein fractions, as well as protein isolate from S. marianu m seeds
Amino Acid Compositions
The amino acid compositions of the various protein fractions are shown in . The SSP, protein fractions, and SPI were richer in polar amino acids (aspartic acid/asparagine, glutamic acid/glutamine, and arginine), which were the common characteristics of all storage proteins.[Citation21] The seed proteins also included all essential amino acids (exception of tryptophan, not be determined in the present study) recommended by FAO/WHO for 2–5 year olds.[Citation22] Infants have very critical nutritional requirements due to rapid growth and immaturity of gastrointestinal function, and nine amino acids have been identified to be essential for infants. As shown in , the percentages of histidine, valine, isoleucine, phynylalanine + tyrosine of the SSP, protein fractions and SPI were higher than their counterparts stated in the FAO/CitationWHO (1990) standard. However, the overall quality of the SSP, protein fractions, and SPI were slightly compromised by the low level of lysine, which accounted for between 67.07 to 76.90% of the lysine requirement for children as recommended by the FAO/WHO.[Citation22]
Table 3 Amino acid composition of SSP, protein fraction, and SPI, and the FAO/WHO indicated requirements (2–5 years old) for the essential amino acids.a
SDS-PAGE Analysis
SDS-PAGE band patterns of protein fractions and SPI under reducing conditions are presented in . SDS-PAGE band patterns of the SPI indicated a broad range of polypeptides (16.3 to 112.1 kDa) (, lane 2). The most intense and widest bands resolved between 23.0 and 36.9 kDa. SA showed 12 distinct bands, similar to SPI. The most concentrated bands of SA resolved at 23.0 kDa, 32.9–40.9 kDa. The 13 bands of SG were in the MW range of 23.0–40.9 kDa, and 85.7–112.1 kDa (, lane 4). There was only one lighter band with MW of 23.3kDa that resolved at SP (, lane 5). The bands of SGL (, lane 6) were comparably minor but still detectable. The most concentrated bands resolved between 23.0 and 40.9 kDa.
Figure 1 SDS-PAGE band patterns of protein fractions and SPI under reducing conditions. Lanes 1–6: (1) molecular mass markers; (2) SPI: protein isolates; (3) SA: albumin fraction; (4) SG: globulin fraction; (5) SP: prolamin fraction; (6) SGL: glutelin fraction. Protein concentration, 4 mg protein/mL; Sample load volume, 15 μL. (Color figure available online.)
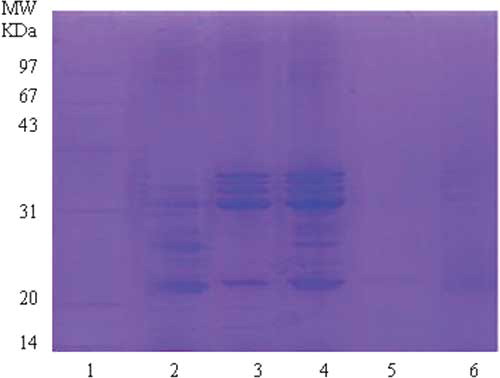
Conformational Characterization
Secondary conformation
Secondary structure elements, such as α-helices and β-types, have dichroic activity in the wavelength range from 190 to 260 nm.[Citation23] showed far-UV CD spectra of the protein fractions and SPI at 190–250 nm, in the far-UV region. The spectra showed a typical shape and feature of the mainly β-types secondary structure, i.e., negative bands at 216–218 nm and 220–230 nm (weak) and positive bands at 195–198 nm. The secondary structure compositions (including α-helices, β-strands, β-turns, and random coils) of these samples were calculated by their far-UV CD spectra according to the CONTIN/LL program in CDPro software (BBSRC, Swindon, UK; used for analysis DICHROWEB, University of London, UK). The calculations, which gave estimates of secondary structural components for various proteins, were shown in . The far-UV CD spectra and the secondary structure compositions of SA were similar to those of SPI (, ). This phenomenon was consistent with SA (the most dominant fraction of S. marianum seed proteins) having similar protein constituent patterns as SPI. As shown in and , the SGL fraction has a typical shape and feature of mainly β-types secondary structure, containing the highest content of β-turns structures. In the case of SG, a slight shift (about 3 nm) in band at 195 nm towards lower wavelength was observed (relative to SPI in band at 198 nm), which also was an indirect indication of a high content of α-helix. The results on the α-helices and β-strands contents were consistent with the conclusion that the globulins from plant seed mainly belong to the class of β-types proteins.[Citation24]
Table 4 Secondary structure compositions of various protein fractions and SPI calculated from far-UV CD spectrum using the CONTIN/LL program in CDPro software
Fluorescence spectroscopy
The tertiary conformation of these proteins was further analyzed by the intrinsic emission fluorescence spectroscopy, as given in . The determination chiefly carried out by the polarity of the environment of the tryptophan residues, which provided a sensitive means of monitoring conformational changes in proteins, and protein–protein as well as ligand–protein interactions.[Citation25] The maximum fluorescence emission spectrum suffers a red-shift when chromophores become more exposed to the solvent.[Citation11] The quantum yield of fluorescence decreases when the chromophores interact with quenching agents either in a solvent or the protein itself. The SG exhibited a fluorescence emission spectrum with a maximum at 347.8 nm, which is the characteristic fluorescence profile of tryptophan residues in a relatively hydrophobic environment.[Citation26] Maximum emission spectrum for SGL was at 352.0 nm (4.2 nm red-shift relative to SG), and the quantum yield of fluorescence was less (), which indicated some tryptophan residues exposed to the exterior of the proteins. This difference in fluorescence intensity may be attributed to the difference in quenching agents (such as polyphenols) contents, since they would cause quenching of fluorescence intensity in the region between 300–400 nm by interaction of the aromatic ring in the polyphenols with those of aromatic residues, e.g., tryptophan and tyrosine residues.[Citation27] The maximum of the emission spectra of SPI and SA were at 357.9 and 361.1 nm, respectively, which were the characteristic profiles of tryptophan residues in a much more hydrophilic environment.[Citation28] Quantum yields for the fluorescence of SPI and SA were also much less, but the fluorescence spectra were much broader, compared with those of SG or SGL. The results indicated the highly flexible tertiary conformation for SPI and SA, which were in agreement with the observation described by Tang and Wang.[Citation11] For the albumin from common buckwheat seeds, the maximum of the emission spectra suffered red-shift from globulin, and the quantum yield of fluorescence of albumin was much less than that of globulin.
Protein Solubility-pH Profiles
The pH-dependent protein solubility profiles of the DSSP, protein fractions, and SPI were presented in . Isoelectric points of the proteins from the S. marianum seeds were between 4.0 and 5.0. Generally, solubility was reduced as the pH increased until it reached the isoelectric point, followed by a progressive increase in solubility with further increase in pH. The similar results were reported in a previous study, which found that minimum solubility of protein isolates from Bambara Groundnut (Voandzeia subterranean L.) occurred at pH 5, and the isolates exhibited higher solubility than the corresponding isoelectric (IEP) isolates over all pH values.[Citation29] The different PS patterns of the DSSP, protein fractions, and SPI were observed. The PS profile of SA was much less pH-dependent over the tested pH range, relative to DSSP, SG, and SGL (). The PS of SA was in the range of 38–90% at pH 2–10. At pH 5, the PS of DSSP, SG, and SGL was only 16.70, 11.16, and 8.80%, respectively. As shown in , the PS profile of SA was similar to that of SPI. This phenomenon was consistent with SA having the similar protein constituent patterns as SPI, the most dominant of S. marianum seed proteins. In the case of SG, it exhibited a PS-pH typical profile of a number of globulins from many plant seeds, e.g., soy globulins and buckwheat globulins. The results suggested that the albumin fraction would have good potential for use in acidic protein-fortified beverages, e.g., protein-rich carbonated beverages,[Citation30] since protein solubility largely affects other functionalities, such as emulsifying, foaming, and gelating. The high solubility of the proteins indicated their promising food applications.
Figure 4 Protein solubility profiles of the protein fractions and SPI as a function of pH. The PS was expressed as proportion of soluble proteins to total proteins. Each datum point was the mean and standard deviation of triplicate determinations. DSSP: defatted powders of S. marianum seed (-■-); SA (-▵-); SG (-□-); SGL (-★-); and SPI (-○-).
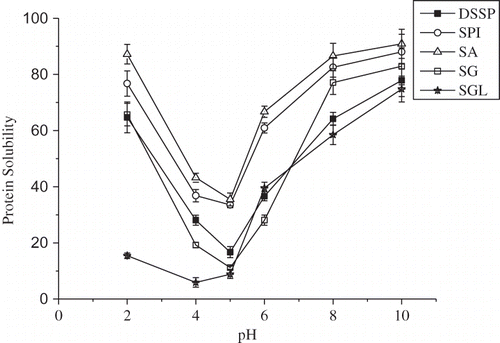
Foaming and Emulsifying Properties
Foam capacity and its stability for DSSP were reported, since foaming is an important characteristic feature of most of seed proteins. The data were presented in . The DSSP showed a considerable amount of foam capacity, which was higher than those of seed powders in akee (27.05%),[Citation31] and similar to that of milkweed seeds (101 mL),[Citation13] but lower than that of soyflour soybean protein (135 mL) generated by the same concentration.[Citation32] The foam stability after 30 min for the DSSP was higher than that of milkweed seeds (40%),[Citation13] but lower than those of soybean, which was reported to retain 95% of its foam at the end of the allotted standing time.[Citation32] The emulsion ability of DSSP was greater than that of akee proteins (Bilphia sapida) (25.65%).[Citation31] For the DSSP tested, EA was lower than ES, which indicated the strengthening and stabilizing of emulsion due to heating at 80°C prior to centrifugation. Lamsal et al.[Citation33] also reported stabilizing of alfalfa soluble leaf proteins emulsion by heating.
Table 5 Functional properties of defatted powders of S. marianum seed.a
Water Holding and Fat Absorption Capacities
WHC of a protein plays an important role in imparting characteristics, such as texture, viscosity, and adhesion.[Citation13] As shown in , the WHC of DSSP was higher than that of Gum karaya (Sterculia urens L.) seed meal (1.11 g water/g protein),[Citation34] nearly equal to 3.70 g water/g protein reported for milkweed seed protein.[Citation13] In the case of FAC, the value of DSSP was higher than that of Gum karaya (Sterculia urens L.) seed meal (1.14 g fat/g protein),[Citation34] nearly equal to 1.50 g fat/g protein reported for buckwheat protein.[Citation10]
CONCLUSION
Extraction, physicochemical, and functional properties of proteins from the S. marianum seeds was investigated. These properties varied among the various protein fractions and SPI, with albumins fraction being the most abundant seed proteins. Essential amino acids in the seeds favorably compared with the recommended level for pre-school children. The protein fractions were composed of the glycopeptides with an estimated molecular mass range of 16.3–112.1 kDa. Circular dichroism (CD) spectra indicated that the secondary conformations of protein fractions from the S. marianum seeds were rich in β-types, though these protein fractions and SPI had some differences in their secondary conformations. The maximum emission spectrum of SA was at 361.1 nm, which was a characteristic profile of tryptophan residues in the most hydrophilic environment. Functional properties indicated that S. marianum seed proteins could be used as a protein extender in adhesives or used in formulating acid foods, such as meat and milk analogue products and protein-rich beverages.
ACKNOWLEDGMENTS
The authors gratefully acknowledge Professor B. A. Wallace and Dr. L. Whitmore at the University of London for providing the CDPro software. The authors also are thankful to Chief Pharmacist Chen Li of the Food and Drug Administration Bureau of Zhenjiang for positively identifying S. marianum seed samples.
REFERENCES
- Flora , K. , Hahn , M. , Rosen , H. and Benner , K. 1998 . Milk thistle (Silybum marianum) for the therapy of liver disease . The American Journal of Gastroenterology , 93 ( 2 ) : 139 – 143 .
- Sobolová , L. , Skottová , N. , Vecera , R. and Urbánek , K. 2006 . Effect of silymarin and its polyphenolic fraction on cholesterol absorption in rats . Pharmacological Research , 53 ( 2 ) : 104 – 112 .
- Kvasnicka , F. , Bíba , B. , Sevcík , R. , Voldrich , M. and Krátká , J. 2003 . Analysis of the active components of silymarin . Journal of Chromatography A , 990 ( 1–2 ) : 239 – 245 .
- Ding , L. 2009 . “Zhaoyang” variety of silybum marianum . Modern Chinese Medicine , 11 ( 8 ) : 38 – 40 .
- Chen , Y. and Wang , C. 1998 . Basic research on comprehensive utilization of Silybum marianum . Acta Agriculturae Boreali Occidentalis Sinica , 7 ( 1 ) : 79 – 81 .
- Hadolin , M. , Skerget , M. , Knez , Z. and Bauman , D. 2001 . High pressure extraction of vitamin E-rich oil from Silybum marianum . Food Chemistry , 74 ( 3 ) : 355 – 364 .
- Kinsella , J.E. 1976 . Functional proteins of proteins in foods: A survey . Journal of Food Science , 37 ( 3 ) : 219 – 280 .
- AOAC . 1990 . Official Methods of Analysis , 15th , Washington , DC : Association of Official Analytical Chemists .
- Osborne , T.B. and Mendel , L.B. 1914 . Nutritive properties of proteins of the maize kernel . The Journal of Biological Chemistry , 18 : 1 – 16 .
- Tang , C.-H. 2007 . Functional properties and in vitro digestibility of buckwheat protein products: Influence of processing . Journal of Food Engeering , 82 ( 4 ) : 568 – 576 .
- Tang , C.-H. and Wang , X.-Y. 2010 . Physicochemical and structural characterisation of globulin and albumin from common buckwheat (Fagopyrum esculentum Moench) seeds . Food Chemistry , 121 ( 1 ) : 119 – 126 .
- Laemmli , U.K. 1970 . Cleavage of structural proteins during assembly of the head of bacteriophage T4 . Nature , 227 ( 5259 ) : 680 – 685 .
- Hojilla-Evangelista , M.P. , Evangelista , R.L. and Wu , V.Y. 2009 . Characterization of milkweed (Asclepias spp.) seed proteins . Industrial Crops and Products , 29 ( 2–3 ) : 275 – 280 .
- Wang , J.C. and Kinsella , J.E. 1976 . Functional properties of novel protein: Alfalfa leaf proteins . Journal of Food Science , 41 ( 2 ) : 286 – 292 .
- Tomotake , H. , Shimaoka , I. , Kayashita , J. , Nakajoh , M. and Kato , N. 2002 . Physicochemical and functional properties of buckwheat protein product . Journal of Agricultural and Food Chemistry , 50 ( 7 ) : 2125 – 2129 .
- Bradford , M.M. 1976 . A rapid and sensitive method for the quantitation of microgram quantities of protein utilizing the principle of protein-dye binding . Analytical Biochemistry , 72 ( 1–2 ) : 248 – 254 .
- Li , F. , Yang , L. , Zhao , T. , Zhao , J. , Zou , Y. , Zou , Y. and Wu , X. 2010 . Optimization of enzymatic pretreatment for n-hexane extraction of oil from Silybum marianumseeds using response surface methodology . Food and Bioproducts Processing , DOI: 10.1016/j.fbp.2011.02.010
- Conforti , P.A. and Lupano , C.E. 2011 . Selected properties of Araucaria Angustifolia and Araucaria Araucana seed protein . International Journal of Food Properties , 14 : 84 – 91 .
- Adebowale , Y.A. , Adeyemi , I.A. , Oshodi , A.A. and Niranjan , K. 2007 . Isolation, fractionation and characterisation of proteins from Mucuna bean . Food Chemistry , 104 ( 1 ) : 287 – 299 .
- Rawel , H.M. , Rohn , S. and Kroll , J. 2003 . Influence of a sugar moiety (rhamnosylglucoside) at 3-O position on the reactivity of quercetin with whey proteins . International Journal of Biological Macromolecules , 32 ( 3–5 ) : 109 – 120 .
- Carbonaro , M. , Cappelloni , M. , Nicoli , S. , Lucarini , M. and Carnovale , E. 1997 . Solubility−digestibility relationship of legume proteins . Journal of Agricultural and Food Chemistry , 45 ( 9 ) : 3387 – 3394 .
- FAO/WHO . 1990 . Protein quality evaluation. Report of Joint FAO/WHO Expert Consultation , Rome , , Italy : FAO/WHO .
- Kelly , S.M. , Jess , T.J. and Price , N.C. 2005 . How to study proteins by circular dichroism . Biochimica et Biophysica Acta–Proteins and Proteomics , 1751 ( 2 ) : 119 – 139 .
- Zirwer , D. , Gast , K. , Welfle , H. , Schlesier , B. and Dieter , S.K. 1985 . Secondary structure of globulins from plant seeds: A re-evaluation from circular dichroism measurements . International Journal of Biological Macromolecules , 7 ( 2 ) : 105 – 108 .
- Pallarès , I. , Vendrell , J. , Avilès , F.X. and Ventura , S. 2004 . Amyloid fibril formation by a partially structured intermediate state of [alpha]-chymotrypsin . Journal of Molecular Biology , 342 ( 1 ) : 321 – 331 .
- Dufour , E. , Hoa , G.H.B. and Haertlé , T. 1994 . High-pressure effects on [beta]-lactoglobulin interactions with ligands studied by fluorescence . Biochimica et Biophysica Acta–Protein Structure and Molecular Enzymology , 1206 ( 2 ) : 166 – 172 .
- Suryaprakash , P. , Kumar , R.P. and Prakash , V. 2000 . Thermodynamics of interaction of caffeic acid and quinic acid with multisubunit proteins . International Journal of Biological Macromolecules , 27 ( 3 ) : 219 – 228 .
- Shifrin , S. , Luborsky , S.W. and Grochowski , B.J. 1971 . L-Asparaginase from E. Coli. B. Physico-chemical studies of the dissociation process . The Journal of Biological Chemistry , 246 : 7708 – 7714 .
- Adebowale , Y.A. , Schwarzenbolz , U. and Henle , T. 2011 . Protein isolates from Bambara Groundnut (Voandzeia Subterranean L.): Chemical characterization and functional properties . International Journal of Food Properties , 14 : 758 – 775 .
- Kinsella , J.E. 1979 . Functional properties of soy protein . Journal of the American Oil Chemists’ Society , 56 ( 3 ) : 242 – 257 .
- Akintayo , E.T. , Adebayo , E.A. and Arogundade , L.A. 2002 . Chemical composition, physicochemical and functional properties of akee (Bilphia sapida) pulp and seed flours . Food Chemistry , 77 ( 3 ) : 333 – 336 .
- Hojilla-Evangelista , M.P. , Sessa , D.J. and Mohamed , A. 2004 . Functional properties of soybean and lupin protein concentrates produced by ultrafiltration–diafiltration . Journal of the American Oil Chemists’ Society , 81 ( 12 ) : 1153 – 1157 .
- Lamsal , B.P. , Koegel , R.G. and Gunasekaran , S. 2007 . Some physicochemical and functional properties of alfalfa soluble leaf proteins . LWT–Food Science and Technology , 40 ( 9 ) : 1520 – 1526 .
- Galla , N.R. and Dubasi , G.R. 2010 . Chemical and functional characterization of Gum karaya (Sterculia urens L.) seed meal . Food Hydrocolloids , 24 ( 5 ) : 479 – 485 .