Abstract
Moisture adsorption isotherms of freeze‐dried bovine colostrum powder were obtained using the gravimetric method at three temperatures (15, 25, and 35°C) in the water activity range of 0.11–0.86. The moisture adsorption isotherms of freeze‐dried bovine colostrum powder showed a sigmoid isotherm curve typical of the type II BET classification shape. The Guggenheim-Anderson-de Boer and Brunauer-Emmett-Teller models were recommended to fit experimental data well. The shelf life of freeze‐dried bovine colostrum powder packaged in aluminium-laminated polyethylene pouches was evaluated for 243 days, and the storage stability was investigated when stored at 25°C and 50% relative humidity. A slight increase in moisture content, a linear increase in color difference, and a slight decrease in IgG concentration were found within 60 days storage under the above-mentioned conditions.
INTRODUCTION
Bovine colostrum is the initial milk secreted by cows within the first 4 days after parturition.[Citation1] It contains abundant bioactive components, including growth factors, immunoglobulins (Igs), lactoperoxidase, lysozyme, lactoferrin, cytokines, nucleosides, vitamins, peptides, and oligosaccharides, which are of increasing relevance to human health.[Citation2] Adding bovine Igs into infant formula and other foods may help to reduce viral and microbial infections, and may provide consumers with improved immune activity.[Citation3] In addition, IgG, the principal Ig of bovine colostrum, provides the major components of passive immunity to the new-born calf.[Citation4] Drying is a traditional method for preservation of foods, which can reduce transportation and storage cost and extend the shelf life. Moreover, freeze drying has been commonly employed for processing and preserving highly heat-sensitive and high-added-value food products, such as fish, meat, and coffee.[Citation5] Colostrum-based products obtained by freeze drying have been reported by other researchers.[Citation6,Citation7]
The concept of water activity is now being used although it has some limitations, because it has been observed and verified by many researchers that the active water could be much more important to the stability of some food than the total amount of water present.[Citation8–10 Citation Citation10 Additionally, a food product is most stable at its monolayer moisture content.[Citation10] Therefore, the sorption isotherms of foodstuffs are important tools because they can describe relationships between equilibrium moisture content (EMC) and water activity, which are dependent on structure and composition of the food material, as well as pressure and temperature.[Citation11] For drying process and storage of food materials, the knowledge of their sorption behavior is essential, as sorption isotherms are helpful for establishing the final moisture contents; evaluating energy requirements of drying; and predicting chemical, physical, and microbial stability of foods as a function of moisture content of the product. Until now, many mathematical equations have been proposed for describing the sorption isotherm and applied in a lot of foods and biological materials.[Citation12] However, there are very few reports on moisture sorption isotherms of freeze-dried bovine colostrum (FDBC) powder, whose composition differs from ruminant milk powder in established lactation.
The stability of FDBC powders during storage is significantly affected by moisture content or water activity of FDBC powders at a given temperature. In addition, water content also affects the susceptibility of protein to heat denaturation. In general, as the amount of water sorbed by protein is reduced, the protein becomes more resistant to heat denaturation.[Citation13] For a low-moisture food product, shelf life prediction by theoretical calculations is practical because actual storage testing, using a long-term stability study, is time consuming and costly because of regular analysis at each time interval during storage. The theoretical shelf life calculation methods are based on the relationship between moisture content of a food product, storage environment, and barrier property of packaging material,[Citation14] and have been commonly used for predicting shelf life of moisture-sensitive foods, such as mango soy-fortified yogurt powder,[Citation15] and spray-dried yogurt powder.[Citation16] Packaged food materials at a certain storage period are subjected to moisture gain depending on a number of factors: the storage temperature and relative humidity, the sorption properties of the product, the water activity gradients relative to the storage atmosphere, and the water vapor permeability of the packaging materials.[Citation14]
The objectives of this study were to provide experimental data for the sorption characteristics of FDBC powder at the temperatures of 15, 25, and 35°C in the water activity range of 0.11–0.86 and to model the sorption isotherms with the Brunauer-Emmett-Teller (BET) and Gugenheim-Anderson-de Boer (GAB) equations. In addition, the storage stability of FDBC powder packaged in aluminium-laminated polyethylene (ALPE) pouches and stored at 25°C, 50% relative humidity (RH) for 60 days was investigated in terms of quality parameters, including moisture content, color, and IgG concentration; shelf life of FDBC powder under the above-mentioned storage condition was predicted by theoretical calculation based on critical moisture content of FDBC powder.
MATERIALS AND METHOD
Materials
Fresh bovine colostrums used in this experiment were provided by a dairy farm of Bright Dairy and Food Co., Ltd., Shanghai, China and employed with a constant total solids mass concentration of 9.59 ± 0.01, containing fat of 0.74 ± 0.02, protein of 4.93 ± 0.01, lactose of 2.96 ± 0.01, ash of 0.956 ± 0.002 g/100 g samples, respectively. FDBC powders used in the adsorption experiment were obtained by drying the fresh bovine colostrum in a freeze dryer (Shanghai Yucheng Dryer Equipment Co., Ltd., Shanghai, China) in one batch. The plate temperature was maintained at 35°C with a vacuum of 70–130 Pa in the chamber, while condensing plate temperature was kept at −60°C, and drying continued up to 72 h. All the samples were transformed into powder and stored in a desiccator with silica gel until used for experiments. For the storage experiment, the aluminium-laminated polyethylene (ALPE) films were purchased from a local market.
Moisture Adsorption Isotherm of FDBC Powder
The adsorption isotherms of FDBC powder were determined using the standard static-gravimetric technique as recommended by the COST 90 project.[Citation17] Seven saturated salt solutions selected to give different relative levels of humidity in the range of 0.11–0.86 were employed. The salt solutions used and their corresponding relative levels of humidity at different temperatures were taken from data reported by Greenspan.[Citation18]
Bovine colostrum powders collected from a freeze dryer were kept in vacuum desiccators over P2O5 at room temperature. Desiccated powders were further dried in a vacuum oven at 30°C for 5–7 days before experiments so as to remove residual moisture. Every three replications of FDBC powder samples (1 ± 0.001) g were placed in previously weighed glass sorption jars, and then quickly placed inside desiccators over seven salt solutions of known relative humidity. A test tube containing thymol was placed inside the desiccators with high relative humidity to prevent mould growth during storage. The desiccators were placed in a temperature-controlled cabinet, with an accuracy of ±1°C at the selected temperatures of 15, 25, and 35°C. FDBC powder samples were weighed at intervals of 24 h. The total time for removal, weighing, and putting back the samples in the desiccators were about 30 s. This minimized the degree of atmospheric moisture sorption during weighing. Equilibrium was reached when the sample weight difference between two successive measurements was less than the balance accuracy of 0.001 g. This involved a period of approximately 3 weeks. EMC of the samples was determined using AOAC method 927.05.[Citation19]
Modeling of Moisture Adsorption Isotherms of FDBC Powder
The BET and GAB equations describing physical phenomena were commonly used for describing the relationship between EMC and water activity.[Citation12,Citation20] The GAB model has been particularly successful for water activities up to 0.9 and is presented in EquationEq. (1):
The BET equation describes most of the type II isotherms in water activity ranging from 0.05 to 0.45,[Citation12] and is presented in EquationEq. (2):
Prediction of Shelf Life and Assessment of Quality Changes in FDBC Powder During Storage
Eighteen aluminium-laminated polyethylene (ALPE; 12 μm PET, 8 μm Al, 80 μm PE) pouches (140 mm × 135 mm) were filled with (6 ± 0.5) g of FDBC powders with intitial moisture content of 5.145 g H2O/100 g dry solids and were closed by heat sealing, avoiding any air space but without vacuum application. The water vapor permeability, k (g m−2 day−1 Pa−1), of the packaging material (ALPE) was measured using a water vapor permeability analyzer (Mocon Permatran-W, 3/61, Mocon Inc., Minneapolis, MN, USA). ALPE sealed pouch samples were placed in a binder constant climate chamber (constant climate chamber KBF series, Binder GmbH, Tuttlingen, Germany) setting the temperature at 25°C and relative humidity of 50%. Storage was carried out for 60 days and each pouch was exposed to the same environment. Three samples of ALPE pouches were taken out from the chamber at the time of 0 d, 7 d, 15 d, 30 d, 45 d, and 60 d during 60 days’ storage and analyzed for quality parameters, such as moisture content, color, and IgG concentration. All tests were done in triplicate and additional parallels were analyzed if the individual values from the triplicates deviated more than 1% from the triplicate mean. The shelf life was calculated using the following equation:[Citation14]
The moisture contents were determined by AOAC method 927.05.[Citation19] The color values of powder samples were measured as CIE parameters L, a, and b with a color difference meter (Model WSC-S, Shanghai Precision and Scientific Instrument Co., Ltd., Shanghai, China). The L, a, and b values of the fresh as well as stored powder were measured and using fresh bovine colostrum as a reference, the net (total) color difference (ΔE) was calculated using EquationEq. (4):
An aliquot (1 ± 0.1) g samples of FDBC powder were assayed for IgG concentration by the single radial immune-diffusion procedure of Fahey and McKelvey[Citation22] and the results were expressed as (g/100 g dry solids). The samples were prepared by an acid coagulation procedure.[Citation23] Three replications of the experimental procedures mentioned above were carried out.
Statistical Analysis
A nonlinear regression analysis by the least square method was carried out to fit the two equations using the SAS 9.2 software program (SAS Institute Inc., Cary, NC, USA). Data for storage stability were also subjected to the analysis of variance (ANOVA) using the above-mentioned software. The equation predicting shelf life of FDBC powder was analyzed using the Mathematica 6.0 software program (Wolfram Research, Inc., Champaign, IL, USA).
RESULTS AND DISCUSSION
Moisture Adsorption Isotherms
Moisture adsorption isotherms of FDBC powder at temperatures of 15, 25, and 35°C are presented in . EMC at each aw represents the mean value of three replications. The standard deviation of each experimental point (kg/kg dry solids) was within the range of 0.001 to 0.03. At all three temperatures, the adsorption isotherms of FDBC powder showed the sigmoidal type BET II shaped curve, which was common for many amorphous solids.[Citation24] Similar results were reported by many researchers in other dairy products, including powdered milk,[Citation25] chhana podo,[Citation26] dried acid casein,[Citation27] and yogurt powder.[Citation16,Citation28]
It was easily found that at a constant temperature, EMC values increased with an increase of water activity.[Citation16] The experimental data also indicated that EMC decreased with increasing temperature at all levels of water activity. The same results were reported by other researchers.[Citation29,Citation30] This trend may be attributed to different binding capacity of the substrate with free water at different temperatures. In general, proteins and carbohydrates, which are main components of FDBC powder, have more water-binding capacity at low temperatures than at high temperatures. Palipane and Driscoll[Citation31] suggested that at increased temperatures water molecules get activated to higher energy levels, causing them to become less stable and break away from the water-binding sites of the substrate, thus decreasing the EMC. Furthermore, an increase in temperature caused an increase of water activity at the same moisture content, which in turn caused an increase in the reaction rate leading to quality deterioration.[Citation32]
Table 1 Constants of the isotherm models for FDBC powder
Fitting of Equation to Sorption Isotherm Data
The parameters for two isotherm models used to predict adsorption isotherms of FDBC powder are presented in . It is clear from that the GAB and BET models gave a good fit to the experimental data with R 2 > 0.9 and E and RMSE values below 5%, which were considered to be acceptable for practical purposes. Similarly, Mortenson and his co-authers[Citation19] and Kim and Bhowmik[Citation28] reported that the GAB equation fitted well with experimental data with E values less than 5%. Additionally, it was found that SEE for both GAB and BET models were below 0.6, indicating that the equations fitted well for experimental data. The residuals for GAB and BET equations were random in pattern, which further verified that two models were suitable for describing the adsorption behavior of FDBC powder in the water activity range of 0.11–0.86 and 0.11–0.43, respectively. The results were consistent with the observations of Janjai and his co-authers[Citation33] and Sawhney and his co-authers,[Citation27] who recommended the GAB model for description of equilibrium isotherms of litchi and dried acid casein from buffalo skim milk based on RMSE values below 5% and a random distribution of the residuals and RMSE values below 10%, respectively. Furthermore, the applicability of the BET model was limited because the model hypothesis failed and the model was not able to predict sorption isotherm precisely when aw was beyond 0.50. In spite of the theoretical limitations of the BET model, the BET monolayer moisture content represented tightly bound water and was observed to be optimum water content for storage stability of dried food. M 0 obtained from the BET model at 35°C was 4.484 g H2O/100 g dry solids, comparable to 4.834 g H2O/100 g dry solids predicted from chhana podo using the BET model.[Citation26] The representative GAB and BET models for predicting adsorption isotherm of FDBC powder at 25°C were presented in .
Shelf Life of FDBC Powder
The initial moisture content of FDBC powder was 5.145 g H2O/100 g dry solids. The final moisture content was decided on the basis of the free flowing ability of the powder and its water activity. This moisture content was considered as critical moisture content and the corresponding water activity was calculated using the GAB parameters. The estimated GAB parameters M 0, C, and K at 25°C were 5.505 g H2O/100 g dry solids, 13.874, and 0.882, respectively. The area, A, of ALPE pouches was 0.0378 m2. aw (e) in this experiment was 50%, equal to the relative humidity in the climate chamber. The amount of dry solids, W, in the 6 g powder was 5.706 g. The integral part of the equation was estimated between the limits of initial moisture content: 5.145 g H2O/100 g dry solids; and critical moisture content: 6.856 g H2O/100 g dry solids. Ps at 25°C is 3.169 × 103 Pa. Water vapor permeability, P(T), of the ALPE pouches was experimentally determined as 1.58 × 10−8 kg m−2 day−1 Pa−1. By putting the values of W, P(T), A, Ps , Mi , Mc in the equation, the shelf life of FDBC powder was estimated for 243 days under the conditions of relative humidity of 50% and a temperature of 25°C.
Quality Changes of FDBC Powder During Storage
Adsorption of moisture can significantly affect the shelf life of FDBC powder. When FDBC powder gains too much moisture, it becomes lumpy or caking, making its quality out of consumers’ requirements. Change in moisture content during the storage of FDBC powder was presented in . Moisture content increased with storage time and was significantly affected by storage time according to the SAS 9.2 software (SAS Institute Inc., Cary, NC, USA) (p < 0.01). Moreover, the moisture content had a strong influence on the physicochemical stability of milk powder during storage and distribution and the oxidative stability of milk powder varied with the moisture content.[Citation34] Color change of FDBC powder with storage time was shown in and was significantly affected by storage time (p < 0.01). Color change increased linearly with storage time, which was similar to those in other dairy products, such as mango soy-fortified yogurt powder[Citation15] and the powdered milk.[Citation35] In bovine colostrum, IgG is the major immune component, indeed representing the majority of total colostral protein. Besides IgG stabilization during the freeze-drying process, adequate storage stability is of importance. Changes of IgG concentration with storage time were shown in and were significantly affected by storage time (p < 0.01). The IgG concentration showed a slight decrease with increasing storage time. It has been confirmed that denaturation of IgG was affected by many other factors, such as heat treatment, pH, microorganism, etc.[Citation36–38 Citation Citation38
Figure 3 Changes in moisture content (g H2O/100 g dry solids) of FDBC powder packaged in ALPE pouches during storage at 25°C and 50% RH.
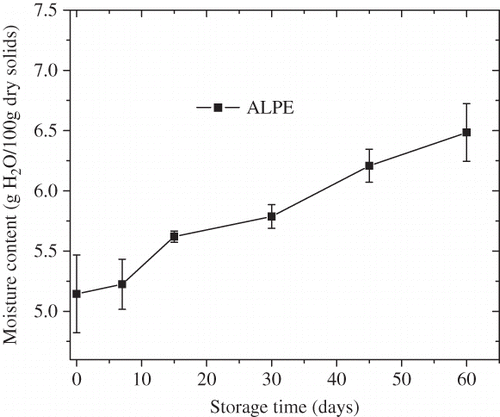
Kinetics of Quality Changes in FDBC Powder
Kinetic modeling is necessary to derive basic kinetic information for a system in order to describe the reaction rate as a function of experimental variables and, hence, to predict changes in a particular food during processing and storage.[Citation39] There are numerous references on the kinetics of color change and IgG denaturation of food materials in the literature.[Citation15,Citation36,Citation38,Citation40] The majority of these works report zero-order (EquationEq. 5) or first-order (EquationEq. 6) degradation reaction kinetics:
Table 2 Regression analysis of color change and IgG concentration from zero- and first-order reaction kinetics of FDBC powder packaged in ALPE pouches
CONCLUSION
The moisture adsorption isotherms of FDBC powder showed a sigmoid isotherm curve typical of the type II BET classification shape. The equilibrium moisture content of FDBC powder increased with decreasing temperature at a given water activity at three temperature levels of 15, 25, and 35°C. The GAB and BET equations were recommended for describing the adsorption behavior of FDBC powder in the temperature range of 15–35°C and a water activity range of 0.11–0.86 and 0.11–0.43, respectively. The shelf life of FDBC powder in ALPE pouches under a storage condition of 25°C and 50% RH was calculated for 243 days based on the critical moisture content of the powder and the package permeability. Within the 60-days storage period under the above-mentioned storage conditions, a slight increase in moisture content, a linear increase in color difference, and a slight decrease in IgG concentration were observed. Color change during storage followed the zero-order kinetics model and IgG denaturation followed the first-order kinetics model. In addition, D value at 25°C was calculated for 580 days.
ACKNOWLEDGMENTS
The authors gratefully acknowledge the National Department of Science and Technology of China under National Key Technology R&D Program (2006BAD04A14) for providing the financial support.
REFERENCES
- Gopal , P.K. and Gill , H.S. 2000 . Oligosaccharides and glycoconjugates in bovine milk and colostrums . British Journal of Nutrition , 84 : 69 – 74 .
- Michaelidou , A. and Steijns , J. 2006 . Nutritional and technological aspects of minor bioactive components in milk and whey: Growth factors, vitamins and nucleotides . International Dairy Journal , 16 ( 11 ) : 1421 – 1426 .
- Li , S.Q. , Zhang , H.Q. , Balasubramaniam , V.M. , Lee , Y.Z. , Bomser , J.A. , Schwartz , S.J. and Dunne , C.P. 2006 . Comparison of effects of high-pressure processing and heat treatment on immunoactivity of bovine milk immunoglobulin G in enriched soymilk under equivalent microbial inactivation levels . Journal of Agricultural and Food Chemistry , 54 ( 3 ) : 739 – 746 .
- Gapper , L. , Copestake , D. , Otter , D.E. and Indyk , H.E. 2007 . Analysis of bovine immunoglobulin G in milk, colostrum and dietary supplements: A review . Analytical and Bioanalytical Chemistry , 389 ( 1 ) : 93 – 109 .
- Sokhansanj , S. and Jayas , D.S. “ Drying of foodstuffs ” . In Handbook of Industrial Drying , 3rd , Edited by: Arun , S. and Mujumdar , A.S. 537 London, 2007 : CRC Press .
- Hsu , K.H. and Fennema , O. 1989 . Changes in the functionality of dry whey protein concentrate during storage . Journal of Dairy Science , 72 ( 4 ) : 829 – 837 .
- Klobasa , F. , Goel , M.C. and Werhahn , E. 1998 . Comparison of freezing and lyophilizing for preservation of colostrum as a source of immunoglobulins for calves . Journal of Animal Science , 76 ( 4 ) : 923 – 926 .
- Rahman , M.S. 2010 . Food stability determination by macro-micro region concept in the state diagram and by defining a critical temperature . Journal of Food Engineering , 99 ( 4 ) : 402 – 416 .
- Rahman , M.S. 2009 . Food stability beyond water activity and glass transtion: Macro-micro region concept in the state diagram . International Journal of Food Properties , 12 ( 4 ) : 726 – 740 .
- Rahman , M.S. 2006 . State diagram of foods: Its potential use in food processing and product stability . Trends in Food Science and Technology , 17 ( 3 ) : 129 – 141 .
- Mulet , A. , García-Pascual , P. , Sanjuán , N. and García-Reverter , J. 2002 . Equilibrium isotherms and isosteric heats of morel (Morchella esculenta) . Journal of Food Engineering , 53 ( 1 ) : 75 – 81 .
- Lewichi , P.P. 2009 . “ Data and models of water activity. II: Solid foods ” . In Food Properties Handbook , Edited by: Shafiur Rahman , M. 68 – 143 . London : CRC Press .
- Kinsella , J.E. , Fox , P.F. and Rockland , L.B. 1986 . Water sorption by proteins: Milk and whey proteins . Critical Reviews in Food Science and Nutrition , 24 ( 2 ) : 91 – 139 .
- Hernandez , R.J. and Giacin , J.R. 1997 . “ Factor affecting permeation, sorption and migration processes in packaged-product systems ” . In Food Storage Stability , Edited by: Taub , I.A. and Singh , R.P. 269 – 330 . New York : CRC Press .
- Kumar , P. and Mishra , H.N. 2004 . Storage stability of mango soy fortified yoghurt powder in two different packaging materials: HDPP and ALP . Journal of Food Engineering , 65 ( 4 ) : 569 – 576 .
- Koç , B. , Yilmazer , M.S. , Balkir , P. and Ertekin , F.K. 2010 . Moisture sorption isotherms and storage stability of spray-dried yogurt powder . Drying Technology , 28 ( 6 ) : 816 – 822 .
- Wolf , W. , Speiss , W.E.C. and Jung , G. 1985 . “ Standardization of isotherm measurements (COST Project 90 and 90 BIS) ” . In Properties of Water in Foods , Edited by: Simatos , D. and Multon , J.L. 661 – 677 . Dordrecht , , Netherlands : Martnus Nijhott Publishers .
- Greenspan , L. 1977 . Humidity fixed points of binary saturated aqueous solutions . Journal of Research of the National Bureau of Standard–Physics and Chemistry , 81 ( 1 ) : 89 – 96 .
- International , AOAC . 1990 . Official Methods of Analysis of AOAC , Gaithersburg , MD : AOAC International .
- Mortenson , M.A. , Labuza , T.P. and Reineccius , G.A. 2010 . Moisture sorption isotherms for un-modified and osan-substituted dextrin and gum acacia used as carrier materials for spray dried encapsulation of flavoring materials . International Journal of Food Properties , 13 ( 5 ) : 992 – 1001 .
- Boquet , R. , Chirife , J. and Iglesias , H.A. 1979 . Equations for fitting water sorption isotherms of foods . III. Evaluation of various three-parameter models. International Journal of Food Science and Technology , 14 ( 5 ) : 527 – 534 .
- Fahey , J.L. and McKelvey , E.M. 1965 . Quantitative determination of serum immunoglobulins in antibody-agar plates . Journal of Immunology , 94 ( 1 ) : 84 – 90 .
- David , E.J. , Copestake , H.E.I. and Otter , D.E. 2006 . Affinity liquid chromatography method for the quantification of immunoglobulin G in bovine colostrum powders . Journal of AOAC International , 89 ( 5 ) : 1249 – 1256 .
- Hiatt , A.N. , Taylor , L.S. and Mauer , L.J. 2011 . Effects of co-formulation of amorphous maltodextrin and deliquescent sodium ascorbate on moisture sorption and stability . International Journal of Food Properties , 14 ( 4 ) : 726 – 740 .
- Abdenouri , N. , Idlimam , A. and Kouhila , M. 2010 . Sorption isotherms and thermodynamic properties of powdered milk . Chemical Engineering Communications , 197 ( 8 ) : 1109 – 1125 .
- Jayaraj Rao , K. , Heartwin Amala Dhas , P. , Magdaline Eljeeva Emerald , F. , Ghosh , B.C. , Balasubramanyam , B.V. and Kulkarni , S. 2006 . Moisture sorption characteristics of chhana podo at 5°C and 35°C . Journal of Food Engineering , 76 ( 3 ) : 453 – 459 .
- Sawhney , I.K. , Sarkar , B.C. and Patil , G.R. 2010 . Moisture sorption characteristics of dried acid casein from buffalo skim milk . LWT–Food Science and Technology , 44 ( 2 ) : 502 – 510 .
- Kim , S.S. and Bhowmik , S.R. 1994 . Moisture sorption isotherms of concentrated yogurt and microwave vacuum dried yogurt powder . Journal of Food Engineering , 21 ( 2 ) : 157 – 175 .
- Vullioud , M. , Márquez , C.A. and De Michelis , A. 2006 . Equilibrium sorption isotherms and isosteric heat of rose hip fruits (Rosa Eglanteria) . International Journal of Food Properties , 9 ( 4 ) : 823 – 833 .
- Ikhu-Omoregbe , D.I.O. 2006 . Comparison of the sorption isotherm characteristics of two cassava products . International Journal of Food Properties , 9 ( 2 ) : 167 – 177 .
- Palipane , K.B. and Driscoll , R.H. 1992 . Moisture sorption characteristics of in shell macadamia nuts . Journal of Food Engineering , 18 : 63 – 76 .
- Van den Berg , C. and Bruin , S. 1981 . “ Water activity and its estimation in food systems: Theoretical aspects ” . In Water Activity: Influence on Food Quality , Edited by: Rockland , L.B. and Stewart , G.F. 1 – 61 . New York : Academic Press .
- Janjai , S. , Lamlert , N. , Tohsing , K. , Mahayothee , B. , Bala , B.K. and Müller , J. 2010 . Measurement and modeling of moisture sorption isotherm of litchi (Litchi Chinensis Sonn.) . International Journal of Food Properties , 13 ( 2 ) : 251 – 260 .
- Reh , C. , Bhat , S.N. and Berrut , S. 2004 . Determination of water content in powdered milk . Food Chemistry , 86 ( 3 ) : 457 – 464 .
- Nielsen , B.R. , Stapclteldt , H. and Skibsted , L.H. 1997 . Differentiation between 15 whole milk powders in relation to oxidative stability during accelerated storage: Analysis of variance and canonical variable analysis . International Dairy Journal , 7 ( 8–9 ) : 589 – 599 .
- Chen , C.C. and Chang , H.M. 1998 . Effect of thermal protectants on the stability of bovine milk immunoglobulin G . Journal of Agricultural and Food Chemistry , 46 ( 9 ) : 3570 – 3576 .
- Korhonen , H. , Marnila , P. and Gill , H.S. 2000 . Milk immunoglobulins and complement factors . British Journal of Nutrition , 84 ( Supplement S1 ) : 75 – 80 .
- Li Chan , E. , Kummer , A. , Losso , J.N. , Kitts , D.D. and Nakai , S. 1995 . Stability of bovine immunoglobulins to thermal treatment and processing . Food Research International , 28 ( 1 ) : 9 – 16 .
- Van Boekel , M.A.J.S. 1996 . Statistical aspects of kinetic modelling for food science problems . Journal of Food Science , 61 ( 3 ) : 477 – 486 .
- Fukumoto , L.R. , Li-Chan , E. , Kwan , L. and Nakai , S. 1994 . Isolation of immunoglobulins from cheese whey using ultrafiltration and immobilized metal affinity chromatography . Food Research International , 27 ( 4 ) : 335 – 348 .