Abstract
Crayfish protein, present in the wastes from crayfish processing, has been investigated as an ingredient of surimi-like gel products, which may be regarded as a renewable, available, and low-cost raw material. The aim of this study was to evaluate the influence of protein concentration on the gelation behaviour and gel properties of crayfish protein isolate-based systems. Gelation was performed by heating crayfish protein isolate dispersions at 90°C for 30 min. Then, gels were cooled at 4°C and the evolution of linear viscoelastic properties upon setting was analysed for 24 h. An increase in both linear viscoelasticity and water holding capacity was found as protein concentration increased, although an asymptotic evolution was found at the highest CFPI concentrations. Scanning electron microscopy revealed occurrence of an extended cross-linked network for crayfish protein isolate gels. These results suggest that crayfish protein can be properly used as a valuable ingredient in food gels, where protein concentration may be modulated to enhance gel strength.
INTRODUCTION
Red crayfish (Procambarus clarkii) is native to the United States and was introduced in Spain in 1974. Since its introduction, this crayfish species has experienced an invasive growth, generating important environmental problems.Citation[1] First, crayfish products were used mainly as a supplement in animal feed, or discarded as wastes, contributing an important focus of environment pollution. Nowadays, there is an important crayfish industry focused on the use of red crayfish as a high quality food product.Citation[2, Citation3] In fact, this crayfish is considered to be a source of a variety of valuable proteins of high nutritional value, being rich in essential amino acids. Crayfish products also contribute functional ingredients, such as polyunsaturated acids ω-3 and ω-6, as well as natural antioxidants, such as astaxanthin.Citation[4, Citation5] Moreover, some benefits derived from the use of protein from red crayfish have been described for the treatment and prevention of several diseases.Citation[6] However, an analysis of its functional properties is required in order to assess its usefulness as an ingredient in food applications.
In recent years, physicochemical properties of proteins from red crayfish were studiedCitation[7] as well as some of their functional properties, such as emulsifying activity affected by pH modification and thermal treatments,Citation[8, Citation9] ability to form gels of these proteins,Citation[10] and gel characterization as a function of pH values.Citation[11] However, the knowledge of how protein concentration may affect the ability of these proteins to form stable gels, showing suitable potential for the manufacture of surimi, is also very important. The market of surimi, which comes from Japan, has been developed in the last decades. The use of fish proteins to prepare surimi has been increased from its introduction in the United States market in the 1980s. Some estimates remark the consumption in 2003 to be around 90,000 tons in the USA and 120,000 tons in Europe, where France and Spain are the most important consumers.Citation[12]
Crayfish proteins are mainly constituted by miofibrillar, sarcoplasmatic, and low amounts of stromal proteins.Citation[13] Upon heating, these proteins may dissociate and associate in different ways to form gels. The ability of proteins to denature, form aggregates, and gel (i.e., by heat treatment, high pressure, or pH modification) is a key factor contributing to the microstructure and texture of several kinds of food systems. Sarcoplasmatic proteins, with a globular and relatively simple structure, show a weak gelation capacity and, therefore, a small contribution to the texture of processed foods (meat, fish, mollusc, or crustacean). On the other hand, myofibrillar proteins, especially myosin and actomyosin, constitute multiple domains that tend to form viscoelastic networks and gels with high consistency.Citation[14]
Thermal gelation of myofibrillar proteins is produced in several steps: (1) denaturation from the native state, (2) aggregation of denatured or partially denatured protein, and (3) formation of physical entanglements or intermolecular cross-links (reticulation). When the extension of aggregates reaches a critical value, a three-dimensional network is formed leading to gel formation. Aggregation only requires a previous partial denaturation, as has been demonstrated with myosinCitation[15] or globular proteins.Citation[16] Among other interactions, the most important to be taken into account are: hydrogen bonds,Citation[17] electrostatics interactions that participate mainly in the formation of myosin fibres,Citation[18] hydrophobic interactions that are a consequence of the surface hydrophobicity of protein and are favoured at a high temperature (at least 60°C),Citation[19] and covalent bonds between myosin molecules that contribute to gel strength.Citation[20] Some authors consider that both hydrophobic interactions and covalent disulfide bridges constitute the main mechanism of gel formation, as is the case for surimi gels subjected to thermal or high pressure treatments.Citation[21]
Rheological properties, such as linear viscoelasticity, have been extensively used to study the sol-gel transition along heatingCitation[22– Citation24] and are closely related to protein gel microstructure.Citation[25, Citation26] In fact, a combination of rheological and scanning electron microscopy techniques has been widely used to characterize the protein gel microstructure.Citation[27– Citation29] Besides, a more strengthened gel is harder and retains more water than those with more open matrixes.Citation[30] In this context, a characterization of protein isolate and gels (including physicochemical, microstructural, and rheological properties), prepared from a by-product of the crayfish industry having a marginal value would contribute to assess its potentials in the production of value-added protein matrices, such as surimi-like gel products. Thus, the objective of this work was to study the gelation ability of a crayfish protein isolate (CFPI) as well as to evaluate the linear viscoelastic properties and microstructure of gels prepared at different protein concentrations.
MATERIALS AND METHODS
Materials
Crayfish flour (CF) was manufactured at pilot-plant scale by ALFOCAN S.A. (Isla Mayor, Seville, Spain). Crayfish meat was separated from the exoskeleton and comminuted to form meat slurry that was dried at 150–160°C in a rotatory drum dryer, to obtain a low moisture crayfish powder. The flour supplied by ALFOCAN S.A. consisted of 64 wt% protein, 18 wt% lipids, 13 wt% ashes, and 5 wt% moisture. All general chemicals used were of analytical grade and were purchased from Sigma Chemical Company (St. Louis, MO, USA). Distilled water was used for the preparation of all solutions.
Preparation of Crayfish Protein Isolate
Crayfish protein isolate (CFPI) was prepared from CF by solvent extraction of lipids, alkaline extraction of soluble proteins and isoelectric precipitation.Citation[10] CF powder was sieved by using a 600-μm mesh and then was defatted by percolation and maceration process during two days with hexane at 30°C. Flour was air-dried and stored at 4°C until use. Defatted flour was dispersed in water (100 g L−1) giving rise to pH 10.5 with 250 g kg−1 NaOH. The dispersion was stirred at room temperature for 4 h and centrifuged at 900× g for 25 min at 4°C in a RC5C Sorvall centrifuge (Sorvall Instruments, Wilmington, DE, USA). The supernatant was then adjusted with 6N HCl to pH 3.4, which corresponds to the isoelectric point (pI) of crayfish protein system,Citation[31] and centrifuged at 9000× g for 10 min at 4°C. The pellet was washed and resuspended with distilled water. The protein dispersion was freeze-dried in a Freeze Mivile 3 (VIRTIS, Warminster, PA, USA).
Chemical Composition of CF Protein Isolate
The protein content was determined in quadruplicate as %N × 6.25 using a LECO CHNS-932 nitrogen micro analyser (Leco Corporation, St. Joseph, MI, USA).Citation[32] Lipid content was analysed by Soxhlet extraction. Moisture and ash content of the isolate was determined in quadruplicate by AOAC 1995 approved methods.Citation[33]
Gelation of CFPI Dispersions
Aqueous dispersions of CFPI (100 g kg−1) at different pH values, from pH 2 to 11, were prepared using different buffer solutions. These dispersions were placed in glass tubes with tightly closed stoppers. Gelation was performed by heating the glass tubes in a water bath at 90°C for 30 min.Citation[34] The tubes were cooled immediately in a water bath at 15°C. Gel samples were kept at 4°C for 24–48 h before analysisCitation[35] with the exception of gels used for studying the setting process. These gels were the gels obtained after heating in a thermostatic bath (AHB).
Viscoelasticity Measurements of Gels
Dynamic viscoelasticity measurements were performed in a controlled-strain rheometer (model ARES) from TA Instruments (New Castle, DE, USA). Strain sweep tests were performed in order to establish the linear viscoelasticity range. All the dynamic viscoelasticity frequency sweep measurements (0.02–100 rad·s−1) were carried out at a strain clearly lower than the critical value for linear viscoelasticity. The geometry used consisted of two 25-mm diameter serrated plates made of aluminium, using a gap between plates of 1 mm. Rheological tests were carried out at 20°C. Gels (AHB) were cut with the proper size with the same diameter of that belonging to the plate geometry (25 mm). All gels studied were subjected to the same thermorheological history (30 min at room temperature) before performing any rheological tests.
On the other hand, gels formed with dispersions after heating in situ in the rheometer (AHR) were performed with three different steps: (i) The first step consisted of a temperature ramp carried out at a constant heating rate (1.5°C/min) from 20 to 90°C. (ii) After the first step, a sudden decrease (10°C/min) in temperature from 90 to 20°C was performed. (iii) Finally, an equilibration stage at the end of the temperature cycle was carried out. To ensure a correct strain control, strain sweep tests were previously performed for different regions and samples to keep measurements within the linear response regime.
Water-Holding Capacity of Gels
Each gel (0.3 to 1.3 g) was equilibrated at room temperature and placed on a nylon plain membrane (5.0-mm pores, Micronsep, New York, NY, USA) maintained in the middle position of a centrifuge tube. Water loss was determined by weighing before and after centrifugation at 120× g for 5 min at 15°C.Citation[36] Water-holding capacity (WHC) was expressed as the percentage of the initial water remaining in the gel after centrifugation. Each value is the mean (standard deviation) of at least four determinations.
Microstructure of Gels
Scanning electron microscopy (SEM) was used in collaboration with the Microscopy Service (CITIUS, Universidad de Sevilla, Sevilla, Spain), to evaluate the microstructure of the gels formed, following the same procedure used in a previous work for heat-set egg yolk gels.Citation[37] Gel samples were immersed in 30 g kg−1 glutaraldehyde for 72 h and washed several times with distilled water and then post-fixed in 10 g kg−1 osmium tetroxide at 4°C. SEM samples were rinsed for 1 h in distilled water before being dehydrated in a grade of ethanol series, 50, 70, 90, and 3 × 100 vol% and dried at the critical point. Each dried sample was mounted on a bronze stub and coated with gold, the specimens being observed with a Philips XL-30 scanning electron microscope (Eindhoven, the Netherlands).
Statistical Analysis
At least three replicates of each measurement were carried out. Statistical analyses were performed using t-test and one-way analysis of variance (ANOVA, p < 0.05) by means of the statistical package SPSS 18 (IBM, Arkmon, NY, USA). Standard deviations from some selected parameters were calculated.
RESULTS AND DISCUSSION
Protein Composition of CFPI
Chemical composition of both crayfish flour (CF) and protein isolate (CFPI) was reported in a previous work.Citation[10] The average composition of CF was 641.5 g·kg−1 protein, 188.6 g·kg−1 lipids, 134.3 g·kg−1 ashes, and 35.6 g·kg−1 moisture, while the CFPI, obtained according to the above described isolation procedure, consisted of 906.1 ± 21.4 g·kg−1 protein, 9.6 ± 0.8 g·kg−1 lipids, 39.8 ± 2.4 g·kg−1 ashes, and 44.5 ± 12.1 g·kg−1 moisture. On the other hand, electrophoresis showed that CFPI was mainly constituted by actin, myosin, and high molecular weight aggregates.Citation[11]
Setting of CFPI Gels
CFPI gels were prepared at two different pH values (pH 2 and 6) and 100 g kg−1 concentration. Gels were stored at 5°C and linear viscoelasticity properties of gels (AHB) were evaluated after different cooling periods: 1, 4, 6, and 24 h. These measurements were carried out at a strain lower than the critical value to ensure that all the results were obtained within the linear response regime. Therefore, strain sweep tests were performed in order to establish the linear viscoelasticity range. The other measurements in the article were always performed at least 24 h after preparation.
shows frequency sweeps for CFPI gels at 100 g kg−1 obtained by treatment in bath (AHB) at pH 2 (a) and pH 6 (b) for different storage time. CFPI systems after heat treatment generally showed a gel-like behaviour, where G′ was much higher than G″, within the whole experimental frequency range, and the slopes of both viscoelasticity functions vs. frequency were moderate (<0.16). This behaviour was very similar to that found for the gels prepared in situ (AHR),Citation[10] being typical of well developed network structures. Moreover, irrespectively of time, the gel obtained at pH 2 showed higher values of viscoelastic moduli due to the high degree of protein cross-linking.Citation[11]
Figure 1 Storage (G’) and loss (G”) moduli of dispersions of CFPI gels at 100 g kg1 as a function frequency and storage time at pH 2 (a) and 6 (b).
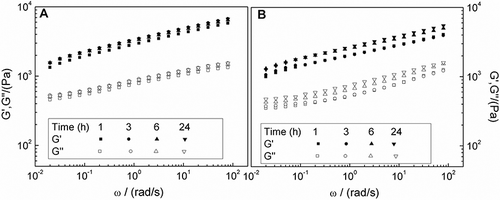
The mechanical spectrum profile was not modified along the cooling time. However, an increase in G′ and G″ during the first stage of cooling (between 3 and 6 h) was observed, particularly at pH 6. illustrates this evolution more clearly by plotting the storage modulus at 1 rad/s, G′1, as a function of the storage time. A slight increase in the G′1 parameter may be noticed until an equilibrium value was reached approximately after cooling for 6 h. This effect was more evident at pH 6. Anyway, these results confirm the need of a cooling period at low temperature before application of any test, to allow full development of physical interactions, which contributes to a reinforcement of protein network. This phenomenon is known as “setting,”Citation[38, Citation39] which, according to these results, led to completely develop the CFPI gel matrix within a cooling period of 24 h.
Viscoelasticity Properties of CFPI Gels
A rheological characterization of CFPI dispersions and heat-induced gels as a function of concentration was studied in a previous paper. In that paper,Citation[12] heat treatment was performed in situ, with a peltier unit connected to the plate-plate sensor system of the rheometer. Moreover, it is worth mentioning the relevance of pH in the gelation process. In this study, gels at pH 6 were selected because this pH brought about maximum rigidity in this system,Citation[11] being coincident with the results found by other authors for heat-set myosin gels at pH 6.Citation[40, Citation41]
The mechanical spectra obtained for CFPI gels prepared at pH 6 in the thermostatic bath are plotted as a function of protein concentration (). As expected, a gel-like behaviour was found for all the concentrations studied. A remarkable strengthening of the gel-like network by thermal processing may be deduced after comparing these results to those previously obtained for CFPI dispersions.Citation[10] A similar effect has been recently published for actomyosin from green mussel.Citation[42] In addition, an increase in concentration leads to a significant evolution towards higher values of G′ and G″ in the experimental frequency range.
Figure 3 Storage (G’) and loss (G”) moduli of dispersions of CFPI gels at pH 6 at different protein concentration obtained after heating on a thermostatic bath (AHB).
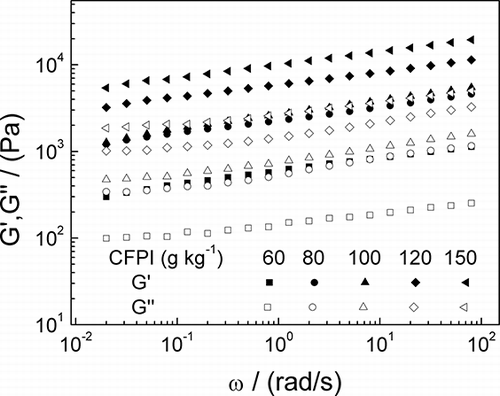
The evolution of the elastic (G′1) and viscous (G″1) moduli at 1 rad/s was plotted as a function of concentration (), for CFPI cold-set gels prepared after heating in the thermostatic bath (AHB), heating in situ in the rheometer (AHR), and for CFPI dispersion before heating (BH). Both dispersions and gels show a continuous increase with the CFPI concentration with a tendency towards asymptotic values. However, the effect of protein concentration is more evident in the case of unprocessed dispersions. The tendency of CFPI dispersions to exhibit remarkable gelation properties even before the application of heat may be related to molecular changes brought about by the drum-drying of protein concentrate used as the raw material for the isolation process. A similar CFPI concentration profile can be observed for gels subjected to the two heat treatments, although the bath treatment seems more efficient, always leading to higher values in G′1 and G″1. This difference may be attributed to the effect of “setting,” which causes a further strengthening of the gel for AHB samples.
Water-Holding Capacity (WHC) of CFPI Gels
It was previously proved ( and ) that viscoelasticity of CFPI gel is a concentration-dependent phenomenon. Elasticity of a protein gel is directly related with the type of matrix formed, which is dependent of the nature and number of interactions established between protein molecules. Consequently, macroscopic properties of gels, as WHC, will be influenced by the balance between protein-protein and protein-water interactions in the gel and the amount of these interactions. WHC data for CFPI, as a function of protein concentration, can be observed in . The higher values of WHC were obtained for the most concentrated systems, which also presented the highest values of G′1 (). Thus, a significant increase in WHC took place by increasing the CFPI concentration from 60 to 80 g kg−1. Moreover, above this concentration CFPI gels always displayed WHC values higher than 80%, although the increase in WHC was not so pronounced because the protein network is completely formed. The poor results obtained at 60 g kg−1 may be related to a weak protein network, where the lower proportion of protein-protein interactions is not enough to constitute a matrix that retains large amounts of water. Similar results of WHC (>60%) were found in other vegetable proteins.Citation[33, Citation43] As for gels from animal protein sources, WHC values ranging from 60 to 80% were reported.Citation[43– Citation45]
Microstructure of CFPI Gels
SEM micrographs of heat-induced CFPI gels (AHB) obtained at pH 6 at 100 and 150 g kg−1 () show an extended cross-linked network although bead-like protein regions can be also observed. Results showing similar structures for thermally induced gels of myosin and myosin sub-fragments were also reported.[40,41] Microstructure appears completely formed at both protein concentrations that did not yield marked differences, being consistent with the results obtained for linear viscoelastic properties and WHC measurements. However, gel at 150 g kg−1 shows a more evident excess of proteins that are not included in the structure.
CONCLUSIONS
Dynamic viscoelastic measurements revealed the ability of CFPI to form network structures under thermal processing, which led to a remarkable enhancement in gel strength. These measurements also proved the ability to detect further reinforcement phenomena, such as cold-setting process. In addition, this gel network microstructure for CFPI systems was also reinforced (either before or after thermal processing) by increasing CFPI concentration. Thus, the most concentrated gel showed a closer structure and, as a consequence, higher water holding capacity and viscoelastic properties were obtained. Both thermal treatments carried out in situ or by using a thermal bath gave rise to similar results of linear viscoelastic properties and, consequently, gel strength. However, some differences took place that may be attributed to slight disparities in the thermal history (as the thermal cycles applied are similar but not coincident), to differences in the geometry of the gelation system, and, above all, to the effect of the cold-setting process. This fact may involve a remarkable practical consequence since it opens the possibility to carry out scaling-up from the results obtained by thermal gelation in a rheometer, provided that the effect of setting was previously established. According to the results obtained, crayfish protein isolate prepared as a by-product from the crayfish industry showed excellent gelling behaviour at different protein concentrations. This fact confirmed the potential of red crayfish in the production of surimi-like gel products.
ACKNOWLEDGMENTS
The authors acknowledge the financial support from the MEC (FPU grant). This work was also part of a research project No. AGL2007-65709 supported by the Spanish MCYT and FEDER and No. 10-TEP-6134 supported by the CICE (Andalousian Government, Spain).
REFERENCES
- Gutierrez-Yurrita , P.J. and Montes , C. 1998 . Enviromental factors controlling crayfish Procambarus clarkia activity in the Doñana National Park freshwater marsh (SW-Spain) . Comparative Biochemistry and Physiology , 120 : 713 – 721 .
- Westman , K. and Westman , P. 1992 . Present status of crayfish management in Europe . Finnish Fish Research , 14 : 1 – 22 .
- Anastacio , P. and Marques , J-C. 1995 . Population biology and production of the red swamp crayfish Procambarus clarkii (Girand) in the lower Modego River Valley, Portugal . Journal of Crustacean Biology , 15 ( 1 ) : 156 – 168 .
- Cremades , O. , Parrado , J. , Alvarez-Ossorio , M.C. , Jover , M. , Collantes de Teran , L. , Gutierrez , J.F. and Bautista , J. 2003 . Isolation and characterization of carotenoproteins from crayfish (Procambarus clarkii) . Food Chemistry , 82 : 559 – 566 .
- Guerin , M. , Huntley , M.E. and Olaizola , M. 2003 . Haematococcus astaxanthin: Applications for human health and nutrition . Trends in Biotechnology , 21 : 210 – 216 .
- Mori , T.A. and Beilin , L.J. 2001 . Long-chain omega 3 fatty acids, blood lipids and cardiovascular risk reduction . Current Opinion in Lipidology , 12 : 11 – 17 .
- Cremades , O. 2004 . Caracterización y producción de carotenoproteínas de P. Clarkii , Sevilla , , Spain : PhD Thesis, Universidad de Sevilla .
- Romero , A. , Bengoechea , C. , Cordobes , F. and Guerrero , A. 2009 . Application of thermal treatments to enhance gel strength and stability of highly concentrated crayfish-based emulsions . Food Hydrocolloids , 23 : 2346 – 2353 .
- Romero , A. , Cordobes , F. and Guerrero , A. 2009 . Influence of pH on linear viscoelasticity and droplet size distribution of highly concentrated O/W crayfish flour-based emulsions . Food Hydrocolloids , 23 : 244 – 252 .
- Romero , A. , Cordobes , F. , Puppo , M.C. , Villanueva , A. , Pedroche , J. and Guerrero , A. 2009 . Linear viscoelasticity and microstructure of heat-induced crayfish protein isolate gels . Food Hydrocolloids , 23 : 964 – 972 .
- Romero , A. , Cordobes , F. , Guerrero , A. and Puppo , M.C. 2011 . Crayfish protein isolated gels. A study of pH Influence . Food Hydrocolloids , 25 : 1490 – 1498 .
- Park , J.W. 2005 . “ Surimi Seafood: Products, Market and Manufacturing ” . In Surimi and Surimi Seafood , Edited by: Park , J.W. Boca Raton , FL : Taylor and Francis .
- Suzuki , T. 1981 . Fish and Processing Technology , London , , UK : Applied Science Publishers .
- Damodaran , S. 1997 . “ Food proteins: An overview ” . In Food Proteins and Their Applications , Edited by: Damodaran , S. and Paraf , A. 1 – 24 . New York : Marcel Decker .
- Chan , J.K. , Gill , T.A. and Paulson , A.T. 1992 . The dynamics of thermal denaturation of fish myosins . Food Research International , 25 : 117 – 123 .
- Clark , A.H. 1998 . “ Gelation of globular proteins ” . In Functional Properties of Food Macromolecules , Edited by: Hill , S.E. , Ledward , D.A. and Mitchell , J.R. 77 – 142 . Gaithersburg , MD : Aspen Publisher .
- Dickinson , E. and Hong , S.T. 1995 . Influence of water-soluble nonionic emulsifier on the rheology of heat-set protein stabilized emulsion gels . Journal of Agricultural and Food Chemistry , 43 : 2560 – 2566 .
- Miroshinichenko , N.S. , Balanuk , I.V. and Nozdrenko , D.N. 2000 . Packing of myosin molecules in muscle thick filaments . Cell Biology International , 24 ( 6 ) : 327 – 333 .
- Clark , A.H. 1998 . “ Gelation of globular proteins ” . In Functional Properties of Food Macromolecules , Edited by: Hill , S.E. , Ledward , D.A. and Mitchell , J.R. 77 – 142 . Gaithersburg , MD : Aspen Publishers .
- Lanier , T.C. , Carvajal , P. and Yongsawatdigul , J. 2005 . “ Surimi gelation chemistry ” . In Surimi and Surimi Seafood , Edited by: Park , J. Boca Raton , FL : Taylor and Francis .
- Gilleland , G.M. , Lanier , T.C. and Hamann , D.D. 1997 . Covalent bonding in pressure-induced fish protein gels . Journal of Food Science , 62 ( 4 ) : 713 – 716 .
- Sánchez , C. and Burgos , J. 1997 . Gelation of sunflower globulin hydrolysates: Rheological and calorimetric studies . Journal of Agricultural and Food Chemistry , 45 : 2407 – 2412 .
- Rawdkuen , S. , Benjakul , S. , Visessanguan , W. and Lanier , T.C. 2008 . Rheological and textural properties of Pacific Whiting surimi gels as influenced by chicken plasma . International Journal of Food Properties , 11 : 820 – 832 .
- Campo-Deaño , L. , Tovar , C.A. , Pombo , M.J. , Solas , M.T. and Borderías , A.J. 2009 . Rheological study of giant squid surimi (Dosidicus gigas) made by two methods with different cryoprotectants added . Journal of Food Engineering , 94 : 26 – 33 .
- Beveridge , T. , Jones , L. and Tung , M.A. 1984 . Progel and gel formation and reversibility of gelation of whey, soybean, and albumen protein gels . Journal of Agricultural and Food Chemistry , 32 : 307 – 313 .
- Burey , P. , Bhandari , B.R. , Rutgers , R.P.G. , Halley , P.J. and Torley , P.J. 2009 . Confectionery gels: A review on formulation, rheological and structural aspects . International Journal of Food Properties , 12 : 176 – 210 .
- Puppo , M.C. and Añon , M.C. 1998 . Structural properties of heat-induced soy proteins gels as affected by ionic strength and pH . Journal of Agricultural and Food Chemistry , 46 : 3583 – 3589 .
- Sun , S. , Song , Y. and Zheng , Q. 2009 . Rheological behavior of heat-induced wheat gliadin gel . Food Hydrocolloids , 23 : 1054 – 1056 .
- Ko , S. and Gunasekaran , S. 2009 . In situ microstructure evaluation during gelation of β-lactoglobulin . Journal of Food Engineering , 90 : 161 – 170 .
- Foegeding , E.A. , Bowland , E.L. and Hardin , C.C. 1995 . Factors that determine the fracture properties and microstructure of globular protein gels . Food Hydrocolloids , 9 : 237 – 249 .
- Romero , A. , Cordobés , F. , Puppo , M.C. , Guerrero , A. and Bengoechea , C. 2008 . Rheology and droplet size distribution of emulsions stabilized by crayfish flour . Food Hydrocolloids , 22 : 1033 – 1043 .
- Etheridge , R.D. , Pesti , G.M. and Foster , E.H. 1998 . A comparison of nitrogen values obtained utilizing the Kjeldahl nitrogen and Dumas combustion methodologies (Leco CNS 2000) on samples typical of an animal nutrition analytical laboratory . Animal Feed Science and Technology , 73 : 21 – 28 .
- AOAC . 1995 . Official Methods of Analysis , 15th , Arlington , VA : Association of Official Analytical Chemists . Arlington
- Damodaran , S. 1988 . Refolding of thermally unfolded soy proteins during the cooling regime of the gelation process: effect on gelation . Journal of Agricultural and Food Chemistry , 36 : 262 – 269 .
- Puppo , M.C. , Lupano , C.E. and Añon , M.C. 1995 . Gelation of soybean protein isolate in acidic conditions. Effect of pH and protein concentration . Journal of Agricultural and Food Chemistry , 43 : 2356 – 2361 .
- Queguiner , C. , Dumay , E. , Cavalier , C. and Cheftel , J.C. 1989 . Reduction of Streptococcus themolphilus in a whey protein isolate by low moisture extrusion cooking without loss of functional properties . International Journal of Food Science and Technology , 24 : 601 – 612 .
- Cordobes , F. , Partal , P. and Guerrero , A. 2004 . Rheology and microstructure of heat-induced egg yolk gels . Rheologica Acta , 43 : 184 – 195 .
- Numakura , T. , Seki , N. , Kimura , T. , Toyoda , K. , Fujita , T. , Takama , K. and Arai , K. 1985 . Cross-linking reaction of myosin in fish paste Turing setting (suwari) . Nippon Suisan Gakkaishi , 51 : 1559 – 1565 .
- Kim , B.Y. 1987 . Rheological investigation of gel structure formation by fish proteins during setting and heat processing , Raleigh , NC : Ph.D. Thesis, North Caroline State University .
- Ishioroshi , M. , Samejima , K. , Aire , Y. and Yasui , T. 1980 . Heat induced gelling properties of actomyosin: Effect of blocking the myosin-actin interaction in heat-induced gelation of myosin in the presence of actin . Agricultural and Biological Chemistry , 44 : 2185 – 2194 .
- Samejima , K. , Ishioroshi , M. and Yasui , T. 1981 . Relative roles of the head and tail portions of the molecule in heat-induced gelation of myosin . Journal of Food Science , 46 : 1412 – 1418 .
- Binsi , P.K. , Shamasundar , B.A. and Dileep , A.O. 2006 . Some physico-chemical, functional and rheological properties of actomyosin from green mussel (Perna viridis) . Food Research International , 39 : 992 – 1001 .
- Alvarez , C. and Tejada , M. 1997 . Influence of texture of suwari gel son kamaboko gels made from sardine (Sardina pilchardus) surimi . Journal of the Science and Food Agriculture , 75 ( 4 ) : 472 – 480 .
- Jin , S.K. , Kim , I.S. , Kim , S.J. , Jeong , K.J. , Choi , Y.J. and Hur , S.J. 2007 . Effect of muscle type and washing times on physico-chemical characteristics and qualities of surimi . Journal of Food Engineering , 81 : 618 – 623 .
- Baxter , S.R. and Skonberg , D.I. 2008 . Gelation properties of previously cooked minced meat from Jonah crab (Cancer borealis) as affected by washing treatment and salt concentration . Food Chemistry , 109 : 332 – 339 .