Abstract
Complex liposomes containing both a hydrophilic drug vitamin C and hydrophobic drug medium-chain fatty acids were prepared by double emulsion method and double emulsion-dynamic high pressure microfluidization, respectively. The results showed that the complex nanoliposomes (medium-chain fatty acids-vitamin C nanoliposomes) prepared by double emulsion-dynamic high pressure microfluidization exhibited higher entrapment efficiency of medium-chain fatty acids (48.66 ± 2.59)%, relatively higher entrapment efficiency of vitamin C (64.00 ± 5.27)%, lower average size diameter (92.8 ± 6.85) nm, and better storage stability at 4°C for 90 days than those prepared by double emulsion. In vitro drug release studies of medium-chain fatty acids-vitamin C nanoliposomes prepared by double emulsion-dynamic high pressure microfluidization were also investigated. Prolonged drug releases of medium-chain fatty acids-vitamin C nanoliposomes were observed compared with liposomes encapsulating one drug (medium-chain fatty acids or vitamin C) over a period of 24 h. It was indicated that double emulsion-dynamic high pressure microfluidization could be a potential approach in the preparation of nanoscale complex liposomes encapsulating both a hydrophilic drug and hydrophobic drug.
INTRODUCTION
Medium-chain fatty acids (MCFAs) are composed of octanoic acid (an 8-carbon-medium-chain fatty acid) and decanoic acid (a 10-carbon-medium-chain fatty acid).[Citation1] In animals’ bodies, MCFAs are absorbed in the portal vein system, compared to the long-chain fatty acids that are absorbed in the lymphatic system. It has been known that MCFAs in triglycerides are more rapidly hydrolyzed by pancreatic lipase than long-chain fatty acids.[Citation2,Citation3] Carnitine in seriously ill patients may already be deficient, so MCFAs could be used as an energy source for those patients. Besides, they can save the amount of protein,[Citation1] inhibit the formation of body fat, and suppress diseases caused by pathogenic bacteria.[Citation4,Citation5] However, the water solubility of MCFAs is relatively weak, which often prevents their parenteral and oral administration from being effective. In addition, an excess of non-esterified MCFAs may have serious side effects, such as stimulation to the secretion of cholecystokinin and perhaps other intestinal hormones.[Citation6] Vitamin C (Vit.C), an essential nutrient for humans and other certain animal species, is widely used in skincare and skin lightener cosmetics.[Citation7] It could reduce the damage of free radicals on the skin, delay aging, and reduce the formation of melanin.[Citation8] However, Vit.C is unstable and could be easily oxidized in the presence of oxygen, humidity, high temperatures, and heavy metals during process and storage, which could cause the decrease of its utilization.[Citation9]
In order to overcome their shortcomings and enhance their bioavailabilities, liposomes could be applied as a delivery system. They could prolong the residence time of the drugs in blood, releasing the drugs slowly in vivo.[Citation10] The phospholipid bilayer membrane structure of liposomes guarantees that both hydrophilic and hydrophobic drugs could be efficiently entrapped in it simultaneously.[Citation11] Due to better distributions in the organisms, small liposomes (nanoliposomes) with diameters of about 100 nm are frequently used as a delivery system for drugs and nutrition.[Citation12] Nanoliposomes have similar physical, structural, and thermodynamic properties as conventional liposomes.[Citation13] Some studies suggest that the bioavailability of encapsulated non-polar components is higher in nanoliposomes than conventional liposomes because of small particle size and high surface-to-volume ratio.[Citation14,Citation15] In our previous studies, MCFAs liposomes were prepared by several different methods, such as film ultrasonic dispersion, modified ethanol injection, and reverse phase evaporation. MCFAs liposomes prepared by thin film ultrasonic dispersion had a high entrapment efficiency of 82.7% and a good distribution in size diameters.[Citation16] The properties of easy-energy-supply of MCFAs nanoliposomes obtained by dynamic high pressure microfluidization were also investigated.[Citation17] In addition, vitamin C nanoliposomes were prepared by film evaporation-dynamic high pressure microfluidization.[Citation18] At present, there is still no report regarding the preparation of MCFAs-Vit.C nanoscale complex liposomes. Complex liposomes and nanoliposomes are commonly discussed topics. Preparation of nanoscale complex liposomes is very challenging by traditional methods, due to the difficulties in the implementation of small particle size and high drug entrapment efficiency.
In most studies reported, only one drug was entrapped within controlled release liposomes at a time. In some studies, encapsulation of hydrophobic drugs was found to be higher than hydrophilic drugs.[Citation19,Citation20] Yet little knowledge is available on the co-encapsulation of both hydrophobic and hydrophilic drugs, especially if they exhibit significantly different solubility behaviors. Hombreiro-Pérez and co-workers[Citation21] investigated the preparation and characterization of nifedipine and propranolol HCl microparticles prepared by the o/w and w/o/w solvent evaporation methods. Hammady and co-workers[Citation22] used water-oil-water (w/o/w) double emulsion solvent evaporation method to co-encapsulate all-trans retinoic acid and calf thymus DNA.
A variety of methods have been employed to prepare liposomes, such as freeze-thaw, thin layer evaporation, film ultrasonic dispersion, modified ethanol injection, and reverse phase evaporation (RPE).[Citation23,Citation24] Most of these methods show favorable characteristics when preparing liposomes entrapping one hydrophobic or hydrophilic drug. It will probably have some disadvantages for preparing liposomes that entrap both hydrophobic and hydrophilic drugs. There are many problems influencing the manufacture and development of liposomes, such as stability issues, batch to batch reproducibility, low drug entrapment, and particle size control.[Citation25] Combined methods, which consist of two or more different preparation methods, are needed for the preparation of liposomes that entrap both hydrophobic and hydrophilic drugs.
Many authors have previously shown that double emulsion method (DE) was the most appropriate method to encapsulate hydrophilic drugs within microparticles.[Citation26,Citation27] Dynamic high pressure microfluidization (DHPM) is a technology that uses the combined forces of shear, cavitation, and ultra-high pressures up to 200 MPa.[Citation28] It could reduce the particle sizes of liposomes greatly[Citation29] and could be applied for large-scale production of liposomes in a continuous process[Citation30] without employing toxic solvents.[Citation31,Citation32] Various liposomes have been prepared by DHPM, such as MCFAs,[Citation17] DC-Cho cationic,[Citation33] and hemoglobin liposomes.[Citation34]
In this study, combined methods (DE-DHPM) were used for preparing MCFAs-Vit.C nanoscale complex liposomes in order to provide theoretical guiding for the preparation of nanoscale complex liposomes entrapping other hydrophilic drugs and hydrophobic drugs. The comparison of MCFAs-Vit.C complex liposomes prepared by DE-DHPM and DE under the optimal conditions was studied. Physicochemical characterizations (particle size, drug entrapment efficiency, morphology, in vitro drug release, and storage stability) of MCFAs-Vit.C nanoscale complex liposomes prepared by DE-DHPM were also investigated.
MATERIALS AND METHODS
Materials
Medium-chain fatty acids were kindly provided by a USA company (UPMC, Pittsburgh, Pennsylvania, USA). Octanoic acid methyl ester and decanoic acid methyl ester were purchased from AccuStandard (New Haven, USA). Vitamin C was purchased from Sinopharm Chemical Reagent Co. Ltd. (Shanghai, China). Soybean phosphatidylcholine (SPC) was provided by Merya’s Lecithin Co. Ltd. (Beijing, China). Cholesterol (CHO) was obtained from Tianjin Damao Chemical Reagent Co. Ltd. (Tianjin, China). N-Hexane and methanol were of chromatographic grade. Acetic acid, EDTA, fast blue salt B, and other reagent chemicals were all of analytical grade.
Preparation of MCFAs-Vit.C Complex Liposomes by DE
Referring to the method of Hombreiro Pérez and co-workers,[Citation35] MCFAs-Vit.C complex liposomes were prepared by double emulsion method (DE) under the optimum conditions as follows: a 100:25:4 mass ratio of soybean phospholipids (1200 mg), cholesterol (300 mg), and vitamin E (48 mg) were dissolved in 15 mL of absolute ethanol containing MCFAs (300 mg), followed by an injection of 2 mL twice-distilled water under vigorous stirring at a temperature of 50°C. The primary emulsion was placed in a rotary evaporator (RE52-02, Shanghai Yarong Instrument Co., China) to remove part of the solvent under reduced pressure for 10 min. Then, 25 mL of twice-distilled water containing 450 mg surfactant (tween-80) and 60 mg Vit.C was added at this point. The resulting emulsion was constantly agitated for about 20 min at 50°C, and any traces of the solvent were removed by rotary evaporation under reduced pressure at 50°C for about 1 h until it formed a milk-like liquid, resulting in the formation of complex liposomes.
Preparation of MCFAs-Vit.C Complex Liposomes by DE-DHPM
MCFAs-Vit.C complex nanoliposomes were prepared by double emulsion-dynamic high pressure microfluidization (DE-DHPM) under the optimum conditions. The crude liposomes were made following the same procedure and composition as MCFAs-Vit.C complex liposomes obtained by double emulsion method (DE) as mentioned above. To prepare nanoscale complex liposomes, the crude liposomes were further treated in a microfluidizer (M-110EH30, Microfluidic Corporation, Newton, MA, USA) under optimum treatment condition (120 MPa, three passes) at room temperature. Finally, MCFAs-Vit.C nanoscale complex liposomes were obtained.
Determination of Entrapment Efficiency (EE) of MCFAs
According to the methods of our previous studies[Citation16,Citation17] after minor modifications, entrapment efficiency (EE) of MCFAs was determined. About 1 mL of MCFAs-Vit.C complex liposomes was centrifuged at 12000× g for 20 min, and then 2 mL of n-hexane was added to withdraw unentrapped MCFAs. The rest of the suspension was mixed with 7 mL methanol and then sonicated for 25 min to demulsify the complex liposomes. The fatty acid methyl esters (FAME) were made by direct transesterification.[Citation36,Citation37] FAMEs were measured by gas chromatography (Agilent 6890 Series GC System, Aglient Technologies, Santa Clara, CA, USA) with a flame ionization detector. The chromatographic column was HP-innowax polyethylene glycol (30 m × 0.32 mm × 0.5 μm) and the flow rates of various gases were nitrogen, 20 mL/min; hydrogen, 40 mL/min; air, 450 mL/min. The temperatures of injection port and detector were maintained at 280°C. The oven temperature was programmed to maintain at 150°C for 5 min, then rise to 180°C at a rate of 6°C/min and stay at 180°C for 2 min. The injection volume was 1 μl. Peak areas and retention times were calculated and FAMEs were identified by comparing retention times to the standard octanoic acid methyl ester and decanoic acid methyl ester. The entrapment efficiency of MCFAs (EEMCFAs) was calculated from Eq. (1):
Determination of Entrapment Efficiency (EE) of Vit.C
Non-encapsulated Vit.C was separated from MCFAs-Vit.C complex liposomes by centrifugation. About 5 mL of liposomes was centrifuged at 12000× g for 20 min. According to some previous reports after some modifications,[Citation38−Citation40] the drug content in the supernatant was quantified by UV-Visible spectrophotometry. Then, 1 mL of above treated mixture solution was placed in a 10-mL colorimetric tube followed by adding 0.3 mL of EDTA (0.25 M), 0.5 mL of acetic acid (0.5 M), and 1.25 mL of fast blue salt B (2 g/L) in sequence, then diluted to 10 mL with deionized water. After 20 min the above mixture was determined spectrophotometrically at 420 nm using a UV-visible spectrophotometer (T6, Purkinje General, Peking, China). The entrapment efficiency of Vit.C (EEVit.C) was calculated from Eq. (2):
Characteristics of MCFAs-Vit.C Complex Liposomes
Particle size and size distribution
The particle sizes of MCFAs-Vit.C complex liposomes prepared by DE and DE-DHPM were determined by dynamic laser light scattering method at 25°C using a Nicomp 380 ZLS (Santa Barbara, CA, USA). The intensity was detected at an angle of 90°. MCFAs-Vit.C complex liposomes were diluted with twice-distilled water before measurement.[Citation41−Citation43]
Transmission electron microscopy
MCFAs-Vit.C complex liposomes prepared by DE-DHPM and DE were diluted approximately at 1:10 with twice-distilled water. One drop of the diluted sample was left alone for 3 min. The solution was placed on a copper grid for 5 min before the excess liquid was sipped up by the filter papers,[Citation44,Citation45] and then air-dried at room temperature before being observed under TEM (Hitachi H-600, Japan).
Stability of MCFAs-Vit.C nanoscale complex liposomes
The stability was assessed by comparing different changes in mean diameters and drug encapsulation efficiency of MCFAs-Vit.C nanoscale complex liposomes prepared by DE-DHPM at fixed time intervals (1, 4, 7, 10, 13, 16, 30, 45, 60, 90 days) respectively. The nanoscale complex liposomes were stored under (4°C) shade in a sealed condition.[Citation46]
In vitro drug release studies
Liposomes have the property of prolonging the residence time of the drugs in blood, releasing the drugs slowly in vivo. Preparation of nanoliposomes and complex liposomes with suitable sustained release manner is very challenging. In order to further investigate the sustained release manner of MCFAs-Vit.C nanoscale complex liposomes, MCFAs-Vit.C nanoscale complex liposomes were kept constant at 37 ± 0.5°C and stirred at 150 rpm. MCFAs-Vit.C liposomes, MCFAs liposomes, and Vit.C liposomes were prepared by DE-DHPM, respectively. Non-encapsulated drug was separated from liposomes by centrifugation at 12000× g for 20 min. In vitro drug release[Citation47] from MCFAs-Vit.C nanoliposomes, MCFAs liposomes, and Vit.C liposomes were performed in phosphate buffer pH 7.4 (137 mM NaCl, 17 mM Na2HPO4, 1 mM KH2PO4) with solutions containing Vit.C or MCFAs as the control groups. Then, 10 mL of MCFAs liposomes, Vit.C liposomes, MCFAs-Vit.C nanoliposomes, solutions containing Vit.C, and solutions containing MCFAs were loaded in dialysis bags with the cutoff size of 3500 Da and diameter of 27 mm before being placed into 100-mL twice-distilled water (kept constant at 37 ± 0.5°C and stirred at 150 rpm), respectively. At predetermined time intervals (2, 4, 6, 8, 10, 12, 14, 18, 24 h), 2 mL of samples were withdrawn (replaced with fresh medium), filtered, and analyzed for drug contents (MCFAs and Vit.C content) by gas chromatography and UV-visible spectrophotometry as described above, respectively. Each batch was analyzed in triplicate. The releasing rates of MCFAs (RMCFAs) and Vit.C (Rvit.C) were calculated from Eqs. (3) and (4) separately:
Statistical Analysis
All experiments were done in triplicate unless otherwise specified and the values were expressed as means ± SD. Data were subjected to statistical analysis by Student’s t-test. Differences were considered significant at p < 0.05.
RESULTS AND DISCUSSION
Comparison of Liposomes Prepared by DE and DE-DHPM
There were many factors affecting the particle sizes and entrapment efficiency of liposomes. It was concluded that the preparation methods had a great influence on particle size and drug entrapment efficiency of liposomes. Maitani and coworkers[Citation48] prepared cationic liposomes by the dry-film method with sonication until a homogeneous size distribution ranging from 150 to 230 nm was obtained. Elbayoumi and coworkers[Citation49] used the lipid film hydration method to prepare antinucleosome antibody-modified liposomes, which was extruded through 200 nm and then 100 nm pore size nucleopore polycarbonate membranes (15 times) to obtain uniform vesicles of 130 nm in size. So the comparison of DE and DE-DHPM under the optimal conditions was done in the research. MCFAs-Vit.C complex liposomes prepared by DE and DE-DHPM under their optimal conditions showed relatively satisfactory Vit.C entrapment efficiency with no obvious differences (62.2 ± 3.86% and 64.00 ± 5.27%). MCFAs entrapment efficiency and particle size of MCFAs-Vit.C nanoscale complex liposomes prepared by DE-DHPM were (48.66 ± 2.59%) and (92.8 ± 6.85 nm), while those of complex liposomes prepared by DE were (25.15 ± 2.35%) and (238.7 ± 5.68 nm) respectively. It could be seen that DE-DHPM showed favorable characteristics in preparing MCFAs-Vit.C nanoliposomes, indicating that DHPM had a great effect on the particle sizes and entrapment efficiency of the complex liposomes. Takahashi and coworkers[Citation29] reported that the liposomes prepared by DHPM presented lower particle size of below 130 nm and higher entrapment efficiency than those by a homogenizer (400 nm). In our previous study, MCFAs nanoliposomes had a lower particle size of 76.2 nm and higher entrapment efficiency of 70.5% after DHPM treatment than those made by Bangham method.[Citation17] However, Lagoueyte and coworkers have pointed out the drawbacks of high-pressure microfluidization.[Citation50] The high shearing action of high-pressure microfluidization can potentially damage the structure or function of the encapsulated material. Another disadvantage of the method is the EE reduction, which is caused by the breakdown of liposomes after over treatment.
Characteristics of the Liposomes
Transmission electron microscopy
The morphologies of MCFAs-Vit.C liposomes prepared by DE and DE-DHPM were evaluated by transmission electron microscopy (TEM) experiments. The typical micrographs of the freshly prepared MCFAs-Vit.C liposomes by DE and DE-DHPM are shown in and 2, respectively. Both types of liposomes had spherical shapes. The sizes of both liposomes observed in the typical micrographs were smaller than the results obtained by particle size measurements. This is probably due to hydration and swelling of the particles in aqueous buffer.[Citation30] MCFAs-Vit.C liposomes prepared by DE-DHPM were smaller and more uniform than DE. However, a small part of MCFAs-Vit.C nanoliposomes particles prepared by DE-DHPM were in irregular spherical-shape, possibly due to the complex effects of cavitation, shear and high velocity impact brought by DHPM. In our previous study, the crude MCFAs liposomes prepared by Bangham’s method were predominantly spherical in shape, while MCFAs nanoliposomes prepared by DHPM with much smaller vesicles were in irregular spherical-shape or elliptical membranous structures.[Citation17] In this study, MCFAs-Vit.C nanoliposomes prepared by DE-DHPM were predominantly spherical in shape, indicating that the combined method of DE-DHPM under optimal conditions was successful in preparing MCFAs-Vit.C nanoliposomes with small particle size without destroying their spherical shape.
FIGURE 1 TEM picture of MCFAs-Vit.C complex liposomes by double emulsion. The bar represents 200 nm.
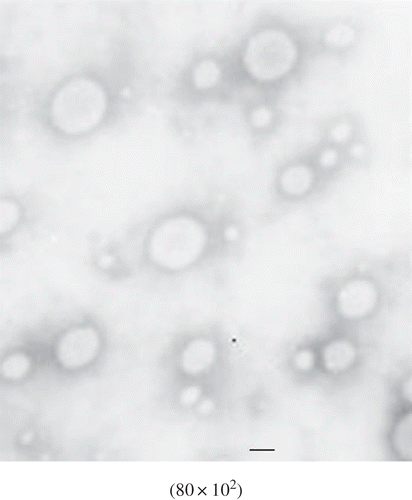
FIGURE 2 TEM picture of MCFAs-Vit.C nanoscale complex liposomes by double emulsion-dynamic high pressure microfluidization. The bar represents 100 nm.
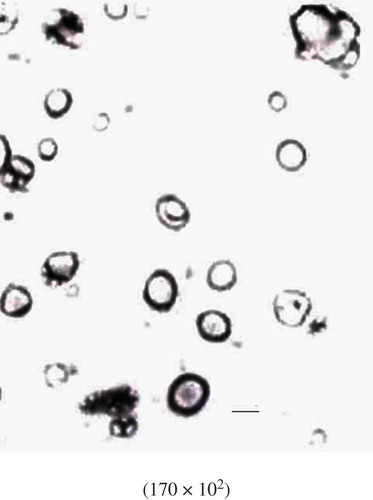
TABLE 1 Physicochemical characteristics of MCFAs-Vit.C complex liposomes at 4°C
Storage Stability
The stability of the freshly prepared MCFAs-Vit.C complex liposomes by DE and DE-DHPM stored under (4°C) shade for a certain time was investigated in our previous experiments. However, MCFAs entrapment efficiency of MCFAs-Vit.C complex liposomes prepared by DE decreased greatly from 25.15 to 10.26%, and Vit.C entrapment efficiency decreased greatly from 62.20 to 32.16% with average size diameter increasing from 238.7 to 385.6 nm after 30 days’ storage. In addition, there was little sedimentation present in MCFAs-Vit.C liposomes suspension by DE, which made MCFAs-Vit.C complex liposomes prepared by DE unsuitable for longer time study of stability. It could be seen from these results that MCFAs-Vit.C nanoscale complex liposomes by DE-DHPM exhibited better stability than those by DE. The long term stability of MCFAs-Vit.C nanoscale complex liposomes by DE-DHPM stored under (4°C) shade for 90 days is shown in . As shown in , the particle size had smaller changes during the storage period of 90 days, which changed from 95.5 ± 5.86 nm to 138.3 ± 5.26 nm. The increment of a 45% in size after 90 days was possibly due to the partial aggregation brought by the minimization of liposomes’ high surface-to-volume ratios. In our previous studies, the stability of MCFAs nanoliposomes prepared by DHPM at 4°C for 3 months was investigated, which presented good stability with small changes in particle size from 86.8 ± 1.01 nm to 172.6 ± 5.51 nm.[Citation17] Besides, MCFAs-Vit.C nanoscale complex liposomes had little loss of entrapped MCFAs and Vit.C during 90 days. These results showed that the MCFAs-Vit.C nanoscale complex liposomes prepared by DE-DHPM had a favorable stability during storage. Interestingly, greater loss of entrapped Vit.C in some degree was observed than MCFAs. This is possibly because MCFAs and Vit.C are located in different compartments of MCFAs-Vit.C nanoscale complex liposomes. As described in the study of Gulati and coworkers,[Citation51] who reported that problems like loss of entrapped drugs on storage are minimal with hydrophobic drugs, and reduction of entrapment efficiency in addition to instability have been found to be associated with the liposomal entrapment of hydrophilic drug other than that of high hydrophobic drug.
In Vitro Drug Release Studies
The in vitro drug release profiles obtained for each formulation are presented in and , by representing the percentage of Vit.C and MCFAs release with respect to the amount of Vit.C and MCFAs encapsulated. As shown in , rapid Vit.C releases were observed in all these groups (MCFAs-Vit.C nanoscale complex liposomes, Vit.C liposomes, and Vit.C solution) from 2 to 10 h. After 12 h, the release of the total amount of Vit.C in MCFAs-Vit.C nanoscale complex liposomes was 31.72 and 55.02% was for Vit.C liposomes, while 99.8% was for Vit.C solutions (almost release completely). Then, they all released slowly without great changes after 14 h. Overall, MCFAs-Vit.C nanoscale complex liposomes exhibited the slowest release rates compared to Vit.C liposomes and the solutions containing Vit.C alone over the period of 24 h (P < 0.05).
FIGURE 3 In vitro drug release of Vit.C from liposomes at 37°C (n = 3, ±SD). □: The percentage of Vit.C release in Vit.C liposomes; ▪: the percentage of Vit.C release in MCFAs-Vit.C nanoscale complex liposomes; ▴: the percentage of Vit.C release in solution containing Vit.C.
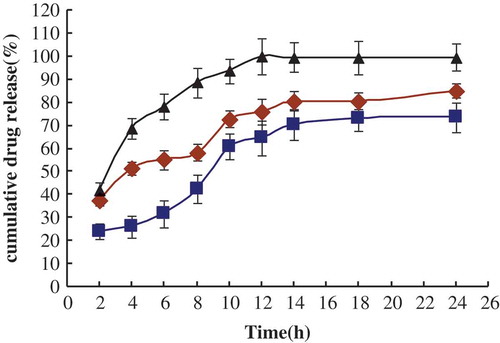
FIGURE 4 In vitro drug release of MCFAs from liposomes at 37°C (n = 3, ±S D). ♦: The percentage of MCFAs release in MCFAs liposomes; ▪: the percentage of MCFAs release in MCFAs-Vit.C nanoscale complex liposomes; ▴: the percentage of MCFAs release in solution containing MCFAs.
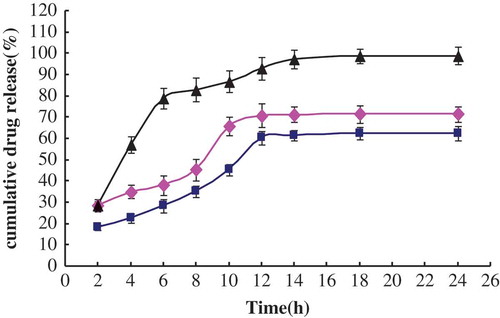
As shown in , MCFAs-Vit.C nanoscale complex liposomes had the slowest release profile compared with MCFAs liposomes and the solution containing MCFAs (P < 0.05). Relatively rapid MCFAs releases were also observed in all these groups from 2 to 10 h, followed by a slower release rate after 10 h, during which MCFAs were released in an approximately linear mode. After 12 h, the release of the total amount of MCFAs in MCFAs-Vit.C nanoscale complex liposomes was just 60.34%, and 70.8% was for MCFAs liposomes. These results were similar to the report of Maestrelli and coworkers,[Citation52] who mentioned that liposomes formulations had slower and more prolonged drug release in comparison to simple drug solutions. Overall, it could be concluded that release of Vit.C and MCFAs from liposomes in co-encapsulated form gave an excellent sustained release profile.
CONCLUSIONS
MCFAs-Vit.C complex liposomes were prepared by double emulsion (DE) and double emulsion-dynamic high pressure microfluidization (DE-DHPM) separately. MCFAs-Vit.C nanoscale complex liposomes prepared by DE-DHPM had a good size distribution, good appearance and relatively high entrapment efficiency of MCFAs and Vit.C. The MCFAs entrapment efficiency of liposomes was 48.66 ± 2.59% with the mean diameter 92.8 ± 6.85 nm, while Vit.C was 64.00 ± 5.27%. MCFAs-Vit.C nanoscale complex liposomes exhibited a favorable stability during the storage period of 90 days at 4°C. The results of in vitro drug release studies revealed that MCFAs-Vit.C nanoscale complex liposomes, exhibiting no obvious difference in release rates of MCFAs liposomes or Vit.C liposomes, had a prolonged drug release compared with Vit.C or MCFAs solutions. In conclusion, DE-DHPM could be a potential approach in preparing nanoscale complex liposomes containing both the hydrophilic drug and hydrophobic drug, offering an inner liposome protection, high drug encapsulation efficiency and a prolonged drug release.
ACKNOWLEDGMENTS
This research is supported by the National Natural Science Foundation of China (21266021), and the research program of State Key Laboratory of Food Science and Technology, Nanchang University (No.SKLF-TS-201115).
REFERENCES
- Jones, P.M.; Butt, Y.M.; Bennett, M.J. Effects of odd-numbered medium-chain fatty acids on the accumulation of long-chain 3-hydroxy-fatty acids in long-chain L-3-hydroxyacyl CoA dehydrogenase and mitochondrial trifunctional protein deficient skin fibroblasts. Molecular Genetics and Metabolism 2004, 81, 96–99.
- Greenberger, N.J.; Skillman, T.G. Medium-chain triglycerides: Physiologic considerations and clinical implications. New England Journal of Medicine 1969, 280, 1045–1058.
- Bach, A.C.; Babayan, V.K. Medium-chain triglycerides: an update. American Journal of Clinical Nutrition 1982, 36, 950–962.
- Hirazawa, N.; Oshima, S.; Hara, T.; Mitsuboshi, T.; Hata, K. Antiparasitic effect of medium-chain fatty acids against the ciliate Cryptocaryon irritans infestation in the red sea bream Pagrus major. Aquaculture 2001, 198, 219–228.
- Wong, P.Y.Y.; Nakamura, S.; Kitts, D.D. Functional and biological activities of casein glycomacropeptide as influenced by lipophilization with medium and long chain fatty acid. Food Chemistry 2006, 97, 310–317.
- Decuypere, J.A.; Dierick, N.A. The combined use of triacylglycerols containing medium-chain fatty acids and exogenous lipolytic enzymes as an alternative to in-feed antibiotics in piglets: Concept, possibilities and limitations. An overview. Nutrition Research Reviews 2003, 16, 193–210.
- Liu, N.; Park, H.J. Factors effect on the loading efficiency of Vitamin C loaded chitosan-coated nanoliposomes. Colloids and Surfaces B–Biointerfaces 2010, 76, 16–19.
- Sablani, S.; Al-Belushi, K.; Al-Marhubi, I.; Al-Belushi, R. Evaluating stability of vitamin C in fortified formula using water activity and glass transition. International Journal of Food Properties 2007, 10, 61–71.
- Zhang, L.; Lerner, S.; Rustrum, W.V.; Hofmann, G.A. Electroporation-mediated topical delivery of vitamin C for cosmetic applications. Bioelectrochemistry and Bioenergetics 1999, 48, 453–461.
- Lee, J.S.; Chung, D.; Lee, H.G. Preparation and characterization of calcium pectinate gel beads entrapping catechin-loaded liposomes. International Journal of Biological Macromolecules2008, 42, 178–184.
- Sánchez, M.; Aranda, F.J.; Teruel, J.A.; Ortiz, A. New pH-sensitive liposomes containing phosphatidylethanolamine and a bacterial dirhamnolipid. Chemistry and Physics of Lipids 2011, 164, 16–23.
- Mozafari, M.R.; Khosravi-Darani. K.; Borazan. G.G.; Cui, J.; Pardakhty, A.; Yurdugul, S. Encapsulation of food ingredients using nanoliposome technology. International Journal of Food Properties 2008, 11, 833–844.
- Mozafari, M.R.; Mortazavi S.M. ( Eds.). Nanoliposomes: From Fundamentals to Recent Developments; Trafford Pub, Ltd: Oxford, UK, 2005; 91–98.
- Acosta, E. Bioavailability of nanoparticles in nutrient and nutraceutical delivery. Current Opinion. Colloid & Interface Science 2009, 14, 3–15.
- Huang, Q.R.; Yu, H.L.; Ru, Q.M. Bioavailability and delivery of nutraceuticals using nanotechnology. Journal of Food Science 2010, 75, 50–57.
- Liu, C.; Yang, S.; Liu, W.; Wang, R.; Wan, J.; Liu, W. Preparation and characterization of medium chain fatty acid liposomes by lyophilization. Journal of Liposome Research 2010, 20, 183–190.
- Liu, W.; Liu, W.L.; Liu, C.M.; Liu, J.H.; Yang, S.B.; Zheng, H.J.; Lei, H.W.; Ruan, R.; Li, T.; Tu, Z.C.; Song, X.Y. Medium-chain fatty acid nanoliposomes for easy energy supply. Nutrition 2011, 27, 700–706.
- Yang, S.; Liu, W.; Liu, C.; Liu, W.; Tong, G.; Zheng, H.; Zhou, W. Characterization and bioavailability of vitamin C nanoliposomes prepared by film evaporation-dynamic high pressure microfluidization. Journal of Dispersion Science and Technology 2011. DOI:10.1080/01932691.2011.629511.
- Betageri, G.V.; Parsons, D.L. Drug encapsulation and release from multilamellar and unilamellar liposomes. International Journal of Pharmaceutics 1992, 81, 235–241.
- Di Giulio, A.; Saletti, M.A.; Impagnatiello, A.; Lucarelli, M.; Strom, R.; Oratore, A. Encapsulation and efflux of lactone and hydroxy acid forms of simvastatin in reverse phase evaporation vesicles. International Journal of Pharmaceutics 1993, 89, 133–138.
- Hombreiro-Pérez, M.; Siepmann, J.; Zinutti, C.; Lamprecht, A.; Ubrich, N.; Hoffman, M.; Bodmeier, R.; Maincent, P. Non-degradable microparticles containing a hydrophilic and/or a lipophilic drug: preparation, characterization and drug release modeling. Journal of Controlled Release 2003, 88, 413–428.
- Hammady, T.; El-Gindy, A.; Lejmi, E.; Dhanikula, R.S.; Moreau, P.; Hildgen, P. Characteristics and properties of nanospheres co-loaded with lipophilic and hydrophilic drug models. International Journal of Pharmaceutics 2009, 369, 185–195.
- Xia, S.; Xu, S. Ferrous sulfate liposomes: Preparation, stability and application in fluid milk. Food Research International 2005, 38, 289–296.
- Marsanasco, M.; Márquez, A.L.; Wagner, J.R.; Alonso, S.V.; Chiaramoni, N.S. Liposomes as vehicles for vitamins E and C: An alternative to fortify orange juice and offer vitamin C protection after heat treatment. Food Research International 2011. DOI:10.1016/j.foodres. 2011. 07. 025.
- Sharma, A.; Sharma, U.S. Characteristics and properties of nanospheres co-loaded with lipophilic and hydrophilic drug models. International Journal of Pharmaceutics 1997, 154, 185–195.
- Alex, R.; Bodmeier, R. Encapsulation of water-soluble drugs by a modified solvent evaporation method. I. Effect of process and formulation variables on drug entrapment. Journal of Microencapsulation 1990, 7, 347–355.
- Cohen, S.; Yoshioka, T.; Lucarelli, M.; Hwang, L.H.; Langer, R. Controlled delivery systems for proteins based on poly (lactic/glycolic acid) microspheres. Pharmaceutical Research 1991, 8, 713–720.
- Liu, W.; Liu, J.; Xie, M.; Liu, C.; Liu, W.; Wan, J. Characterization and high-pressure microfluidization-induced activation of polyphenoloxidase from Chinese pear (Pyrus pyrifolia Nakai). Journal of Agricultural and Food Chemistry 2009, 57, 5376–5380.
- Takahashi, M.; Inafuku, K.; Miyagi, T.; Oku, H.; Wada, K.; Imura, T.; Kitamoto, D. Efficient preparation of liposomes encapsulating food materials using lecithins by a mechanochemical method. Journal of Oleo Science 2007, 56, 35–42.
- Bharali, D.J.; Sahoo, S.K.; Mozumdar, S.; Maitra, A. Cross-linked polyvinylpyrrolidone nanoparticles: a potential carrier for hydrophilic drugs. Journal of Colloid and Interface Science 2003, 258, 415–423.
- Zheng, S.; Alkan-Onyuksel, H.; Beissinger, R.L.; Wasan, D.T. Liposome microencapsulations without using any organic solvent. Journal of Dispersion Science and Technology 1999, 20, 1189–1203.
- Jafari, S.M.; He, Y.; Bhandar, B. Nano-emulsion production by sonication and microfluidization-a comparison. International Journal of Food Properties 2006, 9, 475–485.
- Sorgi, F.L.; Huang, L. Large scale production of DC-Chol cationic liposomes by microfluidization. International Journal of Pharmaceutics 1996, 144, 131–139.
- Awasthi, V.D.; Garcia, D.; Klipper, R.; Goins, B.A.; Phillips, W.T. Neutral and anionic liposome-encapsulated hemoglobin: Effect of postinserted poly (ethylene glycol)-distearoylphosphatidylethanolamine on distribution and circulation kinetics. Journal of Pharmacology and Experimental Therapeutics 2004, 309, 241–248.
- Hombreiro-Pérez, M.; Zinutti, C.; Lamprecht, A.; Ubrich, N.; Astier, A.; Hoffman, M.; Bodmeier, R.; Maincent, P. The preparation and evaluation of poly ([epsilon]-caprolactone) microparticles containing both a lipophilic and a hydrophilic drug. Journal of Controlled Release 2000, 65, 429–438.
- Lepage, G.; Roy, C.C. Direct transesterification of all classes of lipids in a one-step reaction. Journal of Lipid Research 1986, 27, 114–120.
- Samman, S.; Chow, J.W.Y.; Foster, M.J.; Ahmad, Z.I.; Phuyal, J.L.; Petocz, P. Fatty acid composition of edible oils derived from certified organic and conventional agricultural methods. Food Chemistry 2008, 109, 670–674.
- Arya, S.P.; Mahajan, M.; Jain, P. Photometric methods for the determination of Vitamin C. Analytical Sciences 1998, 14, 889–895.
- Hernández, Y.; Lobo, M.G.; González, M. Determination of vitamin C in tropical fruits: A comparative evaluation of methods. Food Chemistry 2006, 96, 654–664.
- Korus, A. Level of Vitamin C, polyphenols, and antioxidant and enzymatic activity in three varieties of Kale (Brassica Oleracea L. Var. Acephala) at different stages of maturity. International Journal of Food Properties 2011, 14, 1069–1080.
- Zhao, L.; Xiong, H.; Peng, H.; Wang, Q.; Han, D.; Bai, C.; Liu, Y.; Shi, S.; Deng, B. PEG-coated lyophilized proliposomes: preparation, characterizations and in vitro release evaluation of vitamin E. European Food Research and Technology 2011, 232, 647–654.
- Mu, X.M.; Zhong, Z.S. Preparation and properties of poly (vinyl alcohol)-stabilized liposomes. International Journal of Pharmaceutics 2006, 318, 55–61.
- Hong, Y.J.; Kim, J.C. Egg phosphatidylcholine liposomes incorporating hydrophobically modified chitosan: pH-sensitive release. Journal of Nanoscience and Nanotechnology 2011, 11, 204–209.
- Alonso-Romanowski, S.; Chiaramoni, N.S.; Lioy, V.S.; Gargini, R.A.; Viera, L.I.; Taira, M.C. Characterization of diacetylenic liposomes as carriers for oral vaccines. Chemistry and Physics of Lipids 2003, 122, 191–203.
- Hatziantoniou, S.; Nezis, I.P.; Margaritis, L.H.; Demetzos, C. Visualisation of liposomes prepared from skin and stratum corneum lipids by transmission electron microscopy. Micron 2007, 38, 777–781.
- Wang, T.; Deng, Y.; Geng, Y.; Gao, Z.; Zou, J.; Wang, Z. Preparation of submicron unilamellar liposomes by freeze-drying double emulsions. Biochimica et Biophysica Acta-Biomembranes 2006, 1758, 222–231.
- Fan, M.; Xu, S.; Xia, S.; Zhang, X. Preparation of salidroside nano-liposomes by ethanol injection method and in vitro release study. European Food Research and Technology 2008, 227,167–174.
- Maitani, Y.; Igarashi, S.; Sato, M.; Hattori, Y. Cationic liposome (DC-Chol/DOPE = 1: 2) and a modified ethanol injection method to prepare liposomes, increased gene expression. International Journal of Pharmaceutics 2007, 342, 33–39.
- Elbayoumi, T.A.; Torchilin, V. P. Enhanced cytotoxicity of monoclonal anticancer antibody 2C5-modified doxorubicin-loaded PEGylated liposomes against various tumor cell lines. European Journal of Pharmaceutical Sciences 2007, 32, 159–168.
- Lagoueyte, N.; Paquin, P. Effects of microfluidization on the functional properties of xanthan gum. Food Hydrocolloids 1998, 12, 365–371.
- Gulati, M.; Grover, M.; Singh, S.; Singh, M. Lipophilic drug derivatives in liposomes. International Journal of Pharmaceutics 1998, 165, 129–168.
- Maestrelli, F.; Garcia-Fuentes, M.; Mura, P.; José Alonso, M. A new drug nanocarrier consisting of chitosan and hydoxypropylcyclodextrin. European Journal of Pharmaceutics and Biopharmaceutics 2006, 63, 79–86.