Abstract
The effect of high hydrostatic pressure-750 on rheological properties of rice starch-water system was examined. The starch dispersions (20% w/v) were pressurized at 120, 240, 360, 480, and 600 MPa for 30 min. The storage modulus and loss modulus increased with the pressure increase. A yield stress was generated at a low shear rate. The high hydrostatic pressure-treated starch gels performed shear thinning and the apparent viscosity dropped sharply at 480 MPa. Hysteresis loop area increased with the pressure increased and reached the maximum at 600 MPa. High hydrostatic pressure-treated starch gels were found to be weak gels and thixotropic.
INTRODUCTION
Starch is classified as A-type, B-type, and C-type according to its crystallinity dependent on the configuration of the unit cell of the double helix of the polysaccharides.[Citation1] Cereal starches such as rice, corn, waxy corn, wheat, etc., in most of the cases, show the A-pattern. Rheology is the study of the manner in which materials respond to applied stress or strain.[Citation2,] The rheological response of a material depends on its molecular interactions,[Citation3] thus it can be considered as an analytical tool to provide fundamental insights on the structural organization of food. In food technology, the specific adjustment of the flow behavior of starch gels is of significance in order to be able to regulate production processes and to optimize applicability, stability, and sensory properties of end products.[Citation4] Rice starch is an important renewable resources widely used in food industry. Unmodified native starches have very limited use in the food industry because of their lack of stability under temperature, shear, pH, and refrigeration conditions.[Citation5] Based on the rheological properties, modified rice starch is utilized as a thickening agent, such as in milk-based sweets and jellies, sauces, custards, and desserts.[Citation6] Moreover, modified rice starch can be used as a dispersed phase in electro-rheological fluids and in starch-based coating colors.[Citation7]
High hydrostatic pressure (HHP) is a non-thermal processing technology. It can be considered as the proper or suitable technique for minimally processed and long shelf-life foods,[Citation8] and it has been used in commercial production such as fruits, vegetables, and meat in Japan, America, and Europe. HHP treatment causes disordering of biopolymers, including proteins and starch, as it modifies non-covalent intermolecular interactions.[Citation9] For starch, this disordering leads to pressure-induced gelatinization. A small change in molecular conformation and structure of starch can bring about dramatic changes in its functional properties. Accordingly, this technology has great potential to produce novel starch-containing foods with desired textural characteristics.[Citation10] It would be a great contribution on food safety to get modified starch by HHP instead of chemical processes. Also it would bring much economic benefit because HHP could reduce the gelatinization temperature of starch; meanwhile it could significantly increase the stableness of product as a food additive.
Extensive literature is available on the pasting properties of rice starch caused by HHP. Hayashi[Citation11] observed that a thick suspension of rice starch forms a ball by standing against its own weight after pressurization at 700 MPa.[Citation11] Li et al.[Citation12] studied the microscopy of rice starch dispersion at 600 MPa for 30 min and found that the treatment caused a complete loss of birefringence and a gel-like appearance. A significant increase was observed in swelling power and solubility at low temperature (50–60°C), but opposite trends were found at high temperature (70–90°C).[Citation12] But the rheological properties and microstructure of the pressure-induced gelatinized samples were different from those of heat-induced gelatinized samples. It was reported by Oh et al.[Citation13] that ŋinitial value of normal rice starch and waxy rice starch increased with the increase in pressure level.[Citation13] No attempt has been especially made to study rheological properties of HHP-treated rice starch from dynamic moduli, model fitting, and shear thinning under both steady and dynamic shear stress. In the HHP-rice starch system, understanding the role of treatment pressure and time is also important for improving the rheological properties of HHP rice starch-based products. The first commercial HHP treated products appeared on the market in 1991 in Japan, while HHP processing is now being used for products such as fruit juices, jams, sauces, rice, cakes, and crustacean seafood as sterilization, gelatinization, and shucking method. There is increasing worldwide interest in the use of HHP because of the advantages of this technology over other methods of processing and preservation. The main objective of this study was to investigate the effect of HHP on rheological properties of rice starch treated at different high pressures and treatment times in both steady and dynamic shear, offering a theory support for the application of HHP in starch-based food.
MATERIALS AND METHODS
Materials
Commercial japonica rice (short grain rice) (Toyonishiki variety, Xuesong Brand, Liaoning, China) used in this study was purchased from a local market in Beijing.
Sample Preparation
Rice starch was isolated as described by Singh, Sodhi, and Singh.[Citation14] Rice grains were steeped in water containing 0.2% sodium hydroxide in a ratio of 1:5 w/v for 24 h at 25oC. The soaked liquor was drained off and the rice was ground in a laboratory grinder. The rice flour was mixed with water containing 0.2% sodium hydroxide in a ratio of 1:5 w/v for 10 min and left to stand in a beaker for 12 h and then the supernatant was removed. The process was repeated until the outflow was transparent and gave a negative reaction to the Biuret test for protein. Sediment was suspended in distilled water, passed through a 200-mesh nylon cloth, and washed with distilled water repeatedly until the supernatant no longer showed any pink color with the phenolphthalein. The starch was collected and dried in a cabinet drier for 24 h at 40oC. The dried starch went through a 200 gauge mesh sieve and was preserved in an airtight container at room temperature.
HHP Treatment
Rice starch-water suspensions (20% w/w) were vacuum-packed in 200 mL polyethylene bags with a poly heat bag sealer. Pressure treatments were conducted by a HHP device (high pressure press type HHP-750, produced by Kefa High Pressure Food Processing Inc., Baotou, China) with a cylindrical pressure chamber as described by Chan et al.[Citation15] and Li et al.[Citation16] The pressure-transmitting medium was water, and the maximum working pressure of the unit was 600 MPa. The samples were treated at pressures of 120, 240, 360, 480, and 600 MPa for 30 min at room temperature. Pressure was increased at a rate of approximately 2.4 MPa s−1. The time course of the experiment began when the desired pressure was reached. After the high-pressure treatment, the sample bags were opened and vacuum filtered, frozen by liquid nitrogen, and then freeze-dried. Each dried starch sample was ground into a fine powder through 100 gauge mesh sieve after fully pulverizing in a laboratory blender, and then kept in an airtight container at room temperature for further analysis.
Rheological Measurements
The pasting properties of starch samples were obtained with a strain/stress controlled rheometer AR500 (TA Instrument, Waters Co., Ltd., Surrey, UK) equipped with a cone-plate configuration using a cone diameter (40 mm dia., angle 2o) at gap 0.05 mm. The starch samples were set on the rheometer directly to test pasting properties first and then dynamic and steady rheological properties. Comparing with the measurement of pasting properties regularly conducted with Rapid Visco-Analyzer (RVA), that of pasting and rheological properties by rheometer at once was less affected by the transmission time, temperature, agitation, and shear stress. It was shown that the pasting of the starch dispersions happen in a similar matter with either a RVA or rheometer.[Citation17]
Starch-water slurry of 8% was deposited in the middle of the testing plate. The exposed sample edge was covered with a thin layer of light paraffin oil to prevent evaporation or absorption of atmospheric moisture during measurements. The temperature ramp measurement procedure was selected. Procedure of temperature increase was from 20 to 95oC, which enables a slurry system of rice starch to become a paste and the temperature decreased from 95 to 20oC. The heating rate was 4oC s−1 and the cooling rate was 5oC s−1. The shear rate was set at 200 s−1.
Dynamic-shear properties were obtained from frequency sweeps over the range of 0.1–100 rad s−1 at 0.5% strain. The 0.5% strain was in the linear viscoelastic region. Frequency sweep tests were performed at 25oC. Dynamic moduli G′ (elastic or storage modulus), G“ (viscous or loss modulus) and tanδ (G„/G’) (related with the overall viscoelastic response) were obtained. The shear thinning data were obtained from steady state flow step over the shear rate range of 0–300 s−1 at 25oC. The hysteresis loop area was obtained from steady state flow step over the shear rate range of 300–0 s−1 at 25oC.
Data Analysis and Calculations
In order to test the flow behavior of starch suspension samples, the data were fitted to the following models.
The power-law model:
(1)
where
is the shear stress (Pa),
the shear rate (s−1), K the consistency coefficient (Pa·sn), and n the flow behavior index (dimensionless).[Citation18,Citation19]
Herschel–Bulkley model:
(2)
where
is the yield stress (Pa).[Citation18,Citation20]
Casson model:[Citation18,Citation21]
(3)
Cross model:
(4)
where
and
(Pa s) are the zero-shear viscosity (when
= 0) and the infinite-shear viscosity, respectively,
(s) is a characteristic time.[Citation20]
Hysteresis Loop:
The percentage of relative hysteresis area was calculated by the following equation.[Citation21]
(5)
Areas under the upstream data points (Aup) and under the downstream data points (Adown) as well as the hysteresis area (Aup - Adown) were obtained using Microcal Origin 7.5 (Microcal Software, Inc., Northampton, MA).
All statistical computations and analyses were conducted using SPSS version 16.0 for Windows and Microsoft Excel 2003. Experimental data were expressed as mean value ± standard deviation. A Duncan’s multiple range test was conducted to assess significant differences among experimental mean values (p < 0.05). The data were plotted using the software Microcal Origin 7.5 (Microcal Software, Inc., Northampton, MA). All measurements were conducted in triplicate.
RESULTS AND DISCUSSION
Pasting Behavior Measurements
Statistical analysis of all pasting parameters variations including peak viscosity (PV), trough viscosity, breakdown, final viscosity (FV), setback, and peak time (PT) of rice starch are summarized in . The PV of HHP-treated rice starch was significantly reduced as the pressure increased from 120 to 360 MPa (p < 0.05). PV values of rice starch pressurized at 480 and 600 MPa were significantly higher (p < 0.05) than those of HHP-treated samples from 120 to 360 MPa. PT values of the rice starch were significantly reduced with the increase in pressure level from 120 to 360 MPa (p < 0.05). PT values were prolonged significantly at 480 and 600 MPa (p < 0.05). Trough viscosity (TV) and FV values of treated rice starch at 480 and 600 MPa were significantly higher than those of native rice starch as shown in (p < 0.05).
TABLE 1 Pasting properties of HHP-treated rice starch (30 min) at different pressures from rotational rheometers
PV indicates the maximum swelling extent of rice starch granule before physically breaking down. A high PV value suggested a presence of strong bonding forces within the granule interior.[Citation22] Breakdown (BD) value indicates the ability of resisting shear stress and heat. Rice starch granules with low BD values were more resistible to shear stress and heat.[Citation23] They were not easy to be destroyed. Setback (SB) value shows the consistency of the gel and the involvement of starch retrogradation.[Citation24] Rice starch granules with high SB values were liable to retrogradation.
The extent of granule destruction was different with different pressure levels of the treatment. At low pressures, the structure of rice starch was inattentive so disruption was easily happened in a heating solution. High pressures (480 to 600 MPa) resulted in the loss of granule integrity, subsequent disruption and a release of more solubilized starch, primarily amylose. It appeared that a helica complex was developed by certain leached starch molecules and lipids.[Citation12] The interactions between them could be responsible for the increases in PV, PT, TV, and FV values. At high pressure levels, the rice starch molecules become closely aligned and there were more ruptured starch granules which were not readily destroyed during the pasting process. This was in accordance with the scanning electron micrographs of HHP rice starch samples invested by Li et al.[Citation12] No regular changes in breakdown and setback values of HHP-treated rice starch were observed. This may relate to the varying degrees of destruction in interior structure.
Dynamic Rheological Behavior Measurements
shows the changes in storage modulus (a) and loss modulus (b) as a function of frequency for rice starch after HHP treatment at different pressures for 30 min at 20°C. G′ modulus showed a behavior with an initial rise in modulus, followed by a much slower increase on attainment of the pseudo-plateau region; while G′′ showed a quick rise at low frequency then a slow increase at high frequency. G′ and G′′ of rice starch gel were significantly increased with the increase in frequency before and after HHP treatment (p < 0.05). During the whole frequency, G′ of the sample was significantly higher than G′′ (p < 0.05); the curves of G′ and G′′ remained non-overlapping, which indicated a typical weak gel.[Citation25] Therefore, native and HHP-treated rice starches were both weak gels. G′ and G′′ of rice starch were significantly increased with the increase in treatment pressure level as shown in , (p < 0.05).
FIGURE 1 Variation of (a) storage modulus and (b) loss modulus for HHP-treated rice starch (30 min) at different pressures. Measurements were made at 25°C and 0.5% strain.
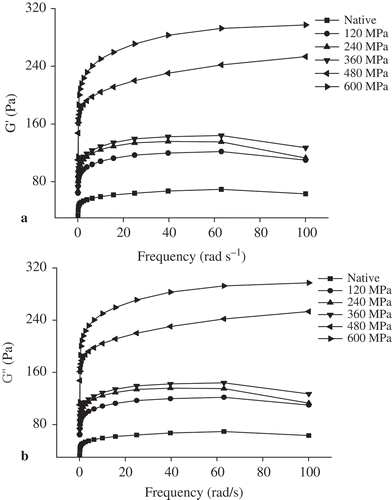
The initial quick rise was thought to be related to the establishment of a three-dimensional network structure between leached amylose and amylopectin and to involve the conversion of increasing amounts of chains in the sol fraction into a gel. The subsequent slower rise may be caused by further cross-linking paralleled by an annealing process such as rearrangement of cross-links and lateral chain aggregation, resulting in a net decrease in the length of elastically active chains.[Citation26] More amylose and amylopectin leased with the increasing in pressure therefore the G′ and G′′ increased as the pressure raised.
The gel strength is related to the content of amylose in starch. Rice starch is A-type with many short chains and the hydrogen bond between short chains and water molecules was weak; therefore, it is easily ruptured and forms a weak gel. It was illustrated that HHP enhanced the gel strength of rice starch, and the gel strength increased as the pressure increased. This was resulted from the change of rice starch granules structure. Hydrogen bonding between starch molecules was disrupted by pressure and the original pattern of the molecules was messed up. The crystalline structure and even the whole starch granule were destroyed by high pressures. Consequently, granules were difficult to be ruptured by heating and shear stress in a solution and the flexibility of rice starch gel was improved. Effect of HHP treatment on rheological properties of different types of starch has been investigated by some researchers. Stolt et al. reported that G′ value of barley starch increased with extending in treatment time.[Citation26] Also G′ of sticky corn starch first increased and then decreased with prolonging in treatment time at pressures higher than 500 MPa.[Citation27] It was explored that G′ of 10% potato starch and 25% barley starch increased with the increase in treatment time.[Citation26] Changes in dynamic viscoelastic properties of starch were affected by process parameters including temperature ranges, treatment time, pressure levels, solvent, etc.[Citation28] Therefore, a further study should be carried on about the effects on dynamic rheological properties of rice starch.
Steady Rheological Flow Measurements
Flow curves of HHP-treated rice starch at different pressures for 30 min at 20°C are shown in . Curves of HHP-treated rice starch were convex to the shear stress axis. The shear stress increased with the increase in shear rate. The rice starch had a spur form in flow curves at the shear rate of 50 s−1.
The HHP-treated rice starch gels were non-Newtonian fluids. In order to perform a quantitative comparison of the starch samples, shear stress-shear rate data were tested based on various rheological models (power law, Herschel-Bulkley, Casson and Cross). It was shown that all the HHP-treated starch gels exhibited extremely thixotropic flow with gel strength having a spur form in the hysteresis loop. The first peak in these upward flow curves was related to the stress needed to start the sample flow and represents the breakdown of the structure, which was referred as yield stress.[Citation29] Rao and Kenny[Citation30] demonstrated that yield stress directly affects the amount of material retained on the walls of containers and process vessels.[Citation30] Lang and Rha[Citation31] emphasized that the experimentally determined yield value was dependent on the method and condition of measurement.[Citation31] Yield stress caused the solution to revert to its normal viscous flow. This was in accordance with the study of the control yeast extract[Citation32] and the 5% curdlan aqueous suspension.[Citation33] Therefore, these flow curves did not follow any of the typical viscous model behavior patterns.[Citation34]
During shearing, starch molecules changed from interweaving in three-dimensional networks to moving along the flow direction. During the temperature declining process, starch molecules joined to a network of higher strength with hydrogen bond. During the steady flow test, as the shear stress increased with the increase in shear rate; when the starch gel of network structure flowed; consequently friction appeared between the walls in the network. More and more starch molecules began to flow instead of maintaining a network, thus the apparent viscosity decreased at a high shear rate. After the peak, the shear stress increased gently with the increase in shear rate. This was in accordance with the study of Banchathanakij and Suphantharika.[Citation34] The first peak of the flow curves was caused by the increase of shear rate. Large shear stress was needed to destroy the network structure of the starch gel; then more and more starch molecules performed the “liquid-like” behavior instead of the previous “gel-like” behavior.[Citation29] The structure of rice starch was destroyed; the swelling of the granules was limited; the content of soluble starch was low. At the same shear rate, the strength of starch gel was higher than that of the native starch, which led to the change of shear stress vs. shear rate profile.
Apparent Viscosity of HHP-Treated Starch
The apparent viscosity of rice starch after HHP treatment at different pressures for 30 min at 20°C is shown in . A remarkable role of HHP was displayed on apparent viscosity of rice starch gel. At the same pressure level, apparent viscosity declined with the increase in shear rate. When the shear rate was fixed, apparent viscosity of rice starch paste increased after HHP treatment at pressure levels 120 and 360 MPa; however, it was significantly decreased after treatment at 360 MPa and then increased after treatment from 480 to 600 MPa. The rice starch sample treated had the lowest apparent viscosity at 480 MPa. These indicated that different pressure levels had different effects on rice starch granules. Meanwhile, there was a pressure point when the starch granules began to be disrupted between 480 and 600 MPa. When the pressure was above the point, there was an obvious sudden change in granule structure, apparent viscosity, and other characteristics of starch paste.
Shear Thinning of Starch Gel
Shear thinning of rice starch after HHP treatment at different pressures for 30 min at 20 °C is shown in . After the starch slurry becomes paste, the apparent viscosity of rice starch first declined drastically then becomes smooth with the increase in shear rate, exhibiting a shear thinning behavior.
Non-Newtonian fluid is characterized by shear-thinning behavior, which means the viscosity decreased with an increase in shear rate.[Citation35] Shear thinning is generally beneficial for the application and processing. In certain range of shear rate, it can greatly decrease the viscosity of the materials and increase its fluidity so as to benefit the pumping transportation and processing, decrease energy consumption and increase productivity.[Citation36] Shear thinning occurred at lower shear rate and it could be attributed to stress-induced breakdown of the network structure. After a sharp reduction the viscosity change was smoothened at high shear rates (). This could be due to the onset of entanglements caused by the presence of the highly branched, high molecular weight amylopectin.[Citation37] Bhandari et al.[Citation38] and Morris[Citation39] reported that this shear-thinning behavior can be explained by breaking of an entangled polysaccharide molecule network during shearing. In an entangled network system, the rate of disruption of existing intermolecular entanglements becomes greater than the rate of reformation of intermolecular entanglement with increasing shear rate. The result is less intermolecular resistance to flow and a lower apparent viscosity.[Citation19]
Hysteresis Loop
When rice starch paste was sheared first at increasing and then at decreasing shear rates, the upward and downward curves of shear stress vs. shear rate profiles do not coincide, thus causing a hysteresis loop. Hysteresis loop is a typical estimate to study thixotropy, which takes some non-Newtonian fluid finite time to attain equilibrium viscosity when introduced to a step change in shear rate. The area enclosed by the hysteresis loop can be interpreted as structural breakdown by the shear field to alter a structure or form a new structure, which then maintained a shear-thinning characteristic on following shear sweeps.[Citation40] It is an indication of the degree of structural breakdown due to shearing.[41] shows relative hysteresis loop area of rice starch pastes treated at different pressures.
FIGURE 5 Experimental hysteresis area values of HHP-treated rice starch (30 min) at different pressures.
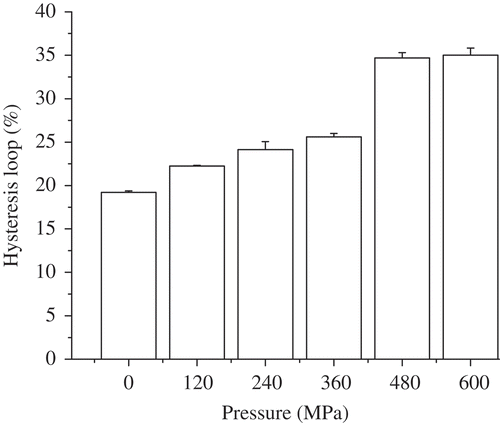
The hysteresis loop area of rice starch paste increased with the increase in pressure from 0 to 600 MPa. Generally, a larger hysteresis loop area suggests a greater extent of destruction in gel structure. The experimental data demonstrated that rice starch paste treated at 600 MPa needed the highest energy to breakdown the structure. This may be attributed to the helical complex combined by molecules and lipids and the intertwinement of amylase-lipid complex and amylopectin molecules at high pressures.
CONCLUSION
Both native and HHP-treated rice starch dispersion systems were weak gels. They belonged to non-Newtonian fluids, displaying shear thinning behavior. A yield stress was generated at low shear rate. HHP caused structure changes of starch granules, including dissolution of amylose and amylopectin, rearrangement of starch molecules, destruction of their crystalline structures, and the formation of amylose-liquid complex. These effects limited the swelling of starch granules under shear stress and heat, Thus, the pasting properties and rheological properties changed dramatically at high pressures.
FUNDING
This work was supported by the Fund of National Natural Science Foundation (No. 30972067) and the National Key Technology R&D Program of the Ministry of Science and Technology of People’s Republic of China (No.2011AA100801).
Additional information
Funding
REFERENCES
- Knorr, D.; Heinz, V.; Buckow, R. High pressure application for food biopolymers. Biochimica et Biophysica Acta-Proteins and Proteomics 2006, 1764, 619–631.
- Steffe, J.F. Rheological Methods in Food Process Engineering, 2nd Ed; Freeman Press: East Lansing, MI, 1996.
- Chamberlain, E.K.; Rao, M.A. Rheological properties of acid converted waxy maize starches in water and 90% DMSO/10% water. Carbohydrate Polymers 1999, 40, 251–260.
- Kulicke, W.M.; Eidam, D.; Kath, F.; Kix, M.; Kull, A.H. Hydrocolloids and rheology regulation of visco-elastic characteristics of waxy rice starch in mixtures with galactomannans. Starch/Stärke 1996, 48, 105–114.
- Zhang, L.T. Denaturalized Starch, South China University of Science and Technology Press: Guangzhou, 1992.
- Da Silva, P.M.S.; Oliveira, J.C.; Rao, M.A. Rheological properties of heated cross-linked waxy maize starch dispersions. International Journal of Food Properties 1998, 1, 23–34.
- Groen, J.; Eklund, D. Influence of starch modification on coating color and coated layer properties. Wochenblatt Für Papierfabrikation 1998, 126, 879–884.
- Farr, D. High pressure technology in the food industry. Trends in Food Science & Technology 1990, 1, 14–16.
- Balny, C. High pressure and protein oligomeric dissociation. High Pressure Research 2002, 22, 737–741.
- Jayaprakasha, H.M.; Yoon, Y.C.; Brueckner, H. Critical aspects in adoption of ultra high pressure technology for food processing. Korean Journal for Food Science of Animal Resources 2000, 20, 44–55.
- Hayashi, R. High-pressure food processing of rice and starch foods. In: Proceedings of World Rice Research; Toriyama, K.; Heong, K.L.; Hardy, B.; Eds.; Japan International Research Center for Agricultural Sciences: Tsukuba, 2005; 590.
- Li, W.; Bai, Y.; Mousaa, S.A.S.; Zhang, Q.; Shen, Q. Effect of high hydrostatic pressure on physicochemical and structural properties of rice starch. Food and Bioprocess Technology 2012, 5 (6), 2233–2241, DOI:10.1007/11947-011-0542-6.
- Oh, H.E.; Hemar, Y.; Anema, S.G.; Wong, M.; Neil Pinder, D. Effect of high-pressure treatment on normal rice and waxy rice starch-in-water suspensions. Carbohydrate Polymers 2008, 73, 332–343.
- Singh Sodhi, N.; Singh, N. Morphological, thermal, and rheological properties of starches separated from rice cultivars grown in India. Food Chemistry 2003, 80, 99–108.
- Chan, H.T.; Leh, C.P.; Bhat, R.; Senan, C.; Williams, P.A.; Karim, A.A. Molecular structure, rheological, and thermal characteristics of ozone-oxidized starch. Food Chemistry 2011, 126, 1019–1024.
- Li, W.; Zhang, F.; Liu, P.; Bai, Y.; Gao, L.; Shen, Q. Effect of high hydrostatic pressure on physicochemical, thermal, and morphological properties of mung bean (Vigna radiata L.) starch. Journal of Food Engineering 2011, 103, 388–393.
- Zhong, F.; Li, Y.; Ibanez, A.M.; Oh, M.H.; Mckenzie, K.S.; Shoemaker, C. The effect of rice variety and starch isolation method on the pasting and rheological properties of rice starch pastes. Food Hydrocolloids 2009, 23, 406–414.
- Mujoo, R.; Ali, S.Z. Changes in physico-chemical and rheological properties of rice during flaking. International Journal of Food Properties 2000, 3, 117–135.
- Park, S.; Chung, M.G.; Yoo, B. Effect of octenylsuccinylation on rheological properties of corn starch pastes. Starch/Stärke 2004, 56, 399–406.
- Peressini, D.; Bravin, B.; Lapasin, R.; Rizzotti, C.; Sensidoni, A. Starch–methylcellulose based edible films: Rheological properties of film-forming dispersions. Journal of Food Engineering 2003, 59, 25–32.
- Tárrega, A.; Durán, L.; Costell, E. Flow behaviour of semi-solid dairy desserts. Effect of temperature. International Dairy Journal 2004, 14, 345–353.
- Hoover, R. Composition, molecular structure, and physicochemical properties of tuber and root starches: A review. Carbohydrate Polymers 2001, 45, 253–267.
- Jyothi, A.N.; Moorthy, S.N.; Vimala, B. Physicochemical and Functional Properties of Starch from two Species of Curcuma. International Journal of Food Properties 2003, 6, 135–145.
- Zaidul, I.S.M.; Yamauchi, H.; Kim, S.J.; Hashimoto, N.; Noda, T. RVA study of mixtures of wheat flour and potato starches with different phosphorus contents. Food Chemistry 2007, 102, 1105–1111.
- Clark, A.H.; Ross-Murphy, S.B. Structural and mechanical properties of biopolymer gels. Advances in Polymer Science 1987, 83, 57–192. 31. Lai, L.S.; Liao C.L. Dynamic rheology of structural development in starch/decolourised hsian-tsao leaf gum composite systems. Journal of the Science of Food and Agriculture 2002, 82, 1200–1207.
- Stolt, M.; Stoforos, N.G.; Taoukis, P.S.; Autio, K. Evaluation and modelling of rheological properties of high pressure treated waxy maize starch dispersions. Journal of Food Engineering 1999, 40, 293–298.
- Tan, F.J.; Dai, W.T.; Hsu, K.C. Changes in gelatinization and rheological characteristics of japonica rice starch induced by pressure/heat combinations. Journal of Cereal Science 2009, 49, 285–289.
- Zhu, L.; Sun, N.; Papadopoulos, K.; Kee, D.D. A slotted plate device for measuring static yield stress. Journal of Rheology 2001, 45, 1105–1123.
- Rao, M.A.; Kenny, J.F. Flow properties of selected food gums. Canadian Institute Food Science Technology Journal 1975, 8, 142–146.
- Lang, E.R.B.; Rha, C. Determination of the yield stress of hydrocolloid dispersions. Journal of Texture Studies 1981, 12, 47–62.
- Halmos, A.L.; Tiu, C. Liquid foodstuffs exhibiting yield stress and shear-degradability. Journal of Texture Studies 1981, 12, 39–46.
- Funami, T.; Yada, H.; Nakao, Y. Curdlan properties for application in fat mimetics for meat products. Journal of Food Science 1998, 63, 283–287.
- Banchathanakij, R.; Suphantharika, M. Effect of different β-glucans on the gelatinisation and retrogradation of rice starch. Food Chemistry 2009, 114, 5–14.
- Marcotte, M.; Taherian, A.R.; Trigui, M.; Ramaswamy, H.S. Evaluation of rheological properties of selected salt enriched food hydrocolloids. Journal of Food Engineering 2001, 48, 157–167.
- Silva, P.M.S.D.; Oliveira J.C.; Rao, M.A. Granule size distribution and rheological behavior of heated modified waxy and unmodified maize starch dispersions. Journal of Texture Studies 1997, 28, 123–138.
- Kapoor, B.; Bhattacharya, M. Steady shear and transient properties of starch in dimethylsulfoxide. Carbohydrate Polymers 2001, 44, 217–231.
- Bhandari, P.N.; Singhal, R.S.; Kale, D.D. Effect of succinylation on the rheological profile of starch pastes. Carbohydrate Polymers 2002, 47, 365–371.
- Morris, E.R. Polysaccharide solution properties: Origin, rheological characterization and implications for food system. In: Frontiers in Carbohydrate Research-1; Millane, R.P.; BeMiller, J.N.; Cahndrasekavan, R.; Eds.; Elsevier Applied Science Publishers: London, 1989; 132–163.
- Viturawong, Y.; Achayuthakan, P.; Suphantharika, M. Gelatinization and rheological properties of rice starch/xanthan mixtures: Effects of molecular weight of xanthan and different salts. Food Chemistry 2008, 111, 106–114.
- Yoo, D.; Yoo, B. Rheology of rice starch-sucrose composites. Starch/Stärke 2005, 57, 254–261.