Abstract
Grinding characteristics of soy and red gram were compared employing single- and two-stage grinding in a mixer grinder. The initial size as well as hardness of soy (˜216 N) and red gram (˜220 N) were nearly equal. However, final particle size of red gram (61 μm) was much lower than soy (255 μm), indicating that red gram was relatively easier to grind. Analysis of microstructure indicated that typical internal structure and intercellular forces could play a significant role in determining the grinding characteristics of individual grains. With the same amount of energy input (200 kJ/kg), size reduction ratio in soy increased from 12 to 16 during two-stage grinding. Energy and particle analyses revealed that a two-stage segregated grinding is suitable for soy while a single-stage grinding is adequate for red gram. Classical dry grinding laws were applicable after initial break up suggesting their suitability for secondary grinding of soy and red gram.
INTRODUCTION
Size reduction of solids is one of the major unit operations in food, pharmaceutical, and the mineral industry. Grinding is achieved by mechanical stress followed by rupture and the energy required depending upon the hardness of the material and also upon the tendency of the material to its friability.[Citation1] Dry grinding is a mere size reduction operation whereas wet grinding involves both physical and chemical changes.[Citation2] Generally energy consumption is much higher in wet grinding compared to dry grinding.[Citation3] Wet grinding resulted in much lower average particle size (˜79–131 μm) compared to dry grinding (˜221–297 μm) of rice and pulses.[Citation4]
Grinding is a very energy inefficient process, and it is important to look for avenues to save energy. Some of the energy is taken up in the generation of new surface, but a greater part of it is dissipated as heat. Murthy et al.[Citation5] reported that nearly 99% of the energy supplied was converted in to heat and only 1% was utilized for actual size reduction during dry grinding of black pepper. There were attempts to improve the efficiency of grinding energy by employing microwave drying as a pretreatment step for carrot, wheat, and maize.[Citation6−Citation8] Indira and Bhattacharya[Citation9] studied the grinding characteristics of five legumes in their split form and reported that energy consumption did not entirely depend on the hardness of the samples. It is difficult to determine the minimum energy required for a given size reduction process but with the help of theories such as Kick’s, Rittinger’s, and Bond’s laws, it has become possible to understand the grinding characteristics. It has been found experimentally, that for the grinding of coarse particles in which the increase in surface area per unit mass is relatively small, Kick’s law is a reasonable approximation. For the size reduction of fine powders, in which large areas of new surface are being generated, Rittinger’s law fits the approximation much better.[Citation1,Citation10] But, unfortunately there is no single law, which predicts the performance of various materials during grinding.[Citation6]
In an effort towards developing grain batter industry, studies were initiated in the laboratory on performance evaluation of various wet grinding systems which concluded that colloid mill has a potential for industrial adoption.[Citation4] Further, when the pattern of crushed strength of soaked grains failed to reason out, it was shown that greater slurry viscosity of parboiled rice was responsible for higher power requirement compared to raw rice during wet grinding.[Citation11] The classical grinding laws were basically developed from the studies on dry grinding of hard materials such as coal and limestone[Citation12] and first applied for wet grinding of grains in the laboratory. Sharma et al.[Citation13] described the influence of type of forces involved in various wet grinding systems on the grinding characteristics of rice and its batter quality. Another study on wet grinding of soybean from this laboratory had shown that all the three classical laws were better applicable after the initial grain break-up.[Citation14]
Red gram is a common pulse used in the Indian culinary preparations. To mitigate its short supply, there were attempts to prepare dhal analogues from a blend of various pulse powders. Soy being rich in protein is a natural choice to substitute and augment red gram in these preparations. In the present study, grinding characteristics of soy and red gram having similar hardness were compared in single- and two-stage grinding in a mixer grinder. Applicability of classical grinding laws and energy consumption were also assessed for primary and secondary grinding of soy and red gram.
MATERIALS AND METHODS
Materials
Soybean (Glycine max; JS335 variety) was procured from M/s National Seeds Corporation, Mysore, India. Dehulled red gram (Cajanus cajan; splits) was purchased from local market. Dehulling and splitting of soybean was done in an emery splitting machine developed at the authors’ institute.
Hardness Test
Hardness of soy and red gram splits were measured using Universal Testing Machine (model: LR 5 series, M/s Llyod Instruments, Hampshire, UK). The hardness was measured on compression mode, as the force required for 50% compression of each grain that result in permanent deformation (breaking) of the grain and this breaking/crushing strength was recorded. Measurements were carried out using a 100 kg load cell and a 12 mm diameter probe at a crosshead speed of 50 mm/min. Hardness value was measured for 40 individual grain-splits and after eliminating the extreme and odd readings, the mean of the remaining readings was reported.
Primary Grinding
Samples were ground in a mixer grinder (1 L capacity jar, 550 W, 18,000 rpm; M/s Johnson, Bangalore, India). Grinding operation was performed intermittently (ON: 1 min; OFF: 1–5 min) so as to avoid excessive heating of material. Preliminary experimental runs were conducted to optimize the loading volume; accordingly a loading volume of 520 mL was fixed. Based on the bulk density of raw materials, 400 g soy and 460 g red gram were used in the experimental runs. Primary grinding of soy and red gram were carried out for different durations starting from 4 min up to 16 min in steps of 4 min. Particle size of ground flour was determined using sieve analysis. Soy flour was defatted before sieve analysis/segregation as it would otherwise lead to particle aggregation during sieving. To maintain identical process conditions, red gram flour was also defatted before sieve analysis.
Secondary Grinding
Overtails (particle size >180 μm) of primary ground flour (ground for 4 min) was separated for subjecting to secondary grinding (). Bulk density was measured and accordingly 360 g soy and 475 g red gram flour were used in secondary grinding to maintain the loading volume. Secondary grinding was carried out for different durations starting from 4 min up to 16 min in steps of 4 min. The ground flour was subjected to sieve analysis for size determination.
Two-Stage Grinding
shows the schematic diagram of two-stage grinding process comprising of both primary and secondary grinding steps. Throughs (particle size <180 μm) of primary ground flour (ground for 4 min) and unclassified secondary ground flour together contributed for the output of two-stage grinding. Final particle size was obtained from the sieve analysis of these two mixed fractions.
Defatting
Soy/red gram flour was loaded into a glass column fitted with a tap at the bottom and fresh hexane was passed through the column until the eluent became colorless. Defatted material was spread out and dried flour was used for sieving.
Size Analyses of Soy and Red Gram Splits
Average size of soy and red gram splits was determined from the instant volume displaced by immersing 100 grains in kerosene. From the average volume of single split grain (V), equivalent spherical diameter (D) was calculated as follows.
Particle Size Analysis
Particle size analysis of soy and red gram flour was carried out using sieve analysis.[Citation1] The flour was subjected to successive sieving starting from large to small sieves with 850, 710, 355, 250, 180, 150, 105, 75, 63, 53, and 37 μm openings. The flour sample (100 g) from each batch was placed in the top sieve of the sieve sifter and vibrated for 10 min after which the material remaining on each of the sieves and in the flour collector (pan) were weighed and percentage of fractions in each of the sieves was calculated. The final average particle size was determined graphically by plotting cumulative weight fractions versus sieve size and selecting a notional sieve size, which allows 80% of the ground materials to pass through it.
Grinding Energy and Classical Laws
Grinding theories depend upon the basic assumption that the energy required to produce a change dL in a particle of a typical size dimension L is a simple power function of L. Mathematically, it is expressed as:
These laws of grinding were analyzed for their applicability to grinding soy and red gram. The energy consumed during grinding was measured using a digital energy meter (model SPVR–96, M/s Maharashtra Electric Corporation, Mumbai, India). The net energy consumed during grinding operation was estimated from the following expression:
Microscopic Structure of Soy and Red Gram
Soy and red gram splits were cut either perpendicular or parallel to the hilum and surface fat was removed using hexane. Samples were placed on the scotch tape fixed to a metal stub and gold coated (˜100 °A). The gold coated samples were scanned in a scanning electron microscope (model: Leo 435 VP, M/s Leo Electron Microscopy, Cambridge, UK) and the selected portions depicting distinct morphological features were photographed.
Statistical Analysis
All results presented are the average of three independent experimental runs. Significant differences were determined by the student t-test using Microsoft Data Analysis Tool Pack. Differences were considered significant at p < 0.05 and marked with different superscripts.
RESULTS AND DISCUSSION
Primary Grinding
Soy contains all the three macro-nutrients viz., protein, carbohydrate, and fat in good proportion. Red gram has high carbohydrate content and far less fat content compared to soy.[Citation15,Citation16] Despite the vast difference in the composition of soy and red gram, their hardnesses were nearly the same (). The possible influence of moisture content on mechanical properties was also eliminated as these measurements were made at a uniform moisture content of ˜11% (w.b.). Besides, their sizes were nearly the same prompting to take up a comparative study on the grinding characteristics of these two grains.
Table 1 Physical properties of soy and red gram
Table 2 Grinding characteristics of primary and secondary ground soy and red gram
The reduction in particle size of soy and red gram with duration of grinding is presented in and . Although, hardness and average initial particle diameter of soy and red gram were almost equal, their final average particle sizes after 16 min grinding were 255 and 61 μm, respectively, indicating that red gram is comparatively easier to grind. Size reduction rate was higher in the first 4 min of grinding and thereafter reduced drastically in both soy and red gram (). Indira and Bhattacharya[Citation9] reported that grinding characteristics of different legumes did not entirely depend on their initial size, shape, and hardness. The results of the present study also showed that size reduction of grains does not depend only on the hardness of the grains. Further, it was inferred that hardness measured in terms of crushing strength may not be a reliable indicator while the actual size reduction could depend on the type of grinding forces involved as well as the internal structure of the grain. The type of grinding forces involved in the mixer grinder are cutting and shearing forces.
Secondary Grinding
The percentages of coarse fraction (particle size >180 μm) in 4 min primary ground soy and red gram flour were 41 and 53%, respectively (). With a view to improve the overall size reduction, a secondary grinding step was tested for regrinding the coarse fraction. Generally bulk density increases with reduction in particle size. Accordingly, bulk density of coarse fraction of red gram increased compared to its splits (). However, bulk density of coarse fraction of soy showed a decrease. This is due to defatting effect wherein fat content substantially reduced from 23% to less than 2%, contributing for weight loss without a significant reduction in size. Secondary grinding was carried out for different durations (4–16 min) and particle sizes were analyzed ().
Secondary grinding of soy reduced the particle size from 499 to 315 μm. The reduction in particle size of soy was higher in primary grinding (93%) than that of secondary grinding (37%). In the case of red gram, secondary grinding reduced the particle size from 851 to 254 μm. As in the case of soy, reduction in particle size of red gram was higher in primary grinding (98%) than that of secondary grinding (70%). The results indicated that coarse fractions of soy and red gram were not easily amenable for further reduction.
The average final particle size of red gram (254 μm) was lower than soy (315 μm) at the end of secondary grinding (). About 70% size reduction was achieved with red gram during secondary grinding whereas only 37% size reduction was observed with soy. As in the case of primary grinding, size reduction achieved was higher in red gram compared to soy during secondary grinding (). It is evident once again that the size reduction of grain does not depend only on the hardness. Energy required for secondary grinding increased with grinding duration or in other words fineness, soy displaying higher energy consumption compared to red gram (). Energy consumption in secondary grinding was higher than primary grinding in both soy and red gram supporting the fact that coarse fractions were not easily amenable for grinding.
Two-Stage Grinding
In a continuous grinding process, a larger aperture control sieve is used in the first stage grinder and a smaller sieve is used in the subsequent stage to achieve the desired final particle size. However, in batch type grinding machines, grinding is simply continued till the desired final particle size is achieved. In the present study, a two-stage grinding process () was proposed based on the results obtained in primary and secondary grinding studies employing a batch type mixer grinder.
Single-stage/primary grinding of soy reduced the particle size from 3880 to 255 μm while two-stage grinding reduced the particle size to 183 μm (). The corresponding overall particle size reductions in single-stage and two-stage grinding were 93 and 95%, respectively. Two-stage grinding led to a smaller final particle size compared to single-stage grinding suggesting its suitability for grinding soy.
Figure 4 Selection of grinding process. GT: Gain in two-stage grinding of soy; GS: gain in single-stage grinding of red gram.
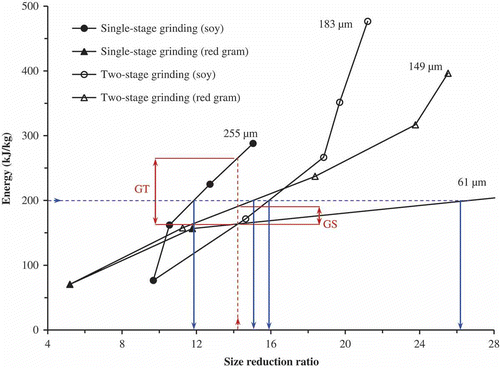
In the case of red gram, the size reduction ratio achieved in single-stage grinding and two-stage grinding were almost the same up to 8 min. Thereafter, reduction ratio showed an increasing trend in single-stage grinding compared to two-stage grinding (). The overall particle size reduction achieved in single-stage grinding (98%) was greater than that of two-stage grinding (96%). In other words, the final average particle size obtained in two-stage grinding (149 μm) was not lower than what was achieved with single-stage grinding (61 μm). This typical behavior of red gram indicated that fines fraction (< 180 μm) of red gram lends easily for further reduction in size compared to soy. The results revealed that a single-stage grinding is desirable for red gram than a two-stage grinding.
Generally a two-stage grinding improves energy efficiency, primarily due to the fact that second stage handles only about half of the initial feed quantity. With the same amount of energy input (200 kJ/kg), single-stage grinding of soy resulted in a size reduction of 12, which increased to 16 with the introduction of two-stage grinding while a size reduction of 26 was achieved in the case of red gram only with single-stage grinding () whereas it was only 15 in the two-stage grinding. The results suggested that a two-stage segregated grinding is suitable for soy which is an energy efficient approach in accordance with the general practice while revealing that such an approach is not desirable for red gram despite being equally hard as that of soy.
Mechanism of Size Reduction
The average particle diameter of red gram after 4 min primary grinding was almost twice that of soy. On the contrary, size reduction in red gram at the end of primary grinding was almost four times higher than soy (). Although this contrasting behavior is intriguing, it made the mechanism of grinding more difficult to understand. and show the photo micrographs of soy and red gram taken from sections perpendicular and parallel to the hilum, respectively. Red gram has higher starch content and starch cells are globular in nature, which are surrounded by cell wall material. Kertesz[Citation17] reported middle lamella of plant tissue is generally composed of pectic substances associated with divalent cations such as calcium and magnesium and proteinaceous material. Both perpendicular ( and ) and parallel ( and ) sections appear similar. They displayed uniformly distributed starch and cell wall structure throughout the cotyledon. In the case of soy, perpendicular section ( and ) shows clear cell wall material consisting of protein bodies and less starch is visible inside the cell wall. While parallel section ( and ) shows an internal structure more like a stack of individual cells one over the other and adjacent cell walls appear to be discontinuous. This arrangement could be easily disturbed with the application of cutting forces facilitating breakage during the initial stage of grinding. However, further rate of size reduction in soy was rather low probably owing to the greater intercellular forces. On the other hand, red gram cell structure is uniform with greater presence of starch bodies resulting in gradual size reduction involving lower intercellular forces. The above analysis suggested that typical internal structure and intercellular forces could play a significant role in the grinding characteristics of individual grains. Indira and Bhattacharya[Citation9] reported that grinding characteristics of legumes depend largely on some inherent properties and not merely on the size or shape of the grain.
In this context, it may be worthwhile to compare the studies reported on cooking of these two grains. During cooking of grains, intercellular material within the middle lamella softens and permits separation of adjacent whole cells.[Citation18] Soy required almost 4-fold higher time to reach a firmness value close to red gram.[Citation19] Gandhi and Bourne[Citation20] reported that soy took almost 5 h to cook in open vessel mainly due to the presence of higher amount of calcium and magnesium present in the cotyledon. Calcium and magnesium present in soy was almost 2–3 times higher than red gram.[Citation15] It could be inferred that intercellular forces between the cells may be higher in soy probably due to higher amount of calcium and magnesium present in the lamella necessitating more intensive cooking. The same analogy might largely hold good for grinding as well, apart from the influence of internal structure of grain.
Energy Assessment and Suitability of Grinding Laws
Energy consumption increased with grinding duration in primary as well as secondary grinding. Further, energy consumed was higher in secondary grinding compared to primary grinding in both soy and red gram at all durations (). Indira and Bhattacharya[Citation9] reported higher energy consumption in fine grinding of unclassified legume flours after coarse grinding. Singh and Goswami[Citation21] also made a similar observation during cryogenic grinding of cumin seed, stating that the energy consumption decreased as control sieve size increased. As discussed earlier, it was difficult to achieve greater size reduction in secondary grinding of soy and red gram compared to primary grinding. In accordance with this fact, higher energy consumption was observed in the case of secondary grinding. Lower values of all the three grinding constants during first 4 min primary grinding of soy, reflected the earlier observation that initial breaking of soy was easier compared to red gram.
Applicability of Kick’s, Rittinger’s, and Bond’s laws was assessed for primary and secondary grinding of soy and red gram. Sharma et al.[Citation13] reported that these laws were better applicable for wet grinding of parboiled rice compared to raw rice indicating the suitability of these laws for hard materials. In the present study, CVs of values of grinding constants were very large (>31%) for primary grinding of soy suggesting that no law was applicable, while Kick’s law and Bond’s work index were found fairly suitable for primary grinding of red gram (). Vishwanathan et al.[Citation14] examined the applicability of these classical dry grinding laws from a different angle and revealed that they were better applicable after the initial break up during wet grinding of soybean. Extending a similar approach in the present study showed that all these three laws were highly applicable for the secondary grinding of soy (CV < 9.6) and to a slightly lesser extent in the case of red gram (CV < 20.9) (). The results once again reaffirm the need to look at biological materials with a difference compared to minerals for which these classical grinding laws were originally proposed.
CONCLUSIONS
The study revealed that hardness measured in terms of crushing strength may not be a reliable indicator while the grinding characteristics of individual grains could depend on the internal structure of the grain and intercellular forces. The amount of calcium and magnesium present in the middle lamella of the grain could be an influencing factor in determining the grinding characteristics. Higher energy consumption during secondary grinding of soy and red gram supported the fact that coarse fractions were not easily amenable for further reduction. Generally a two-stage grinding is expected to improve energy efficiency, but the present results showed that this approach needs to be applied with caution as it depends on the grinding characteristics of individual materials. Classical grinding laws developed for minerals could be applied to soy and red gram with appropriate modification, and applicability of these laws to food grains should be explored further for greater understanding.
NOMENCLATURE
CV | = | Coefficient of variation, dimensionless |
D | = | Equivalent spherical diameter, m |
E | = | Energy consumption, kJ/kg |
En | = | Net energy consumption, kJ/kg |
K | = | Constant, dimensionless |
KK | = | Kick’s constant, kJ/kg |
KR | = | Rittinger’s constant, kJ/(kg-m) |
L | = | Particle size dimension, μm |
L1 | = | Initial particle dimension, μm |
L2 | = | Final particle dimension, μm |
N | = | Constant, dimensionless |
V | = | Volume of single split grain, m3 |
Wi | = | Bond’s work index, kJ/kg |
ACKNOWLEDGMENTS
Vasudeva Singh and S. Umesh Kumar at CFTRI provided valuable suggestions. K.H. Vishwanathan thanks the Council of Scientific and Industrial Research (CSIR) for the award of Senior Research Fellowship.
REFERENCES
- McCabe, W.L.; Smith, J.C.; Harriott, P. Unit Operations of Chemical Engineering, 6th Ed; McGraw-Hill: Singapore, 2001, 965–985.
- Kent, N.L.; Evers, A.D. Technology of Cereals, 4th Ed; Woodhead Publishing: UK, 1994, 259–268.
- Brennan, J.G.; Butters, J.R.; Cowell, N.D.; Lilly, A.E.V. Food Engineering Operations, 2nd Ed; Applied Science Publishers: London, UK, 1976, 61–83.
- Solanki, S.N.; Subramainan, R.; Singh, V.; Ali, S.Z.; Manohar, B. Scope of colloid mill for industrial wet grinding for batter preparation of some Indian snack foods. Journal of Food Engineering 2005, 69, 23–30.
- Murthy, C.T.; Rani, M.; Srinivasa, Rao, P.N. Optimal grinding characteristics of black pepper for essential oil yield. Journal of Food Process Engineering 1999, 22, 161–173.
- Chakkaravarthi, A.; Math, R.G.; Walde, S.G.; Rao, D.G. Grinding characteristics of carrots (Daucus carota, L.). Journal of Food Engineering 1993, 20, 381–389.
- Walde, S.G.; Balswamy, K.; Velu, V.; Rao, D.G. Microwave drying and grinding characteristics of wheat (Triticum aestivum). Journal of Food Engineering 2002, 55, 271–276.
- Velu, V.; Nagender, A.; Prabhakara Rao, P.G.; Rao, D.G. Dry milling characteristics of microwave dried maize grains (Zea mays L.). Journal of Food Engineering 2006, 74, 30–36.
- Indira, T.N.; Bhattacharya, S. Grinding characteristics of some legumes. Journal of Food Engineering 2006, 76, 113–118.
- Earle, R.L. Unit Operations in Food Processing, 1st Ed; Pergamon Oxford: 1966, 284–285.
- Jagtap, P.S.; Subramainan, R.; Singh, V. Influence of soaking on crushing strength of raw and parboiled rice. International Journal of Food Properties 2008, 11, 1–10.
- Fellows, P.J. Food Processing Technology–Principles and Practice, 2nd Ed; Woodhead Publishing: Cambridge, 2000, 99–117.
- Sharma, P.; Chakkaravarthi, A.; Singh, V.; Subramanian, R. Grinding characteristics and batter quality of rice in different wet grinding systems. Journal of Food Engineering 2008, 88, 499–506.
- Vishwanathan, K.H.; Singh, V.; Subramanian, R. Wet grinding characteristics of soybean for soymilk extraction. Journal of Food Engineering 2011, 106, 28–34.
- Gopalan, C.; Rama Sastri, B.V.; Balasubramanian, S.C. Nutritive Value of Indian Foods, National Institute of Nutrition, 2nd Ed; National Institute of Nutrition, ICMR: Hyderabad, 2007, 48–69.
- Vishwanathan, K.H.; Singh, V.; Subramanian, R. Influence of particle size on protein extractability from soybean and okra. Journal of Food Engineering 2011, 102, 240–246.
- Kertesz, Z.I. The Pectic Substances, 1st Ed; Interscience Publishers Inc.: New York, N.Y., 1951.
- Rockland, L.B.; Jones, F.T. Scanning electron microscope studies on dry beans-Effect of cooking on the cellular structure of cotyledons in rehydrated large lima beans. Journal of Food Science 1974, 39, 342–346.
- Pratape, V.M.; Narasimha, H.V. Processing of soybean for use as dhal. Journal of Food Science and Technology 1994, 31, 423–425.
- Gandhi, A.P.; Bourne, M.C. Effect of pre-soaking on the rate of thermal softening of soybeans. International Journal of Food Science and Technology 1991, 26, 117–121.
- Singh, K.K.; Goswami, T.K. Cryogenic grinding of cumin seed. Journal of Food Process Engineering 1999, 22, 175–190.