Abstract
The aim of the present study was to investigate the potential application of a ternary system containing horseradish peroxidase, glucose oxidase, and glucose for the oxidative cross-linking of soybean protein isolate. Protein content, reaction temperature, and original pH were fixed at 30 g/L, 37°C, and 7.0, while suitable glucose, glucose oxidase, horseradish peroxidase addition, and reaction time selected from single factor trials for the one-step treatment were 0.1 mmol, 4.0 U, 200 U/g protein, and 3 h, respectively. In the two-step treatment, glucose, glucose oxidase, and horseradish peroxidase addition were the same, but a reaction time of 1 or 2 h was used for the glucose oxidase or horseradish peroxidase treatment. Two modified soybean protein isolates obtained by the one- and two-step treatment contained some protein polymers, had relative dityrosine content of 414 and 381, respectively, and showed lower in vitro digestibility and less free sulfhydryl than the control soybean protein isolate. During acidification induced by glucono-δ-lactone, the modified soybean protein isolates showed shorter gelation time (57 and 61 versus 72 min), lower loss tangent and gelation point temperature (52.9 and 53.2 versus 62.7°C) but higher final storage modulus than the control soybean protein isolate. The acidified gels prepared from the modified soybean protein isolates also exhibited enhanced hardness, springiness, cohesiveness, and chewiness, and finer microstructural features. The ternary system was capable of treating protein ingredients to improve their gelation properties.
INTRODUCTION
Enzymatic modification, such as proteolysis and cross-linking, is a useful tool to improve functional properties of food proteins. Both transferase- and oxidoreductase-induced cross-linking are potential approaches to obtain new protein ingredients.[Citation1Citation2] Transglutaminase (TGase, EC 2.3.2.13) as one of the transferases is widely used for the cross-linking of casein and soybean proteins.[Citation3Citation4] At the same time, four types of oxidoreductases also have been used by some researchers in their recent studies to cross-link protein substrates, e.g., using tyrosinase for α-lactalbumin, β-lactoglobulin,[Citation5] bovine serum albumin, and β-casein,[Citation6] or using laccase for whey protein isolate,[Citation1Citation7] or using horseradish peroxidase (HRP, EC 1.11.1.7) for α-lactalbumin,[Citation2Citation8] β-lactoglobulin,[Citation1] casein, and soybean proteins,[Citation9] or using glucose oxidase (GOD, EC 1.1.3.4) for milk proteins[Citation10Citation−Citation12] and dough.[Citation13Citation14]
Gelation properties of food proteins can be efficiently modified by the enzymatic cross-linking. When Trichoderma reesei tyrosinase was used to cross-link milk proteins, acid-induced gels prepared thereof had shorter gelation time and a lower final loss tangent.[Citation15] Cross-link of pea protein isolate by TGase led to the heat-induced gels lower gelation point temperature.[Citation16] Cross-linking of wheat gluten by TGase resulted in enhanced textural parameters of the gels.[Citation17] In the above mentioned researches, oxidative cross-linking of food proteins was induced only by one oxidase in most cases. There exists a need to investigate potential application of two or more oxidases for the same purpose. HRP, in the presence of H2O2, is able to cross-link proteins by dityrosine formation,[Citation18] while GOD can catalyze glucose oxidation to produce H2O2 necessary to the cross-linking of food proteins. A ternary system containing HRP, GOD, and glucose theoretically can be applied to cross-link food proteins, i.e., GOD and glucose are used for H2O2 production (first reaction), and HRP and the formed H2O2 are then used for protein cross-linking (coupled second reaction). This ternary system had been used to cross-link caseinate,[Citation19] but has not been applied so far to treat soybean protein isolate (SPI), another widely used protein ingredient in the food industry. A detailed study is suggested to cross-link SPI oxidatively by the ternary system and evaluate functional properties (especially gelation properties) of the obtained product.
In the present study, this ternary system was applied to modify SPI via cross-linking tyrosine residues by a one- and two-step treatment, respectively. Some cross-linking conditions were selected from single factor trials. Gelation properties and in vitro digestibility of two modified SPIs prepared thereof, were characterized by rheological, textural profile analysis, and in vitro digestion, respectively. The aim was to reveal the potential application of the ternary system for soybean proteins to improve their functional (especially gelation) properties.
MATERIALS AND METHODS
Materials and Chemicals
Soybean protein isolate was prepared from defatted soybean flour with a procedure described by Jiang and Zhao,[Citation20] and had a protein content of about 90.7% on dry basis (by the Kjeldahl method). Acrylamide and glycine were obtained from Amresco, Inc. (Solon, OH, USA). Horseradish peroxidase (20 kU/g) was purchased from Shanghai Guoyuan Biotech, Inc. (Shanghai, China). Glucose oxidase (type X- S) prepared from Aspergillus niger (130 kU/g) and trypsin (90 kU/g) were purchased from Sigma-Aldrich Co. (St. Louis, MO, USA). Pepsin (20 kU/g) was purchased from Hui Shi Biochem Reagent Co. (Shanghai, China). Other chemicals used were of analytical grade. Re-distilled water was used to prepare all buffers and solutions.
Oxidative Cross-Linking of Soybean Protein Isolate
SPI solution was adjusted to pH 7.0 by 0.2 mol/L NaOH solution, and denaturalized in a water bath at 92°C for 10 min. A reaction mixture containing SPI (final protein content of 30 g/L), HRP, GOD, and glucose was incubated at 37°C with gentle agitation to carry out the one-step treatment. After the reaction, the mixture was heated at 85°C for 10 min, diluted into 0.5 g/L by 0.2 mol/L carbonate buffer (pH 9.5), and detected for relative fluorescence intensity (i.e., relative dityrosine content) as per the method of Davies et al.[Citation21] in a Hitachi F-4500 fluorescence spectrometer (Tokyo, Japan). A slit width of 0.5 nm and wavelengths of 320 nm (excitation) or 410 nm (emission) were used in the measurement.
Single factor trials were applied to select suitable concentration of HRP, GOD, and glucose or reaction time for the one-step treatment. Relative dityrosine content of the modified SPIs was used as a response of the carried out cross-linking. Investigated HRP, GOD, glucose addition, and reaction time were in the range of 100−300 U, 1.0−8.0 U, 0.01−0.4 mmol/g protein, and 0.5−4 h, respectively. By using the selected conditions, a modified SPI (MSPI-1) was bulk prepared, freeze-dried, and evaluated for its properties.
A two-step treatment was carried out to obtain another modified SPI (MSPI-2). SPI was first treated by the added GOD and glucose for 1 h, and then by addition of HRP for another 2 h. The used addition levels of HRP, GOD, and glucose and other conditions were the same as those used in the one-step treatment. At the same time, a control SPI was treated as MSPI-1 but without HRP, GOD, and glucose addition.
Sodium Dodecyl Sulfate-Polyacrylamide Gel Electrophoresis Analysis
Sodium dodecyl sulfate-polyacrylamide gel electrophoresis (SDS-PAGE) analysis was carried out according to the method of Laemmli[Citation22] by using 13% (w/v) separating gels (pH 8.8) and 4% (w/v) stacking gels (pH 6.8). The sample solutions were prepared at a protein level of 10 g/L, and then diluted by sample buffer of pH 6.8 to another protein level of 5 g/L (with mercaptoethanol addition). The diluted sample solutions of 10 μL were applied for the analysis. Protein markers having molecular weights (MW) of 14.4−97.4 kDa were used at 10 g/L.
Evaluation of In vitro Digestibility and Sulfhydryl or Disulfide Bonds Content
In vitro digestibility was evaluated by the method of Yin et al.[Citation23] with some modifications. In the one-step digestion, a sample solution (20 g protein/L) of 5 mL was adjusted into pH 2.0 by 1 mol/L HCl solution. One milliliter of the pepsin solution containing 3 mg of pepsin powder was added into the sample solution. The mixture was diluted to a volume of 10 mL by water, and incubated at 37°C for 2 h. The digestion was stopped by adding 200 g/L trichloroacetic acid (TCA) of 10 mL. In the two-step digestion, the prepared sample solution was first digested by the added pepsin at 37°C for 1 h and then heated at 90°C for 5 min. The obtained digests were evaporated by nitrogen blowing to dryness, resolved in phosphate buffer (0.2 mol/L, pH 8.0) of 9 mL, and digested at 37°C for 1 h by adding 9 mg trypsin powder dissolved in the phosphate buffer of 1 mL. The digestion was also stopped by adding the TCA solution. The obtained digests were centrifuged at 10,000× g for 20 min. The collected supernatants were diluted by water (three-fold volumes) and measured for their absorbance at 280 nm in an UV-2401 PC spectrophotometer (Shimadzu Corporation, Kyoto, Japan). Sulfhydryl (SH) content was assayed by using the methods from Chan and Wasserman[Citation24] and Thannhauser et al.,[Citation25] but 8 mol/L urea solution was replaced by 6 mol/L guanidine hydrochloride solution. Disulfide bonds (SS) content was calculated by half of the difference between total and free SH content.
Evaluation of Gelation and Rheological Properties
Acid-induced gels were prepared as the described procedure[Citation26Citation27] with minor modification. Sample solutions (40 g protein/L) were adjusted to pH 6.8 by 0.2 mol/L NaOH solution with a final volume of 25 mL, acidified by adding glucono-δ-lactone (GDL) granules (0.15 g/g protein) and mixed thoroughly for 2 min. The GDL addition was sufficient to induce a pH drop to 5 in approximately 2 h and to result in a final pH of about 4 after 5–6 h. The prepared gels were congealed at 4°C for 24 h. Texture profile analysis (TPA) was carried out by using a TA-XT 2 texture analyzer (Stable Micro Systems Ltd., Surrey, UK) with a cylindrical probe (p/0.5). Gel portions (25 mm in diameter and 16 mm in height) were tested by two continuous compressions. The parameter settings were: pre-test speed, 1.0 mm/s; test speed, 1.0 mm/s; post-test speed, 1.0 mm/s; strain, 50%; interval between two compressions, 2 s. Hardness, cohesiveness, adhesiveness, and springiness were calculated by using the software accompanying the analyzer.
Rheological properties of the sample solutions during acidification were monitored by time and temperature sweeps as per the method of Li et al.[Citation26] in a Bohlin Gemini II Rheometer (Malvern Instruments Limited, Worcestershire, UK), which was fitted with a 60-mm-diameter parallel plate system with a 1.0-mm gap. Sample solutions (40 g protein/L) were placed onto the parallel plate system exactly after 2 min of GDL addition. Temperature sweeps were carried out by heating the solutions from 25 to 85°C at a rate of 0.5°C/min. After reaching the final temperature (85°C), the solutions were held at this temperature for 1 min. The measurement was performed at a fixed frequency of 1 Hz and strain of 1%. Time sweeps were performed with a frequency of 1 Hz at 40°C, and the measurements were taken every 40 s for 2 h. After that, the effect of time scale of the applied strain on rheological properties of the gels was assayed by varying the frequency from 0.01 to 100 Hz with 1% strain at 40°C. Storage modulus and loss tangent of the sample solutions were recorded during gel formation. Final storage modulus and loss tangent were defined as the storage modulus and loss tangent values observed after 2 h of GDL addition, at which rheological behavior of sample dispersion was no longer that of a liquid but a gel as defined by the value of storage modulus >10 Pa.[Citation28Citation29]
Microstructure Observation
Microstructure of the acidified gels was observed under scanning electron microscope (SEM), as the method of Kong et al.[Citation3] with some modifications. The gels were cut into 4 × 4 × 3 mm3 cubes, and fixed in 2.5% glutaraldehyde solution in 0.1 mol/L phosphate buffer (pH 6.8) at 4°C for 24 h. The fixed specimens were cut into chips by using a razor blade from the interior of the gel cubes; dipped in the buffer for 10 min; rinsed with cold buffer three times; dehydrated for 15 min each in graded solutions of ethanol, ethanol/tert-butyl alcohol, and tert-butyl alcohol; and immediately frozen for 48 h by immersing in liquid nitrogen. The dried specimens were mounted on aluminum SEM stubs using a carbon-based tape, coated with gold in a Hummer VI sputtering system (Matsushita Electric Industrial Co., Osaka, Japan), examined and photographed by a S-3400N scanning electron microscope (Hitachi, Tokyo, Japan) with an accelerating voltage of 5 kV.
Statistical Analysis
All experiments and analyses were carried out three times, and the data were expressed as means or means ± standard deviations. Multiple comparison analysis was performed by using SPSS 13.0 software (SPSS Inc., Chicago, IL, USA). The least significant differences (LSD) test was used to describe means with 95% confidence.
RESULTS AND DISCUSSION
Oxidative Cross-Linking of Soybean Protein Isolate
The impacts of glucose, GOD, HRP addition, and reaction time on the oxidative cross-linking of SPI via a one-step treatment are revealed in . The data indicate that as SPI was treated by the ternary system, relative fluorescence intensity (i.e., relative dityrosine content) of the modified SPI was enhanced significantly (P < 0.05). It reveals that the ternary system induced dityrosine formation (i.e., oxidative cross-linking) of SPI. The four investigated factors showed impact on the cross-linking. Glucose addition should be a key factor, as it governed H2O2 amount formed in the system and thus the cross-linking of SPI. The results in revealed that glucose added at a level of 0.1 mmol/g protein led to the greatest SPI cross-linking (the highest relative fluorescence intensity). GOD at the level of 4.0 U/g protein resulted in the most SPI cross-linking (). The data in indicate that HRP at the level of 300 U/g protein seemed to be the best addition. Relative fluorescence intensity of the modified SPI by HRP addition at 300 U/g protein was 433, while that by HRP addition at 200 U/g protein was 421. Increase of HRP addition by 50% only yielded increased cross-linking by about 3%. Thus, the suitable HRP addition was 200 U/g of protein. The results in also show that a reaction time of 3 h was enough or suitable.
Figure 1 Impacts of glucose, glucose oxidase, horseradish peroxidase addition (a−c), and reaction time (d) on relative fluorescence intensity of the modified soybean protein isolates. Different capital letters above the columns indicate that one-way ANOVA of the mean values was significantly different (P < 0.05).
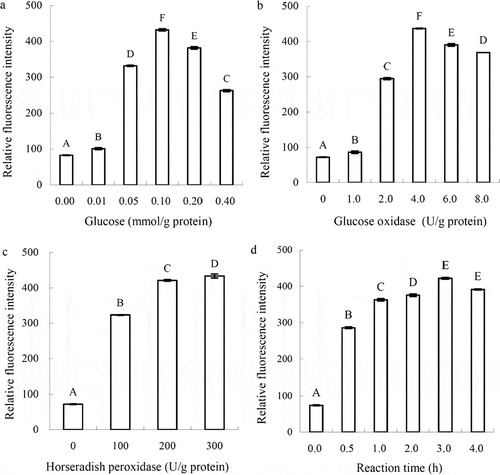
The safeguarded suitable conditions for SPI cross-linking by one-step treatment were thus selected as glucose, GOD, and HRP addition of 0.1 mmol, 4.0 U, 200 U/g protein, and a reaction time of 3 h, while protein concentration, reaction temperature, and original pH were set at 30 g/L, 37°C, and 7.0, respectively. With these conditions, a modified SPI (MSPI-1) with relative dityrosine content of 414 was prepared thereof. Another modified SPI (MSPI-2) having relative dityrosine content of 381 was also prepared by the two-step treatment. One-step treatment was more efficient to cross-link SPI than two-step treatment, as a different reaction procedure and reaction time were applied in the two-step treatment.
HRP can cross-link water-soluble soybean proteins in the presence of H2O2.[Citation9] Three previous researches showed that HRP and H2O2 at 280 U and 0.19 mmol/g protein,[Citation26] HRP and H2O2 at 283.8 U and 0.19 mmol/g protein,[Citation31] or Coprinus Cinereus peroxidase and H2O2 at 2 kU and 0.3 mmol/g protein[Citation32] could cross-link casein or α-lactalbumin. Hiller and Lorenzen[Citation10Citation−Citation12] also applied lactoperoxidase and H2O2 at 4.8−47.5 kU and 1.8−18 mmol/g protein, respectively, to cross-link caseinate, whey protein isolate, and total milk proteins. These researches provided support to the present study, i.e., the applied ternary system also could induce SPI cross-linking. Takasaki et al.[Citation33] had treated wheat gluten with peroxidase and H2O2 at 30 U and 0.47 mmol/g protein for 4 h at 28°C, and found that dityrosine cross-linking increased with H2O2 and peroxidase plus H2O2 level. Hiller and Lorenzen[Citation12] treated yoghurt milk by lactoperoxidase at 8 kU/g protein for 2 h at 40°C, and found that polymer content increased by 3.3-fold when H2O2 was added at 0.24 μmol/L. Compared to these mentioned researches, the present study applied similar reaction temperature, but lower enzyme or H2O2 addition, and shorter reaction time.
Electrophoresis Analysis of Two Modified Soybean Protein Isolates
Electrophoretic analysis results are given in to show the peptide bands of the assayed protein samples. MSPI-1 and MSPI-2 contained some protein polymers with MW greater than 97.4 kDa. These protein polymers did not find in the control SPI. It reveals that some protein molecules in SPI were cross-linked during the treatment, resulting in the formation of these protein polymers. The peptide profiles of MSPI-1 were not different to those of MSPI-2 by naked eyes observation, as relative dityrosine content of MSPI-1 was only 9% higher than that of MSPI-2 (414 versus 381).
Figure 2 Electrophoretic profiles of soybean protein isolate (SPI), two cross-linked SPIs by one- and two-step treatment (MSPI-1 and MSPI-2) under reducing conditions. Lane M, standard protein markers; Lanes 1−3, SPI, MSPI-1, and MSPI-2, respectively.
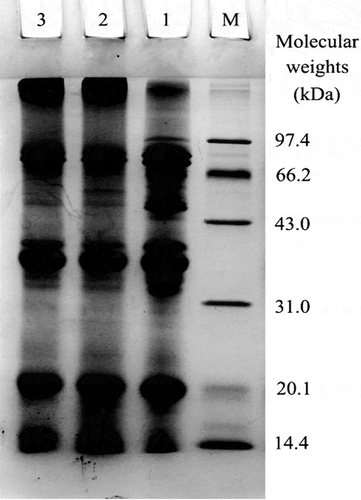
Electrophoresis analysis had been used to show Trichoderma reesei tyrosinase-induced cross-linking of milk proteins, which was reflected by the weakened casein bands and new formed protein polymers of higher MW,[Citation15] or to show laccase-induced cross-linking of β-lactoglobulin.[Citation34] Similarly, SDS-PAGE analysis in the present study also directly revealed the cross-linking of SPI induced by the ternary system.
In vitro Digestibility, Sulfhydryl, or Disulfide Bonds Content of Two Modified Soybean Protein Isolates
The analysis results () revealed that TCA-soluble nitrogen released from SPI, MSPI-1, and MSPI-2 into the digests (reflected by the measured absorbance) was 0.488, 0.398, and 0.397 (peptic-digestion), or 0.793, 0.773, and 0.704 (peptic- and tryptic-digestion), respectively, indicating that the applied cross-linking had some adverse effect on in vitro digestibility of SPI, leading to MSPI-1 and MSPI-2 decreased digestibility of 18% (or 3−11%) in peptic-digestion (or peptic- and tryptic-digestion). Cross-linked caseinate had lower in vitro digestibility due to protection of peptide bonds from proteolysis.[Citation35] Tantoush et al.[Citation34] reported that polymerization of β-lactoglobulin by phenol oxidase damaged its digestibility, while Monogioudi et al.[Citation36] revealed that fully cross-linked β-casein had lower digestibility than the counterpart. The present study shared a similar conclusion to the three reported researches.
Table 1 In vitro digestibility, sulfhydryl (SH), and disulfide bonds (SS) content of soybean protein isolate (SPI) and two cross-linked SPIs
Free, total SH content as well as the calculated disulfide bonds (SS) content of each evaluated sample are also listed in . Free SH content of MSPI-1 and MSPI-2 was significantly lower than the control SPI (4.43−5.42 versus 6.39 μmol/g protein); at the same time, SS content of MSPI-1 or MSPI-2 (36.51 or 36.34 μmol/g protein) was higher than that of the control SPI (35.45 μmol/g protein). These facts showed that the applied ternary system also induced SPI oxidation, and converted some free SH into SS. Exposed SH and SS content of soybean protein isolate were 6.64 and 37.4 μmol/g protein, respectively.[Citation37] Oxidative cross-linking of water soluble protein fractions yielded an increase in SS content,[Citation38] due to the oxidation of free SH.[Citation39] The present result was consistent to these findings.
Gelation Properties of the Modified Soybean Protein Isolates
The investigated gelation properties of MSPI-1 and MSPI-2 are depicted in . Time sweep tests () showed that MSPI-1 and MSPI-2 had gelation time of 57 and 61 min, respectively, 20 or 15% shorter than that of the control SPI (72 min). After gelation, MSPI-1 conferred the dispersion of the highest storage modulus while the control SPI resulted in the dispersion of the lowest one. Changed profiles of storage modulus reflect gelation process and development of the formed gels.[Citation33] Cross-linked protein chains produce larger and more compact protein aggregates, so storage modulus may increase with the increased cross-linking extent,[Citation40] as a small increase in the number of covalent cross-links can result in increased storage modulus.[Citation41] Dityrosine formation in MSPI-1 and MSPI-2 thus led to the acidified gels higher storage modulus. Cross-linking of milk by TGase resulted in a decrease in gelation time, especially a prolonged treatment time.[Citation41] Treatment of milk by Trichoderma reesei tyrosinase yielded shorter gelation time (230 versus 288 min).[Citation15] Similar results were also found in other researches.[Citation42−Citation44] It was considerable that the much cross-linked MSPI-1 had the shortest gelation time but the control SPI had the longest one.
Figure 3 Storage modulus and loss tangent changes during time sweeps (a and b), final storage modulus (c), and storage modulus changes during temperature sweeps (d) of control soybean protein isolate (SPI) and two cross-linked SPIs by one- and two-step treatment (MSPI-1 and MSPI-2) during acidification.
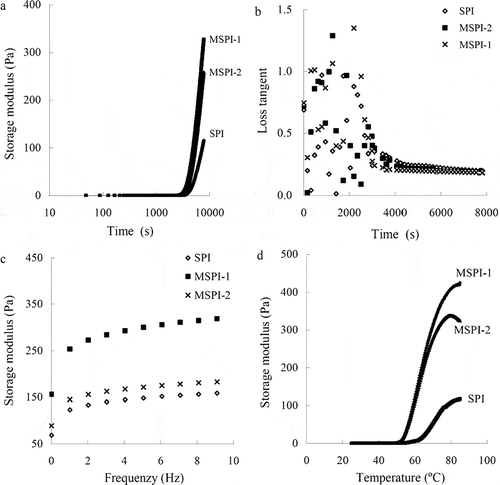
The data in showed that the values of loss tangent of three protein samples first increased up to a maximum and then decreased as sweep time passed. The values of loss tangent of MSPI-1, MSPI-2, and SPI were finally up to 0.183, 0.188, and 0.201, respectively, after a gelation time of 2 h. Loss tangent is a measure of the dynamic character of protein-protein bonds in the gel network,[Citation45] and reflects rearrangement potential of the initial gel network.[Citation46] Higher loss tangent is responsible for less elasticity of the gels,[Citation45] while a lower one indicates decreased relaxation behavior of the protein-protein bonds, and that the type of the bonds forming the gel is altered and the system is transformed towards viscoelastic solid state.[Citation47] A lower final loss tangent was also observed for Trichoderma reesei tyrosinase-treated acidified milk gels.[Citation15] The present result was consistent with this reported result, showing that the carried out oxidative cross-linking impacted the viscoelastic properties of SPI.
Typical frequency sweeps were conducted for MSPI-1, MSPI-2, and control SPI gels after a gelation time of 2 h (). The measured storage moduli were nearly dependent on the applied frequency, reflecting viscoelastic natures of the evaluated gels. Not surprising, the gels prepared from MSPI-1 or control SPI showed the highest or lowest value of storage moduli. Evaluation results about temperature sweeps are given in . Gelation point temperature of MSPI-1, MSPI-2, and control SPI were about 52.9, 53.2, and 62.7°C, while corresponding storage moduli were 425, 325, and 118 Pa, respectively, i.e., the carried out cross-linking conferred two modified SPIs lower temperature requirement and higher ability to form an elastic gel network. The research from Truong et al.[Citation48] indicated that gelation point temperature of whey protein solutions treated by TGase was slightly decreased. Another one from Shand et al.[Citation16] also showed that gelation point temperature of native pea protein isolate was 71°C, while that of a cross-linked counterpart by TGase was 65°C. The present result shared consistence to the two reported results.
Texture and Microstructure of the Acidified Gels from Two Modified Soybean Protein Isolates
The detected textural parameters of the acidified gels prepared from the control SPI, MSPI-1, and MSPI-2 are listed in . The data showed that oxidative cross-linking of SPI conferred greater hardness, springiness, cohesiveness, and chewiness on the acidified gels, especially when one-step treatment was used.
Table 2 Textural profile analysis of the acid-induced gels prepared from soybean protein isolate (SPI) and two cross-linked SPIs
Gel hardness is related to cross-linking extent of network structure,[Citation49] as covalent cross-linking greatly contributes to gel hardness. Treatment of wheat gluten by TGase resulted in the gels increase of 18−130% in hardness, cohesiveness, springiness, and chewiness.[Citation17] A similar result was found by Lucey et al. for the acidified casein gels.[Citation47] The higher the cross-linking extent was, the greater would be the gel hardness and cohesiveness.[50] Cross-linking of porcine blood plasma by TGase caused an increase in gel hardness and springiness,[Citation51] while cross-linking of SPI by TGase yielded an increase of 13.7 or 5.46% in hardness or chewiness.[Citation27] The present assaying results shared similarity to these reported results.
Microstructural characteristics of the prepared acidified gels are shown in . The network of the gels prepared from the control SPI was characterized as a coarser and looser structure with big and irregular holes (). However, the gels prepared from MSPI-1 and MSPI-2 showed an increase in pore density and a more uniform network ( and ). Microstructural changes are related to inter- and intra-molecular cross-linking. Dough treated by GOD and TGase had a continuous and closed gluten network.[Citation52] TGase treatment yielded the prepared yogurt or tofu more compact and uniform microstructure.[Citation50Citation53] Treatment of casein by HRP also led to the acidified gels being a more compact and homogeneous microstructure.[Citation31] The present result was consistent with these mentioned findings.
CONCLUSIONS
A ternary system consisting of horseradish peroxidase, glucose oxidase, and glucose could cross-link soybean protein isolate (SPI) via dityrosine formation, resulting in the treated SPI lowered in vitro digestibility and especially modified gelation properties, including higher final storage modulus, lower loss tangent, shorter gelation time, and lower gelation point temperature. The one-step treatment led to much SPI cross-linking than the two-step treatment, and conferred the modified SPI higher relative dityrosine content and less free sulfhydryl. The applied cross-linking also yielded the acidified gels enhanced textural parameters and finer microstructural features. The ternary system is applicable for treating other protein ingredients for functionality modification.
ACKNOWLEDGMENTS
The authors wish to thank the anonymous reviewers and the editors for their valuable suggestions and work put into this article.
FUNDING
This study was funded by the National High Technology Research and Development Program (“863” Program) of China (Project No. 2013AA102205).
REFERENCES
- Færgemand, M.; Otte, J.; Qvis, K.B. Cross-linking of whey proteins by enzymatic oxidation. Journal of Agricultural and Food Chemistry 1998, 46 (4), 1326–1333.
- Heijnisa, W.H.; Wierenga, P.A.; Janssen, A.E.M.; van-Berkel, W.J.H.; Gruppen, H. In-line quantification of peroxidase-catalyzed cross-linking of -lactalbumin in a microreactor. Journal of Chemical Engineering 2010, 157 (2), 189–193.
- Jiang, S.J.; Zhao, X.H. Cross-linking and glucosamine conjugation of casein by transglutaminase and the emulsifying property and digestibility in vitro of the modified product. International Journal of Food Properties 2012, 15 (6), 1286–1299.
- Zhang, Y.N.; Zhao, X.H. Study on the functional properties of soybean protein isolate cross-linked with gelatin by microbial transglutaminase. International Journal of Food Properties 2013, 16 (6), 1257–1270.
- Partanen, R.; Torkkeli, M.; Hellman, M.; Permi, P.; Serimaab, R.; Bucherta, J.; Mattinen, L.M. Loosening of globular structure under alkaline pH affects accessibility of β-lactoglobulin to tyrosinase-induced oxidation and subsequent cross-linking. Enzyme and Microbial Technology 2011, 49 (2), 131–138.
- Mattinen, M.L.; Lantto, R.; Selinheimo, E.; Kruus, K.; Buchert, J. Oxidation of peptides and proteins by Trichoderma reesei and Agaricus bisporus tyrosinases. Journal of Biotechnology 2008, 133 (3), 395–402.
- Ma, H.; Forssell, P.; Partanen, R.; Buchert, J.; Boer, H. Improving laccase catalyzed cross-linking of whey protein isolate and their application as emulsifiers. Journal of Agricultural and Food Chemistry 2011, 59 (4), 1406–1411.
- Oudgenoeg, G.; Hilhorst, R.; Piersma, S.R.; Boeriu, C.G.; Gruppen, H.; Hessing, M.; Voragen, A.G.J.; Laane, C. Peroxidase-mediated cross-linking of a tyrosine-containing peptide with ferulic acid. Journal of Agricultural and Food Chemistry 2001, 49 (5), 2503–2510.
- Matheis, G.; Whitaker, J.R. Peroxidase-catalyzed cross linking of proteins. Journal of Protein Chemistry 1984, 3 (1), 35–48.
- Hiller, B.; Lorenzen, P.C. Functional properties of milk proteins as affected by enzymatic oligomerisation. Food Research International 2009, 42 (8), 899–908.
- Hiller, B.; Lorenzen, P.C. Optimization of enzymatic oligomerization reaction conditions via ceteris-paribus approach. Food Research International 2011, 44 (9), 3118–3122.
- Hiller, B.; Lorenzen, P.C. Properties of set-style skim milk yoghurt as affected by an enzymatic or Maillard reaction induced milk protein oligomerisation. LWT–Food Science and Technology 2011, 44 (4), 811–819.
- Gujral, H.S.; Rosell, C.M. Improvement of the breadmaking quality of rice flour by glucose oxidase. Food Research International 2004, 37 (1), 75–81.
- Renzetti, S.; Courtin, C.M.; Delcour, J.A.; Arendt, E.K. Oxidative and proteolytic enzyme preparations as promising improvers for oat bread formulations: rheological, biochemical and microstructural background. Food Chemistry 2010, 119 (4), 1465–1473.
- Ercili-Cura, D.; Lille, M.; Partanen, R.; Kruus, K.; Buchert, J.; Lantto, R. Trichoderma reesei tyrosinase on rheology and microstructure of acidified milk gels. International Dairy Journal 2010, 20 (12), 830–837.
- Shand, P.J.; Ya, H.; Pietrasik, Z.; Wanasundara, P.K.J.P.D. Transglutaminase treatment of pea proteins: Effect on physicochemical and rheological properties of heat-induced protein gels. Food Chemistry 2008, 107 (2), 692–699.
- Wang, J.S.; Zhao, M.M.; Yang, X.Q.; Jiang, Y.M.; Chun, C. Gelation behavior of wheat gluten by heat treatment followed by transglutaminase cross-linking reaction. Food Hydrocolloids 2007, 21 (2), 174–179.
- Stahmann, M.A.; Spencer, A.K.; Honold, G.R. Cross linking of proteins in vitro by peroxidase. Biopolymers 1977, 16 (6), 1307–1318.
- Chang, C.H.; Zhao, X.H. In vitro digestibility and rheological properties of the caseinates treated by an oxidative system containing horseradish peroxidase, glucose oxidase and glucose. International Dairy Journal 2012, 27 (1–2), 47–52.
- Jiang, S.J.; Zhao, X.H. Transglutaminase-induced cross-linking and glucosamine conjugation in soybean protein isolates and its impacts on some functional properties of the products. European Food Research and Technology 2010, 231 (5), 679–689.
- Davies, K.J.; Delsignore, M.E.; Lin, S.W. Protein damage and degradation by oxygen radicals. II. Modification of amino acids. Journal of Biological Chemistry 1987, 287 (46), 9902–9907.
- Laemmli, U.K. Cleavage of structural proteins during the assembly of the head of bacteriophage T4. Nature 1970, 227 (5259), 680–685.
- Yin, S.W.; Tang, C.H.; Cao, J.S.; Hu, E.K.; Wen, Q.B.; Yang, X.Q. Effects of limited enzymatic hydrolysis with trypsin on the functional properties of hemp (Cannabis sativa L.) protein isolate. Food Chemistry 2008, 106 (3), 1004–1013.
- Chan, K.Y.; Wasserman, B.P. Direct colorimetric assay of free thiol groups and disulfide bonds in suspensions of solubilized and particulate cereal proteins. Cereal Chemistry 1993, 70 (1), 22–26.
- Thannhauser, T.W.; Konishi, Y.; Scheraga, H.A. Sensitive quantitative analysis of disulfide bonds in polypeptides and proteins. Analytical Biochemistry 1984, 138 (1), 181–188.
- Li, F.; Kong, X.Z.; Zhang, C.M.; Hua, Y.F. Gelation behaviour and rheological properties of acid-induced soy protein-stabilized emulsion gels. Food Hydrocolloids 2012, 29 (2), 347–355.
- Li, L.Y.; Easa, A.M.; Liong, M.T.; Tan, T.C.; Foo, W.T. The use of microbial transglutaminase and soy protein isolate to enhance retention of capsaicin in capsaicin-enriched layered noodles. Food Hydrocolloids 2013, 30 (2), 495–503.
- Abbasi, S.; Dickinson, E. Influence of sugars on higher-pressure induced gelation of skim milk dispersions. Food Hydrocolloids 2001, 15 (3), 315–319.
- Campbell, L.J.; Gu, X.; Dewar, S.J.; Euston, S.R. Effects of heat treatment and glucono-δ-lactone-induced acidification on characteristics of soy protein isolate. Food Hydrocolloids 2009, 23 (2), 344–351.
- Kong, X.Z.; Li, X.H.; Wang, H.J.; Hua, Y.F.; Huang, Y.R. Effect of lipoxygenase activity in defatted soybean flour on the gelling properties of soybean protein isolate. Food Chemistry 2008, 106 (3), 1093–1099.
- Li, J.W.; Zhao, X.H. Oxidative cross-linking of casein by horseradish peroxidase and its impacts on emulsifying properties and the microstructure of acidified gel. African Journal of Biotechnology 2009, 8 (20), 5508–5515.
- Steffensen, C.L.; Mattinen, M.L.; Andersen, H.J.; Kruus, K.; Buchert, J.; Nielsen, J.H. Cross-linking of tyrosine-containing peptides by hydrogen peroxide-activated Coprinus Cinereus peroxidase. European Food Research and Technology 2008, 227 (1), 57–67.
- Takasaki, S.; Kato, Y.; Murata, M.; Homma, S.; Kawakishi, S. Effects of peroxidase and hydrogen peroxide on the dityrosine formation and the mixing characteristics of wheat-flour dough. Bioscience, Biotechnology, and Biochemistry 2005, 69 (9), 1686–1692.
- Tantoush, Z.; Apostolovic, D.; Kravic, B.; Prodic, I.; Mihajlovic, L.; Stanic-Vucinic, D.; Velickovic, T.C. Green tea catechins of food supplements facilitate pepsin digestion of major food allergens, but hampers their digestion if oxidized by phenol oxidase. Journal of Functional Foods 2012, 4 (3), 3–11.
- Roos, N.; Lorenzen, P.C.; Sick, H.; Schrezenmeir, J.; Schlimme, E. Cross-linking by transglutaminase changes neither the in vitro proteolysis nor the in vivo digestibility of caseinate. Kieler Milchwirtschaftliche Forschungsberichte 2003, 55 (4), 261–276.
- Monogioudi, E.; Faccio, G.; Lille, M.; Poutanen, K.; Buchert, J.; Mattinen, M.L. Effect of enzymatic cross-linking of β-casein on proteolysis by pepsin. Food Hydrocolloids 2011, 25 (1), 71–81.
- Shen, L.; Tang, C.H. Microfluidization as a potential technique to modify surface properties of soy protein isolate. Food Research International 2012, 48 (1), 108–118.
- Rasiah, I.A.; Sutton, K.H.; Low, F.L.; Lin, H.M.; Gerrard, J.A. Crosslinking of wheat dough proteins by glucose oxidase and the resulting effects on bread and croissants. Food Chemistry 2005, 89 (3), 325–332.
- Yin, S.W.; Tang, C.H.; Wen, Q.B.; Yang, X.Q.; Li, L. Functional properties and in vitro trypsin digestibility of red kidney bean (Phaseolus vulgaris L.) protein isolate: Effect of high-pressure treatment. Food Chemistry 2008, 106 (3), 1004–1013.
- Kuraishi, C.; Yamazaki, K.; Susa, Y. Transglutaminase: Its utilization in the food industry. International Journal of Food Properties 2001, 17 (2), 221–246.
- Anema, S.G.; Lauber, S.; Lee, S.K.; Henle, T.; Klostermeyer, H. Rheological properties of acid gels prepared from pressure- and transglutaminase-treated skim milk. Food Hydrocolloids 2005, 19 (5), 879–887.
- Myllärinen, P.; Buchert, J.; Autio, K. Effect of transglutaminase on rheological properties and microstructure of chemically acidified sodium caseinate gels. International Dairy Journal 2007, 17 (7), 800–807.
- Schorsch, C.; Carrie, H.; Norton, I.T. Cross-linking casein micelles by a microbial transglutaminase: Influence of cross-links in acid-induced gelation. International Dairy Journal 2000, 10 (8), 529–539.
- Song, F.; Zhang, L.M. Enzyme-catalyzed formation and structure characteristics of a protein-based hydrogel. Journal of Physical Chemistry 2008, 112 (44), 13749–13755.
- Gu, X.; Campbell, L.J.; Euston, S.R. Influence of sugars on the characteristics of glucono-δ-lactone-induced soy protein isolate gels. Food Hydrocolloids 2009, 23 (2), 314–326.
- Ercili-Cura, D.; Lille, M.; Legland, D.; Gaucel, S.; Poutanen, K.; Partanen, R.; Lantto, R. Structural mechanisms leading to improved water retention in acid milk gels by use of transglutaminase. Food Hydrocolloids 2012, 30 (1), 419–427.
- Lucey, J.A.; Van-Vliet, T.; Grolle, K.; Geurts, T.; Walstra, P. Properties of acid casein gels made by acidification with glucono-δ-lactone. 2. Syneresis, permeability and microstructural properties. International Dairy Journal 1997, 7 (6–7), 389–397.
- Truong, V.D.; Clare, D.A.; Catignani, G.L.; Swaisgood, H.E. Cross-linking and rheological changes of whey proteins treated with microbial transglutaminase. Journal of Agricultural and Food Chemistry 2004, 52 (5), 1170–1176.
- Wang, C.H.; Damodaran, S. Thermal gelation of globular proteins: Weight-average molecular weight dependence of gel strength. Journal of Agricultural and Food Chemistry 1990, 38 (5), 1157–1163.
- Tang, C.H.; Li, L.; Wang, J.L.; Yang, X.Q. Formation and rheological properties of ‘cold-set’ tofu induced by microbial transglutaminase. LWT–Food Science and Technology 2007, 40 (4), 579–586.
- Saguer, E.; Fort, N.; Parés, D.; Toldra, M.; Carretero, C. Improvement of gelling properties of porcine blood plasma using microbial transglutaminase. Food Chemistry 2007, 101 (1), 49–56.
- Eugenia-Steffolani, M.; Ribotta, P.D.; Pérez, G.T.; León, A.E. Effect of glucose oxidase, transglutaminase, and pentosanase on wheat proteins: Relationship with dough properties and bread-making quality. Journal of Cereal Science 2010, 51 (3), 366–373.
- Şanlı, T.; Sezgin, E.; Deveci, O.; Şenel, E.; Benli, M. Effect of using transglutaminase on physical, chemical and sensory properties of set-type yoghurt. Food Hydrocolloids 2011, 25 (6), 1477–1481