Abstract
The present study was undertaken to determine the physicochemical, rheological, and thermal properties of six types of Tunisian honey samples from various floral origins (eucalyptus, orange, thyme, mint, rosemary, and horehound). All the honey samples exhibited non-Newtonian behavior at a shear rate ranging between 0.01 and 500 s–1, with the highest levels of viscosity (µ) being observed for thyme, followed by eucalyptus, rosemary, mint, orange, and horehound honeys, respectively. The effect of temperature on the dynamic viscosity of the samples followed an Arrhenius-like pattern, with activation energy values ranging from 21.23 to 34.91 kJ/mol. The results from oscillatory rheology analysis also revealed that the loss modulus predominated over the storage one in the whole frequency range. As determined by differential scanning calorimetry, the glass transition (Tg) and melting temperatures of the Tunisian honey samples varied between –41.55 and –47.06 °C and between 197.9 and 221.1°C depending on their sugar compositions, respectively.
INTRODUCTION
Honey, one of the oldest and most traditional sweetening foods, has been reported to contain about 200 substances.[Citation1] This natural product is essentially a concentrated aqueous solution of different carbohydrates, including fructose, glucose, maltose, sucrose, and other oligo- and polysaccharides. Fructose and glucose are the major sugars of almost all honeys.[Citation2] During the honey digestion process, the principal carbohydrates are transported into the blood stream and utilized for energy requirements by the human body. Honey has also been reported to serve as a source of natural antioxidants, which play beneficial roles in the prevention or alleviation of several human health disorders and diseases, including heart and cancer diseases, immune system deficiencies, age-related cataracts, and various inflammatory conditions.[Citation3] The dietary contributions of those minor components to the recommended daily intake are marginal. However, their importance with respect to nutrition lies in their physiological effects, since they display several health-related properties.[Citation4] Minerals and other trace elements play key roles in the biomedical activities associated with honey. They have a wide range of known biological functions, including the maintenance of intracellular oxidative balance.[Citation5]
The quality characteristics of honey are mainly determined by sensorial, physicochemical, thermal, and rheological properties.[Citation6] The literature presents various studies on the rheological characteristics of honey from various botanical and geographical regions in the world. However, little data is currently available on the rheological and thermal properties of honey from Tunisian origins. The physical properties of honey samples have also attracted increasing attention in academic as well as industrial research.[Citation7–Citation9] The understanding of these properties can provide important information on the optimal conditions for their applications in food byproducts and help control product stability, shelf life, and handling.
Viscosity represents an important property in the technological aspects of honey production for it gives crucial information on the structural variation of sugar soft materials, which is heavily affected by several parameters, including temperature, water content, and presence of crystals and colloids in the product.[Citation10,Citation11] Furthermore, the crystallization of honey is a natural process, wherein honey crystallizes in fine grains and forms a gel-like structure of a dense fluid, which is determined mainly by glucose. There are also other determining factors, including other carbohydrates, moisture content, pollen, and air bubbles, which act as seed crystals. The rheological properties of honey have also been reported to depend on several other parameters such as temperature, shear rate, time, sugar composition, water activity, water content, and crystal size.[Citation7,Citation12–Citation14] Considering the increasing interests in the physicochemical, rheological, and thermal properties of honey worldwide and the limited data currently available on these aspects with respect to Tunisian honey, the present study was undertaken to determine the physicochemical, rheological, thermal, and viscoelastic properties of six Tunisian honey samples from various floral origins.
MATERIAL AND METHODS
Samples
The six honey samples used in the present study were directly obtained from local Tunisian beekeepers. They were collected at various time intervals throughout a whole year and taken from different botanical origins and regions in Tunisia. shows the different origins, types, and production period of the honey samples under investigation. The beekeepers directly extracted the samples into 250 mL sterilized glass bottles with glass caps. The samples were divided into six groups according to their floral origins and stored in a dry and dark place at 20°C until analysis.
TABLE 1 Honey samples from different regions of Tunisia
Proximate Composition Analyses
Water, ash, acidity, and protein contents were determined in accordance with the method described by the Association of Official Analytical Chemists.[Citation15] The pH was measured using a pH meter (Elico pH analyser, Elico Pvt Ltd., Mumbai) for a 10% (w/v) solution of honey prepared in milli-Q water. The electrical conductivity (EC) values of honey solutions at 20% (w/v; dry matter basis) in ultrapure water were measured at 20°C using a Consort conductometer (Consort C830, Belgium). The results were expressed as milli Siemens per centimeter (mS/cm).
Sugar composition was determined by an HPLC apparatus (Shimadzu, Tokyo, Japan) equipped with a refractive index detector (HPLC-RI) at 30°C. A honey sample (1 g) was dissolved in 19 mL milli-Q water, filtered through a 0.22 mL nylon filter into an HPLC vial, capped, and injected (20 μL) into the HPLC. The HPLC column was Supelcogel C-610H, 30 cm, 7.8 mm I.D. fitted with a guard column Supelguard C-610H 5 cm, 4.6 mm I.D. Col: H+11,855. The mobile phase was 0.005 M sulfuric acid at a flow rate of 0.75 mL/min. The external calibration curves produced by standard solutions were used to quantify the amount of sugars in the samples.[Citation16] Honey sugars were identified and quantified by comparing their retention times and peak areas with those of standard sugars. The results were expressed as gram sugar per 100 g of honey.
Rheological Measurements
The rheological properties of Tunisian honey samples were determined using a strain/stress controlled rheometer (Thermo-Haake, Rheostress 1, Germany) equipped with a temperature-control unit (Thermo-Haake, Karlsruhe K15 Germany). The rheometer had a cone-plate configuration with a cone radius of 35 mm and a gap of 0.14 mm between the cone and the plate. Measurements were carried out in the shear rate range of 0.01 to 500 s–1 at constant temperature (20°C). Briefly, 0.85 mL of sample was placed between the cone and the plate, and measurement was started immediately. In total, 25 data points were recorded at 10 s intervals during the shearing. Each measurement was replicated seven times on the same sample with two repetitions. Shear stress was determined as a function of shear rate. Data were fitted to Herschel-Bulkley and Power law models, respectively:
where refers to shear stress (Pa), K to consistency coefficient (Pa.sn),
to shear rate (s–1),
to yield stress, and n to flow behavior index (dimensionless). The time dependence of shear stress at constant shear rate of 10 s–1 and temperature of 20°C was also determined using the Weltmann equation:
where, σ refers to shear stress (Pa), t to time (s), A (value of stress at t = 1 s), and B to constants. A plot of σ versus log time should result in a straight line. While B takes negative values in thixotropic behavior, it takes positive values in antithixotropic behavior.
Amplitude sweep tests were performed at shear stress 0.00001-1 Pa, strain 0.1–20, and a fixed frequency of 1 Hz to determine the linear region of viscoelasticity. Frequency sweep tests were carried out at angular frequency 0.1–100 rad/s and constant shear stress 0.001 Pa to evaluate dynamic rheological properties, including the elastic and viscous modulus G’ and G”.
Honey is viscous in nature and the change of G” with frequency would, therefore, provide more information as compared to G’. The dependence of the G” of honey samples on angular frequency (ω) was modeled by a power type relationship:
where, G’ and G” refer to storage and loss modulus, respectively, ω to oscillation frequency, and k1, k2, a, and b to constants. The exponents a and b are the slope in a log-log plot of G’ and G” versus ω.
Thermal Analysis
The thermal properties of the honey samples were investigated by differential scanning calorimetry using a Perkin-Elmer DSC-7 (Perkin-Elmer Corp., Norwalk, CT). An aluminum volatile pan is a sample pan used with volatile solid or liquid samples which exert significant vapor pressure at the temperature of interest. Pans can withstand the internal pressure of two atmospheres (30 psi). The aluminum volatile pans were purchased from Perkin-Elmer (Norwalk, CT). Sample weights ranged from 5 to 7 mg and were submitted to the following temperature program: Honey samples were placed in aluminum volatile pans and heated at 250°C until complete melting. They were cooled to –65°C at a rate of 5°C/min and then re-heated at temperatures ranging from –65 to 250°C at a rate of 5°C/min.
Statistical Analysis of Data
Data analyses were performed in triplicate. The one-way analysis of variance was used to analyze the significance of difference between data, and the Duncan’s multiple range test was applied to analyze the significance of difference between mean values.
RESULTS AND DISCUSSION
Physicochemical Properties
The physicochemical properties as well as sugar composition are represented in . Water content is commonly used as a criterion to establish honey quality; high values can produce honey fermentation during storage, resulting in the formation of ethyl alcohol and carbon dioxide. The water content (%) of the honey samples under investigation ranged from 17.16 to 20.13. Except for mint, all the other tested Tunisian honey samples were noted to display moisture contents below 20%, which is the maximum prescribed limit for the moisture content as per the Codex standard for honey.[Citation17] The water content of the tested samples was consistent with the values previously reported for some Tunisian honeys collected in 2011, which ranged from 17.27 to 19.80%.[Citation2]
TABLE 2 Physicochemical properties and major sugar contents of Tunisian honeys
The percentage of ash content is an indicator of mineral content. It is considered as a quality criterion that indicates the possible botanical origin of honey. The ash content values recorded for the title honey samples ranged from 0.06 to 0.58% (). Generally, the ash content of blossom honey is ≤0.6% as compared to honeydew honey or blends of honeydew where this value is ≥1.2%.[Citation18] Thus, and based on this criterion, the analyzed Tunisian honey samples could be considered as being similar to blossom honey. In fact, the variability in the ash content of honey samples has previously been attributed to harvesting processes, beekeeping techniques, and the material used for honeybee collection during the foraging on the flora.[Citation6]
The pH is a useful indicator for possible microbial contamination.[Citation19] It is also considered as an important factor during the extraction and storage of honey because it is related to product stability and shelf life.[Citation20] Most bacteria and molds have previously been reported to grow in neutral and mildly alkaline environments, respectively.[Citation21] As illustrated in , all the honey samples showed an acidic character. Their pH values ranged from 3.60 to 4.04.
Furthermore, EC is one of the criteria related to the botanical origin of honey in most European countries (Greece, Spain, Italy, Switzerland, etc.) and is, therefore, commonly used in routine honey quality control. The EC values recorded for the Tunisian honey samples ranged from 0.38 to 0.75 mS cm-1. The lowest EC value was recorded for the horehound honey sample, as compared to the eucalyptus, thyme, rosemary, orange, and mint honey samples. The EC values observed for all samples did not exceeded the maximum value allowed by the Codex Alimentarius of 0.8 mS/cm.[Citation17] The values recorded for free acidity ranged between 8.15 and 27.77 meq/kg. According to the EU (Council Directive, 2001), the upper limit allowed for free acidity is 50.00 meq/kg.[Citation22]
Hydroxymethylfurfural (HMF) is an important parameter widely used for the evaluation of honey freshness and/or overheating. In the present study, the HMF values registered for the six Tunisian honey samples ranged between 12.59 and 27.99 mg/kg. The highest HMF value was observed for eucalyptus, followed by thyme, orange, horehound, and rosemary honeys. There are no significant differences (p > 0.05) between mint and rosemary. Overall, the honey samples under investigation showed HMF contents that did not exceed 60.0 mg/ kg, and considering that HMF content is indicative of honey freshness, this result suggests that the organic honey samples are fresher.[Citation23]
The percentage of proteins in the samples ranged between 0.14 and 0.16%. The water activity for the honey samples ranged between 0.56 and 0.64, which is an important criterion for determining the stability of certain processed foods through the prevention or limitation of microbial growth. The sugar compositions of the title honey samples are summarized in . The major carbohydrates of honey are fructose and glucose. The results obtained in this study revealed that fructose and glucose were present in all the examined samples at percentages ranging from 36.24 to 38.85 % and from 31.06 to 36.97 %, respectively. Eucalyptus honeys showed the highest fructose concentration which was significantly higher than the values recorded for the orange, mint, rosemary, thyme, and horehound honey samples. The rosemary and horehound honey samples were noted to display the highest mean values (more than 35%) of glucose content, compared to the other samples (p < 0.05). Sucrose and maltose were also present in Tunisian honey, though in low amounts.
Flow Behavior
The results presented in show that all the honey samples under investigation (eucalyptus, orange, thyme, mint, rosemary, and horehound) exhibited non-Newtonian behavior at shear rates ranging between 0.01 and 500 s–1 at 20°C (). A non-linear relationship was observed between shear stress (σ) and shear rate (. These results are in good agreement with the findings previously reported by Witczak et al., Smanalieva, Senge, Conforti et al., and Chen et al.[Citation8,Citation9,Citation13,Citation14] Moreover, the plot of the shear stress against shear rate of the honey samples under investigation yielded into a flow index n of less than 1 (thinning fluid), indicating that their flow behavior had a non-Newtonian profile. Similar results were, in fact, previously observed in the literature.[Citation7,Citation13,Citation14] Several studies reported that honeys are non-Newtonian liquids that have high viscosity due to their long chain structure. However, viscosity values varied distinctly depending on the type of honey samples.
FIGURE 1 Shear stress versus shear rate of Tunisian honey samples (eucalyptus, orange, thyme, mint, rosemary, and horehound) at 25°C.
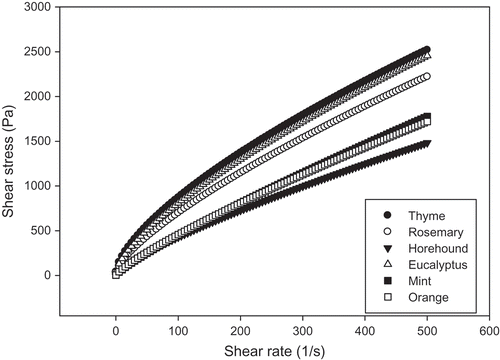
The power law and the Herschel-Bulkley models were used to describe the flow curves of the investigated honey samples. Based on the determination coefficient R2 values, the Herschel-Bulkley model was noted to be a better-fit model for flow curves (R2 > 0.99). The rheological parameters of this model are presented . The Hershel-Bulkley model was noted to be suitable for the description of Tunisian honey rheology. The results illustrated in show that thyme honey had a high value of yield stress (41.18 Pa), followed by eucalyptus (38.27 Pa), rosemary (33.27 Pa), and horehound (3.72 Pa). No significant differences were observed between the orange and mint honey samples in terms of yield stress (p > 0.05). In fact, yield stress is a parameter linked to material behavior change from elasticity to viscosity. It reflected the minimal value of shear stress that caused a distinct flow of material within the time of the experiment. This parameter plays an important role in practice. The equation described in Samanalieva and Senge[Citation14] well describes the low-temperature flow behavior and flow properties of honey samples having a tendency to crystallize. They also reported that the presence of yield stress results from sugar crystals in honey. From the theoretical perspective, yield stress does not exist since, below yield stress, the elastic behavior of a material is always accompanied by some flow.[Citation24,Citation25] Nevertheless, this flow is very small compared to material elasticity and can, therefore, be omitted.[Citation26] The yield stress value estimated from a flow curve depends, however, on the estimation equation. In this case, a yield stress value should be interpreted as a theoretical model value rather than an actual yield value stress value that represents a physical property of the fluid itself. The consistency coefficient values recorded for the Tunisian honey samples varied from 8.47 to 36.23 Pa.sn, and no significant differences were observed between the orange, mint, and horehound honey samples (p > 0.05). The highest yield stress and consistency coefficient values were observed for thyme honey samples containing the lowest content of water, and the lowest values were recorded for mint honey with the highest water content. A correlation was observed between consistency coefficients and fructose (r = 0.352, p < 0.05). In addition, there a positive correlation between consistency and HMF (r = 0.367, p < 0.05). The high viscosity rates of honey with low water content results from the anti-plasticizing effect of sugars in contrast to water. Non-Newtonian flow behavior with yield stress, thixotropy, and obvious presence of crystals were previously observed for honey samples.[Citation8] Another reason for the non-Newtonian thixotropic flow behavior of honey could be attributed to the presence of high-molecular-weight components, such as proteins or dextran. The flow behavior index (n) values displayed by all the Tunisian honey samples were lower than 1, ranging from 0.68 to 0.86, thus confirming that they exhibited non-Newtonian shear thinning flow behavior. A negative correlation between flow behavior index (n) and fructose content (r = –0.389, p < 0.05). The most pseudoplastic properties were exhibited by thyme honey samples.
TABLE 3 Parameters of Herschel-Bulkley model of Tunisian honey samples (eucalyptus, orange, thyme, mint, rosemary, and horehound) at 20°C
Temperature Dependence
The findings revealed that the honey samples under investigation underwent an exponential decrease in viscosity (µ) with the increase of temperature. Thyme honey had higher viscosity values in the temperature range of 20 to 50°C (). The results also showed that heating did not affect the non-Newtonian behavior (data not shown). The decrease in honey viscosity could presumably be attributed to the high thermal movement among molecules, which induced a reduction in the intermolecular forces, thus making the flow easier. This suggests that the increase of the temperature of honey progressively disentangles the arrangements of long chain molecules and helps overcome the intermolecular resistance to flow. This phenomenon is consistent with previous reports in the literature.[Citation27–Citation29] The effect of temperature on honey viscosity (µ) at a constant shear rate (10 s–1) can be calculated using the Arrhenius equation:
FIGURE 2 Effect of temperature on the apparent viscosities of Tunisian honey samples (eucalyptus, orange, thyme, mint, rosemary, and horehound) at shear rate of 10 s–1.
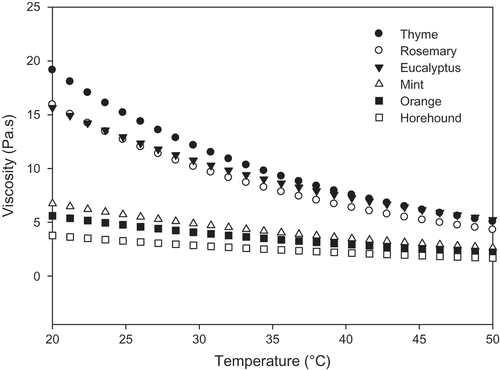
where, µ refers to apparent viscosity at a specific shear rate (10 s–1), µ∞ to the frequency factor, Ea to the activation energy (kJ/mol), R to the gas constant (8.3145 J/mol K), and T to the absolute temperature (K). By rearranging Eq. (6), a (ln µ) versus (1/T) graph can be generated, and the value of Ea can be estimated from the slope of the fitting line.[Citation30] The activation energy (Ea) can be used to measure the sensitivity of apparent viscosity changes to temperature. The activation energy and frequency factor values of the Tunisian honey samples are shown in . The highest activation energy value was observed with the thyme sample (34.91 kJ/mol), and the lowest one was recorded for the horehound sample (21.23 kJ/mol). These values were similar to the ones previously reported for Australian honey samples (22.60 kJ/mol) at a shear rate of 50 s–1.[Citation27] Israeli honey was previously described to have activation energy values ranging from 84.70 to 96.90 kJ/mol.[Citation29]
TABLE 4 Parameters of Arrhenius and Weltman models of Tunisian honey samples (eucalyptus, orange, thyme, mint, rosemary, and horehound)
No significant difference was observed between the activation energy values of the mint and orange honey samples (p > 0.05). The low activation energy of flow was reported to indicate few inter- and intra-interactions between sugar chains.[Citation31] The activation energy value calculated for the shear flow of the eucalyptus honey sample was 28.75 kJ/mol. As a general rule, the higher the activation energy is, the more temperature-dependent the flow becomes. Low activation energy values were previously taken to indicate that honey viscosity properties are less temperature-dependent.[Citation8,Citation32] The susceptibility of heather honey to changes in temperature could also be ascribed to other factors, such as content and hydration degree of protein substances responsible for the formation of a pseudo-gel structure of honey. Cohen and Weihs[Citation29] reported that activation energy (Ea) was composition-dependent, decreasing linearly with increasing moisture content. The content of individual mono-, di- and trisaccharides could also be another equally important factor. Frequency factors (µ∞) increased significantly (p < 0.05) from 1.16×10–5 to 6.20×10–4 (Pa.s) as function of honey types.
Time-dependent rheological data were adjusted by the Weltman model (Eq. [3]), which aimed to characterize the thixotropic behavior of the honey samples. The horehound honey presented lower stress values, which was required for the onset of product degradation, while the thyme and rosemary honeys contributed to an increase in initial stress (A), due to the formation of a more stable sugar network in the honey samples. The initial stress A varied significantly from 344.68 to 897.12 Pa (p < 0.05; ). The findings also revealed that parameter B, which indicated structural degradation intensity, presented no significant differences between the mint, rosemary, and orange honey samples (p > 0.05).
Viscoelastic Properties
The mechanical spectra of the Tunisian honey samples exhibited clear viscoelastic properties () The characteristic slopes of the double-logarithmic plots of the storage and loss modulus (G’ and G”) versus angular frequency were quite similar. In this case, the elastic component was also significantly different from the viscous one throughout the frequency range covered. All samples showed loss modulus G” values that were higher than those of G’, thus confirming the viscous nature of the honey samples and indicating that the values of both moduli increased with increasing angular frequency. These results were similar to the ones previously reported by Ahmed et al.[Citation7] and Oroian et al.[Citation33] Furthermore, the G’ and G” values of the Tunisian honey samples were far higher than those of Indian ones.[Citation7]
FIGURE 3 Variation of storage and loss modulus G’ and G’’ with angular frequency ω for thyme honeys. Measurements were made at 20°C and 1% strain.
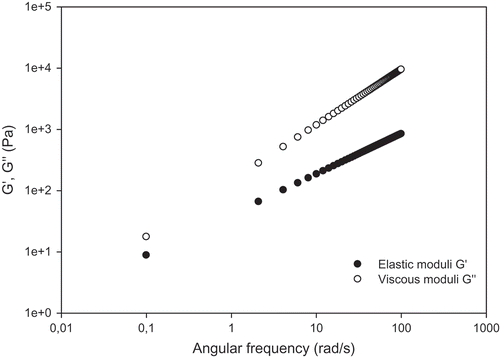
The influence of temperature on the dynamic viscoelasticity of the Tunisian honey samples was investigated at the 20–50°C range. The effect of temperature on the G’ and G” moduli of the samples is shown in . The samples showed higher loss moduli G” than storage G’ moduli throughout the whole range of temperature. There was no crossover point for G’ and G”, indicating that the honey behavior was predominantly viscous and remained in the solid-like state. During initial heating (from 20 to 50°C), G’ decreased slowly with the increase of temperature, reaching a minimum at around 50°C. The initial decrease in G’ could be attributed to the increase in fluidity with increasing temperature. shows that the power-law parameters of elastic and viscous moduli (a, b, k1, and k2) of the Tunisian honey samples underwent a decrease with the increase of temperature. The coefficient k2 was significantly higher than those of elastic moduli k1 (p < 0.05). The highest k2 value was observed for thyme, followed by eucalyptus, rosemary, mint, orange, and horehound honey samples. This decrease may also be ascribed to the energy dissipation movement of the molecules and decrease in intermolecular interactions, which, in turn, decreased the energy needed for the flow, thus decreasing the interference of the hydrodynamic domains.[Citation34,Citation35] As explained by Khondkar et al.[Citation36] the reduction of elasticity might be attributed to the thermal decomposition of some components at high temperature, thus resulting in the reduction of honey elasticity. The exponents a and b were smaller (<1), and the latter values were significantly higher than those of elastic moduli.
TABLE 5 Power-law parameters for the conservation and loss moduli of Tunisian honeys (eucalyptus, orange, thyme, mint, rosemary, and horehound) at different temperatures
Thermal Properties
The crystallization of honey usually results in the increased moisture of the liquid phase, which makes honey susceptible to yeast growth, which may lead to the fermentation of the product, production of subsequent organoleptic modifications, and degradation of quality. The differential scanning calorimeter (DSC) of cooling and heating properties of the eucalyptus, orange, thyme, mint, rosemary, and horehound honeys are summarized in . These results showed that the rosemary and horehound honey samples crystallized at lower onset temperature (–41.55 and –41.94°C, respectively) and melted at higher onset temperatures (219.06 and 221.13°C, respectively) compared to the other honey samples. Besides, the highest and lowest crystallization temperatures (Tg) were recorded for mint and rosemary honey samples, respectively. The onset of crystallization and melting process were –47.06 and 216.26°C for mint, –45.48 and 197.85°C for eucalyptus, –46.27 and 210.64°C for thyme, and –43.91 and 210.59°C for orange honey. No significant variation (p > 0.05) was observed for the glass transition temperature (Tg) of the rosemary and horehound honey samples. Generally, the honey samples with a high fructose to glucose ratio showed DSC crystallization profiles at high temperature values when compared to the honey samples with a low fructose to glucose ratio. Moreover, the fructose/glucose ratio was calculated for all the six honey samples (). This ratio reflects the crystallization state of honey, i.e., when fructose is higher than glucose, the honey is fluid.[Citation37,Citation38] In fact, honey samples that do not crystallize for a long time have previously been reported to show a Fructose/Glucose (F/G) ratio that is higher than 1.33.[Citation14] If the F/G ratio is lower than 1.11, honey crystallizes quickly. The fructose/glucose ratio has also been reported to have an impact on the honey flavor since fructose is much sweeter than glucose.[Citation3] Furthermore, the glass transition temperature (Tg) of honey was also described to depend on various factors, including water activity, sugar composition, and water content.[Citation12,Citation37,Citation38] Higher correlations were, however, observed between glass transition temperature (Tg) and fructose to glucose ratio (Pearson coefficient: r = –0.969, p < 0.01) and between water content and glass transition temperature (r = –0.722, p < 0.01).
TABLE 6 Glass transition Tg, melting point Tm temperatures of six Tunisian honeys (eucalyptus, orange, thyme, mint, rosemary, and horehound)
CONCLUSION
The findings from rheological analysis revealed that all Tunisian honey samples investigated in the present work were non-Newtonian fluids with good elastic properties. Thyme honey exhibited higher viscosity rates than the other examined honey samples. The results from the thermal property assays showed that the Tunisian honey samples crystalize at low temperatures. Thyme honey was also noted to exhibit good stability, a property that is highly valued from the industrial perspective. These results suggest that honey can be used as a functional ingredient in the manufacture of cosmetic, agrochemical, and pharmaceutical products.
ACKNOWLEDGMENT
The authors would like to express their sincere gratitude to Mr. Anouar Smaoui from the English Language Unit at the Faculty of Science of Sfax, Tunisia for his constructive proofreading and language editing services.
FUNDING
The authors would like to thank the Tunisian Ministry of Higher Education, Scientific Research, and Technology for the financial support of the present study.
Additional information
Funding
REFERENCES
- Ferreira, I.C.F.R.; Aires, E.; Barreira, J.C.M.; and Estevinho, L.M. Antioxidant Activity of Portuguese Honey Samples: Different Contributions of the Entire Honey and Phenolic Extract. Food Chemistry 2009, 114, 1438–1443.
- Boussaid, A.; Chouaibi, M.; Rezig, L.; Donsi, F.; Ferrari, G.; Hamdi, S. Physicochemical and Bioactive Properties of Six Honey Samples from Various Floral Origins from Tunisia. Arabian Journal of Chemistry [Online early access]. DOI:10.1016/j.arabjc.2014.08.011. Published Online: Aug 28, 2014.
- The National Honey Board. Honey-Health and Therapeutic Qualities. http://www.nhb.org/download /factsht/compendium 2002. (accessed December 11, 2004).
- Alvarez-Suarez, J.M.; Tulipani, S.; Díaz, D.; Estevez, Y.; Romandini, S.; Giampieri, F.; Damiani, E.; Astolfi, P.; Bompadre, S.; Battino, M. Antioxidant and Antimicrobial Capacity of Several Monofloral Cuban Honeys and Their Correlation with Color, Polyphenol Content, and Other Chemical Compounds. Food and Chemical Toxicology 2010, 48, 2490–2499.
- Shoham, S.; Youdim, M.B. Iron Involvement in Neural Damage and Microgliosis in Models of Neurodegenerative Diseases. Cellular and Molecular Biology 2000, 46(4), 743–760.
- Finola, M.S.; Lasagno, M.C.; Marioli, J.M.; Microbiological and Chemical Characterization of Honeys from Central Argentina. Food Chemistry 2007, 100, 1649–1653.
- Ahmed, J.; Prabhu, S.T.; Raghavan, G.S.V.; Ngadi, M. Physico-Chemical, Rheological, Calorimetric, and Dielectric Behavior of Selected Indian Honey. Journal of Food Engineering 2007, 79, 1207–1213.
- Chen, Y.W.; Lin, C.H.; Wu, F.Y.; Chen, H.H. Rheological Properties of Crystallized Honey Prepared by a New Type of Nuclei. Journal of Food Process Engineering 2007, 32, 512–527.
- Conforti, P.A.; Lupano, C.E.; Malacalza, N.H.; Arias, V.; Castells, C.B. Crystallization of Honey at –20°C. International Journal of Food Properties 2006, 9, 99–107.
- Bhandari, B.; D’arcy, B.; Chow, S. A Research Note: Rheology of Selected Australian Honeys. Journal of Food Engineering 1999, 41, 65–68.
- Juszczak, L.; Fortuna, T. Rheology of Selected Polish Honeys. Journal of Food Engineering 2006, 73, 43–49.
- Gleiter, R.A.; Horn, H.; Isengard, H.D. Influence of Type and State of Crystallization on the Water Activity of Honey. Food Chemistry 2006, 96, 441–445.
- Witczaka, M.; Juszczak, L.; Gałkowsk, D. Non-Newtonian Behaviour of Heather Honey. Journal of Food Engineering 2011, 104, 532–537.
- Smanalieva, J.; Senge, B. Analytical and Rheological Investigations into Selected Unifloral German Honey. European Food Research and Technology 2009, 229, 107–113.
- AOAC. Official Methods of Analysis of AOAC International, 16th Ed; Association of Official Analytical Chemists: Washington, DC, 1990.
- Bonta, V.; Mărghitas, P.; Bobis, O.; Dezmirean, D. HPLC Sugar Profile in Quality and Authenticity Determination of Romananian Honey. Apiculture-from Science to Agri-Business and Apitherapy. Cluj-Napoca Proceedings 2007, 3, 58–63.
- Codex Alimentarius Commission Standards (2001). CODEX STAN 12-1981, Rev.1, 1987, Rev.2.
- Ouchemoukh, S.; Louaileche, H.; Schweizer, P. Physicochemical Characteristics and Pollen Spectrum of Some Algerian Honey. Food Control 2007, 18, 52–58.
- Conti, M.E. Lazio Region (Central Italy) Honeys: Determination of Mineral Content and Typical Quality Parameters. Food Control 2000, 11, 459–463.
- Terrab, A.; Recamales, A.F.; Hernanz, D.; Heredia, F.J. Characterization of Spanish Thyme Honeys by Their Physicochemical Characteristics and Mineral Contents. Food Chemistry 2004, 88, 537–542.
- Conti, M.E.; Saccares, S.; Cubadda, F.; Cavallina, R.; Tenoglio, C.A.; Ciprotti, L. Honey in Lazio: Survey content in trace metals and radionuclides. Riv.Sci. Alim 1998, 2, 107–119.
- EU. Council Directive 2001/110 Relating to Honey. Official Journal of the European Communities 2001, 10, 47–52.
- Gomes, T.; Fea’s, X.; Iglesias, A.; Estevinho, L.M. Study of Organic Honey from the Northeast of Portugal. Molecules 2011, 16, 5374–5386.
- Barnes, H.A.; Walters, K. The Yield Stress Myth? Rheologica Acta 1985, 24(4), 323–326.
- Barnes, H.A. The Yield Stress-A Review or παντα ρει—Everything Flows? Journal of Non-Newtonian Fluid Mechanics 1999, 81, 133–178.
- Mujumdar, A.N.; Beris, A.N.; Metzner, A.B. Transient Phenomena in Thixotropic Systems. Journal of Non-Newtonian Fluid Mechanics 2002, 102, 157–178.
- Sopade, P.A.; Halley, P.; Bhandari, B.D.; Arcy, B.; Doebler, C.; Caffin, N. Application of the Williams-Landel-Ferry Model To The Viscosity-Temperature Relationship of Australian Honeys. Journal of Food Engineering 2002, 56, 67–75.
- Lazaridou, A.; Biliaderis, C.G.; Bacandritsos, N.; Sabatini, A.G. Composition, thermal, and Rheological Behaviour of Selected Greek Honeys. Journal of Food Engineering 2004, 64, 9–21.
- Cohen, I.; Weihs, D. Rheology and Microrheology of Natural and Reduced-Calorie Israeli Honeys as a Model for High-Viscosity Newtonian Liquids. Journal of Food Engineering 2010, 10, 366–371.
- Chouaibi, M.; Mahfoudhi, N.; Rezig, L.; Donsì, F.; Ferrari, G.; Hamdi, S. A Comparative Study on Physicochemical, Rheological, and Surface Tension Properties of Tunsisan Jujube (Zizyphus Lotus L.) Seed and Vegetable Oils. International Journal of Food Engineering 2012, 8, 1–20. DOI:10.1515/1556-3758.2759.
- Mohammadifara, M.A.; Musavia, S.M.; Kiumarsib, A.; Williamsc, P.A. Solution Properties of Targacanthin (Water Soluble Part of Gum Tragacanth Exudate from Astragalus Gossypinus). International Journal of Biological Macromolecules 2006, 38, 31–39.
- Kang, K.M.; Yoo, B. Dynamic Rheological Properties of Honeys at Low Temperatures As Affected by Moisture Content and Temperature. Food Science and Biotechnology 2008, 17(1), 90–94.
- Oroian, M.; Amariei, S.; Escriche, I.; Gutt, G. Aviscoelastic Model for Honeys Using the Time-Temperature Superposition Principle (TTSP). Food Bioprocess Technology 2013, 6, 2251–2260.
- Bohdanecky, M.; Kovar, J. Viscosity of Polymer Solutions. In Polymer Kience Library Vol. 2; Jenkis, A.D.; Ed.; Elsevier Scientific: Amsterdam-Oxford-New York, 1982.
- Lapasin, R.; Pricl, S. Rheology of Polysaccharide Systems. In Rheology of Industrial-pol. Saccharides: Theory and Applications; Lapasin, R.; Pricl, S.; Eds.;. Blackie Academic and Professional: Glasgow, 1995; 250–494.
- Khondkara, D.; Testera, R.F.; Hudsonb, N.; Karkalasa, J.; Morrow, J. Rheological Behaviour of Uncross-Linked and Cross-Linked Gelatinized Waxy Maize Starch with Pectin Gels. Food Hydrocolloids 2007, 21, 1296–1301.
- Ouchemoukh, S.; Schweitzer, P.; Bey, M.B.; Djoudad-Kadji, H.; Louaileche, H. HPLC Sugar Profiles of Algerian Honeys. Food Chemistry 2010, 121, 561–568.
- Venir, E.; Spaziani, M.; Maltini, E. Crystallization in “Tarassaco” Italian Honey Studied by DSC. Food Chemistry 2010, 122, 410–415.