Abstract
Stilbenes contained in various foods are associated with health beneficial effects. In this study six natural and one synthetic stilbene were tested for their potential to regulate the activity of lipoxygenase and cyclooxygenase in vitro. The most potent inhibitor of 5-lipoxygenase was pterostilbene (IC50 = 9.32 μM), whereas the strongest inhibitor of cyclooxygenase-1 and cyclooxygenase-2 was pinostilbene (IC50s = 1.90 and 0.35 μM, respectively). Pterostilbene (IC50s = 11.70 and 27.04 μM for cyclooxygenase-1 and cyclooxygenase-2, respectively) and oxyresveratrol (IC50s = 18.49; 2.79 and 14.71 μM for 5-lipoxygenase, cyclooxygenase-1, and cyclooxygenase-2, respectively) were capable to inhibit catalytic activity of all three tested enzymes. Isorhapontigenin (IC50s = 8.81 and 24.00 μM for cyclooxygenase-1 and cyclooxygenase-2, respectively) and rhapontigenin (IC50s = 24.55 and 36.12 μM for cyclooxygenase-1 and cyclooxygenase-2, respectively) were only moderate or weak inhibitors of both cyclooxygenase forms. In summary, these results indicated that besides the known cyclooxygenase inhibitor resveratrol also other natural stilbenes could be potent inhibitors of the arachidonic acid pathway and deserve further attention as compounds with promising health benefits.
INTRODUCTION
Natural stilbenes are polyphenolic compounds that occur in various foods of plant origin. Many of these compounds are able to alter enzymatic and chemical reactions in our body and therefore, are of great interest due to their medical potential. The most extensively studied compound within this family is doubtlessly resveratrol (3,5,4’-trihydroxy-trans-stilbene), primarily found in peanuts, grapes, and red wine.[Citation1,−Citation5] Resveratrol is often mentioned as a compound responsible for the positive effects of moderate red wine consumption and is supposed to prevent or delay the onset of cancer, heart disease, ischemic and chemically induced injuries, diabetes, pathological inflammation, oxidative stress, and viral infection.[Citation6,Citation7] The beneficial effects of resveratrol and other stilbenes, are partly attributed to their ability to inhibit cyclooxygenase (COX) and 5-lipoxygenase (5-LO).[Citation8−Citation10]
COX-1 and -2 are enzymes catalyzing the conversion of arachidonic acid (AA) to biological mediators called prostanoids. The biosynthesis and actions of prostanoids are blocked by clinically relevant non-steroidal anti-inflammatory drugs (e.g., aspirin, indomethacin, ibuprofen), which provide anti-inflammatory, anti-pyretic, and analgesic effects. The second pharmacologically relevant metabolic pathway of AA is mediated by 5-LO. This enzyme is involved in the biosynthesis of inflammatory mediators named leukotrienes (LTs) and its regulation is used clinically to control asthma.[Citation11] Both COXs and 5-LO are also putative targets for cancer prevention.[Citation12,Citation13] Alternative cancer prevention strategies using diet-based approaches and bioactive food components that target COX and/or 5-LO catalytic activity are currently getting interesting.[Citation14] In this regard, six natural and one synthetic methoxylated and hydroxylated stilbenes were evaluated for inhibition of COX-1, -2, and 5-LO activity in vitro. The active compounds were subsequently docked in silico to the target proteins to propose their mode of inhibition.
MATERIALS AND METHODS
Standards and Chemicals
Isorhapontigenin, pterostilbene, resveratrol, oxyresveratrol, pinostilben, eicosatetraynoic acid (ETYA), calciumionophor A23187, dimethylsulfoxide (DMSO), AA, indomethacin, trypan blue, gentian violet, porcine hematin, L-epinephrine, formic acid, human recombinant COX-2, and COX-1 from ram seminal vesicles were purchased from Sigma-Aldrich (Czech Republic). Dextran T-500 was purchased from Roth (Germany). Zileuton was donated by Farmak a.s. (Czech Republic). Rhapontigenin and 2,3’,4,5’-tetramethoxystilbene (TMS) were purchased from Cayman Chemical (USA). Tris was purchased from Bio-Rad (Czech Republic).
COX-1 and COX-2 Assay
The assay was performed as previously described.[Citation15] COX-1 (1 unit/reaction) from ram seminal vesicles or human recombinant COX-2 (0.5 unit/reaction) was added to 180 μL of incubation mixture consisting of 100 mM tris buffer (pH 8), 5 μM porcine hematin, 18 mM L-epinephrine, and 50 μM Na2EDTA. The test substance dissolved in DMSO or pure DMSO (in case of blank) was added (10 μL) and the mixture was pre-incubated for 5 min at room temperature. The addition of 5 μL of 10 μM AA started the reaction. After 20 min incubation at 37°C, the reaction was stopped by 10 μL of 10% formic acid. All samples were diluted 1:15 in assay buffer and the concentration of PGE2 produced by the reaction was determined by a PGE2 ELISA kit (Enzo Life Sciences, USA) according to the manufacturer’s instructions. Absorbance relative to PGE2 concentration was measured by microplate reader Tecan Infinite M200 (Tecan Group, Switzerland) at 405 nm. The results were expressed as percentage inhibition of PGE2 formation against untreated samples (blanks). The test was performed in three independent experiments with two replicates.
5-LO Assay
The assay performed was a slightly modified version of method described previously.[Citation16] Buffy coat (50 mL) obtained from healthy donors was sedimented in 20 mL of dextrane solution (6% dextran T-500, 1% NaCl) at 4°C. After one hour the supernatant was collected and centrifuged at 1600 rpm at 4°C for 10 min, then the supernatant was discarded. The obtained pellet was washed by phosphate buffered saline (PBS, 0.02% KCl, 0.024% KH2PO4, 0.8% NaCl, 0.288% Na2HPO4 •12H2O, pH 7.4) and again centrifuged. The hereby obtained pellet was lysed (0.17% NH4Cl, 0.2% Tris, pH 7.2) for 5 min at room temperature and then centrifuged at 1400 rpm at 4°C for 5 min. The pellet was washed by PBS again and centrifuged at 1400 rpm at 4°C for 15 min. Finally, the pellet was dissolved in 3 mL of PBS and the cells were tested for the viability. The cells were then diluted to the final concentration of 4500 cell/μL for the test.
The incubation mixture consisted of 225 μL of the cell suspension, 10 μL of 2 mM CaCl2, 10 μL of 10 μM ETYA, 5 μL of tested sample or pure DMSO (in case of blank), 10 μL of 21 μM calcium ionophor A23187, and 5 μL of 120 μM AA. The reaction was stopped after 10 min incubation at 37°C by 20 μL of 10% formic acid. Samples were diluted 40 times in ELISA buffer and the concentration of LTB4 was measured using a commercial LTB4 ELISA kit (Enzo Life Sciences, USA) according to the manufacturer’s instructions. Absorbance relative to LTB4 concentration was measured at 405 nm using a Tecan Infinite M200. The results were expressed as percentage inhibition of LTB4 formation against untreated samples (blanks). The test was performed in three independent experiments with two replicates. At least three concentrations were used for the calculation of IC50 values tested compounds.
Docking
The docking simulation was performed with the software package GOLD 5.1 (GOLD, UK) using the x-ray crystal structure of 5-LO (3o8y[Citation17]) and COX-2 (6cox[Citation18]) from the Protein Data Bank (PDB[Citation19]). The structure of 5-LO does not contain a co-crystallized ligand, so the binding site was defined in a 4 Å radius around the catalytic iron (Fe2_1_A). Water molecules inside the binding pocket were set on toggle and spin, which means that the program can either use them as binding partners in the binding site or disregard them if they provoke a steric hindrance for ligand binding. In the case of COX-2 docking, the binding site was defined as a sphere of 6 Å radius centered at the location of the co-crystallized ligand. Subsequently, 3D protein-ligand interaction patterns of both enzymes were generated in LigandScout 3.1.[Citation20] using default settings.
RESULTS AND DISCUSSION
Impact of Stilbenes on 5-LO Enzymatic Activity
Seven methoxylated or/and hydroxylated stilbenes were tested for their potential to inhibit 5-LO enzymatic activity. This selection included two hydroxylated structures (resveratrol and oxyresveratrol), three monomethoxylated (pinostilbene, rhapontigenin, and isorhapontigenin), one dimethoxylated (pterostilbene), and one tetramethoxylated structure (TMS, the synthetic stilbene; for the structures see ). As shown in , the most potent inhibitor of 5-LO was pterostilbene (IC50 = 9.32 μM), moderate activity was observed for oxyresveratrol (IC50 = 18.49 μM) and only weak or no activity for pinostilbene, resveratrol, rhapontigenin, isorhapontigenin, and TMS (IC50 > 50 μM). To further elucidate the mode of inhibition, pterostilbene was docked into the crystal structure of 5-LO (3o8y). This compound formed hydrogen bonds with Thr364 and His372 which are two of the amino acids that define the binding cavity of 5-LO. A comparison of pterostilbene with the inactive methoxylated compounds (isorhapontigenin, rhapontigenin, TMS, and pinostilbene), indicates that two methoxy groups are most favorable for the inhibition of 5-LO. In the case of oxyresveratrol, the additional hydroxyl group on the stilbene ring increases anti-oxidant activity.[Citation21] Therefore, it is possible that oxyrasveratrol inhibits 5-LO via same mechanism as some structurally related hydroquinones.[Citation22] The low activity recorded for resveratrol is in concordance with three recent studies,[Citation23−Citation25] where the authors concluded, that resveratrol itself is not a potent inhibitor of 5-LO activity while oxidation products of resveratrol (namely it’s oligomers and dimers) are responsible for inhibition of 5-LO. Resveratrol itself interacts with Gln557 and Tyr181 but cannot form the interaction with the iron or the His367 which could explain its low activity.
TABLE 1 IC50 values of stilbenes and reference inhibitors on COX-1, COX-2, and 5-LO
Impact of Stilbenes on COX-1 and COX-2 Enzymatic Activity
The second part of our study was focused on the potential of natural stilbenes to inhibit COX-1 and COX-2 in vitro. IC50 values and COX-1/COX-2 ratios of tested compounds are shown in . The most active inhibitors of COX-1 were pinostilbene, resveratrol, and oxyresveratrol. Moderate activity was observed with isorhapontigenin, pterostilbene, and rhapontigenin. TMS was not active at 50 μM concentration. In the case of COX-2, the activity of the compounds decreased in the same order. Interestingly, pinostilbene was the only tested compound preferentially inhibiting COX-2. In silico docking suggested that pinostilbene forms hydrogen bonds with Arg120, Phe518, and Gln192 (). Gln192 is one of the amino acids that lines the pocket of secondary COX-2 binding site and the interaction with this amino acid is characteristic for COX-2 selective inhibitors.[Citation26]
FIGURE 2 Docking pose of pinostilbene in COX-2. Spheres signify hydrophobic interactions with the binding pocket. The arrows pointing from protein structure to pinostilbene signify a hydrogen bond acceptor. The arrows pointing from pinostilbene to protein structure signify hydrogen bond donor interaction.
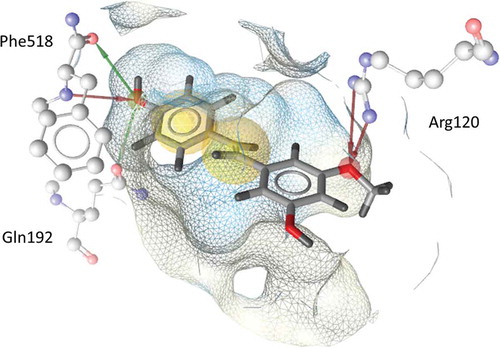
Poor activity of methoxylated derivative of oxyresveratrol (TMS) indicates that hydroxyl groups are for COX inhibition more favorable than methoxy groups. This hypothesis also fits to the inhibitory activity of pinostilbene and pterostilbene. Their structures differ only in the presence of a hydroxyl (pinostilbene which is more active) or methoxy (pterostilbene which is less active) group at position 3 on the ring. On the other hand, resveratrol with hydroxyl groups on position 3 and 5 was less active than pinostilbene. Murias et al.[Citation27] revealed that hydroxylated stilbenes are better COX-2 inhibitors than their methoxylated analogs and that hydroxylated stilbenes (with four or more hydroxyl groups) are more or less significant selective COX-2 inhibitors. However, oxyresveratrol (not included in mentioned study) preferably inhibited COX-1 in our study. It seems there is no evident strict rule for the structure-activity relationship for the stilbenes investigated in our study.
CONCLUSION
This study revealed that not only resveratrol but also other dietary stilbenes could have health benefits via regulation of the AA pathway. Particularly, pinostilbene was a strong inhibitor of COX-2 while pinostilbene and oxyresveratrol were potent inhibitors of COX-1. Pterostilbene was an effective inhibitor of 5-LO and together with oxyresveratrol were the compounds capable to inhibit catalytic activity of all three tested enzymes.
FUNDING
This work was financially supported by the Czech Ministry of Education, Youth and Sports project LH12164 (Czech–Chinese cooperation), the Österreichischer Austausch Dienst (OeAD) Project CZ14/2013, and the Austrian Science Fund NFN project S10711.
REFERENCES
- Liu, C.D.; Wen, Y.Y.; Chiou, J.M.; Wang, K.H.; Chiou, R.Y.Y. Comparative characterization of peanuts grown by aquatic floating cultivation and field cultivation for seed and resveratrol production. Journal of Agricultural and Food Chemistry 2003, 51, 1582–1585.
- Jeandet, P.; Bessis, R.; Sbaghi, M.; Meunier, P. Production of the phytoalexin resveratrol by grapes as a response to botrytis attack under natural conditions. Journal of Phytopathology-Phytopathologische Zeitschrift 1995, 143, 135–139.
- Cantos, E.; Espin, J.C.; Tomas-Barberan, F.A. Postharvest stilbene-enrichment of red and white table grape varieties using UV-C irradiation pulses. Journal of Agricultural and Food Chemistry 2002, 50, 6322–6329.
- Ghatak, A.A.; Chaturvedi, P.A.; Desai, N.S. Indian grape wines: A potential source of phenols, polyphenols, and antioxidants. International Journal of Food Properties 2014, 17, 818–828.
- Raczkowska, J.; Mielcarz, G.; Howard, A.; Raczkowski, M. UPLC and spectrophotometric analysis of polyphenols in wines available in the polish market. International Journal of Food Properties 2011, 14, 514–522.
- Baur, J.A.; Sinclair, D.A. Therapeutic potential of resveratrol: The in vivo evidence. Nature Reviews Drug Discovery 2006, 5, 493–506.
- Pandey, K.B.; Rizvi, S.I. Ferric reducing and radical scavenging activities of selected important polyphenols present in foods. International Journal of Food Properties 2012, 15, 702–708.
- Kimura, Y.; Okuda, H.; Arichi, S. Effects of stilbenes on arachidonate metabolism in leukocytes. Biochimica et Biophysica Acta 1985, 834, 275–278.
- Zykova, T.A.; Zhu, F.; Zhai, X.; Ma, W.-Y.; Ermakova, S.P.; Lee, K.W.; . . . Dong, Z. Resveratrol directly targets COX-2 to inhibit carcinogenesis. Molecular Carcinogenesis 2008, 47, 797–805.
- Kimura, Y.; Okuda, H.; Kubo, M. Effects of stilbenes isolated from medicinal plants on arachidonate metabolism and degranulation in human polymorphonuclear leukocytes. Journal of Ethnopharmacology 1995, 45, 131–139.
- Funk, C.D. Prostaglandins and leukotrienes: Advances in eicosanoid biology. Science 2001, 294, 1871–1875.
- Harris, R.E.; Beebe-Donk, J.; Alshafie, G.A. Cancer chemoprevention by cyclooxygenase 2 (COX-2) blockade: Results of case control studies. Sub-Cellular Biochemistry 2007, 42, 193–212.
- Menna, C.; Olivieri, F.; Catalano, A.; Procopio, A. Lipoxygenase inhibitors for cancer prevention: Promises and risks. Current Pharmaceutical Design 2010, 16, 725–733.
- Romagnolo, D.F.; Papoutsis, A.J.; Selmin, O. Nutritional targeting of cyclooxygenase-2 for colon cancer prevention. Inflammation & Allergy Drug Targets 2010, 9, 181–191.
- Reininger, E.A.; Bauer, R. Prostaglandin-H-synthase (PGHS)-1 and-2 microtiter assays for the testing of herbal drugs and in vitro inhibition of PGHS-isoenzyms by polyunsaturated fatty acids from Platycodi radix. Phytomedicine 2006, 13, 164–169.
- Adams, M.; Kunert, O.; Haslinger, E.; Bauer, R. Inhibition of leukotriene biosynthesis by quinolone alkaloids from the fruits of Evodia rutaecarpa. Planta Medica 2004, 70, 904–908.
- Gilbert, N.C.; Bartlett, S.G.; Waight, M.T.; Neau, D.B.; Boeglin, W.E.; Brash, A.R.; Newcomer, M.E. The structure of human 5-Lipoxygenase. Science 2011, 331, 217–219.
- Kurumbail, R.G.; Stevens, A.M.; Gierse, J.K.; McDonald, J.J.; Stegeman, R.A.; Pak, J.Y.; . . . Stallings, W.C. Structural basis for selective inhibition of cyclooxygenase-2 by anti-inflammatory agents. Nature 1996, 384, 644–648.
- Berman, H.M.; Westbrook, J.; Feng, Z.; Gilliland, G.; Bhat, T.N.; Weissig, H.; . . . Bourne, P.E. The protein data bank. Nucleic Acids Research 2000, 28, 235–242.
- Wolber, G.; Langer, T. LigandScout: 3-d pharmacophores derived from protein-bound ligands and their use as virtual screening filters. Journal of Chemical Information and Modeling 2005, 45, 160–169.
- Rossi, M.; Caruso, F.; Opazo, C.; Salciccioli, J. Crystal and molecular structure of piceatannol; scavenging features of resveratrol and piceatannol on hydroxyl and peroxyl radicals and docking with transthyretin. Journal of Agricultural and Food Chemistry 2008, 56, 10557–10566.
- Landa, P.; Kutil, Z.; Temml, V.; Malik, J.; Kokoska, L.; Widowitz, U.; . . . Vanek, T. Inhibition of in vitro leukotriene B4 Biosynthesis in human neutrophil granulocytes and docking studies of natural quinones. Natural Product Communications 2013, 8, 105–108.
- Pinto, M.C.; Garcia-Barrado, J.A.; Macias, P. Resveratrol is a potent inhibitor of the dioxygenase activity of lipoxygenase. Journal of Agricultural and Food Chemistry 1999, 47, 4842–4846.
- Shingai, Y.; Fujimoto, A.; Nakamura, M.; Masuda, T. Structure and function of the oxidation products of polyphenols and identification of potent lipoxygenase inhibitors from Fe-catalyzed oxidation of resveratrol. Journal of Agricultural and Food Chemistry 2011, 59, 8180–8186.
- Adams, M.; Pacher, T.; Greger, H.; Bauer, R. Inhibition of leukotriene biosynthesis by stilbenoids from Stemona species. Journal of Natural Products 2005, 68, 83–85.
- Michaux, C.; Charlier, C. Structural approach for COX-2 inhibition. Mini-Reviews in Medicinal Chemistry 2004, 4, 603–615.
- Murias, M.; Handler, N.; Erker, T.; Pleban, K.; Ecker, G.; Saiko, P.; . . . Jäger, W. Resveratrol analogues as selective cyclooxygenase-2 inhibitors: Synthesis and structure-activity relationship. Bioorganic & Medicinal Chemistry 2004, 12, 5571–5578.