Abstract
This study examined some physicochemical properties of actomyosin isolated from pre-rigor chicken breast muscle (Pectoralis major) to gain insight on its interaction with 0.2–1.0M sodium bicarbonate (NaHCO3) either with or without 3% sodium chloride (NaCl). NaHCO3 has been a frequent quality raiser with respect to water holding capacity of meat, which is adversely affected at low pH. This study document a remarkable inactivation of ATPases and the weakening of actin-myosin interaction with increasing molarity of NaHCO3, irrespective of the presence or absence of NaCl. Similarly, there was a concomitant increase in turbidity of soluble fraction, increase in total protein solubility and reactive SH groups. The observations on protein solubility/insolubility are supported by SDS-PAGE profiles. Therefore, the conformational changes induced by NaHCO3 are concentration dependent and appear different from those during thermal transitions. The findings suggest that low concentration of NaHCO3 may be more suitable component of marinades, since it affects the main myofibrillar contractile complex less adversely.
INTRODUCTION
Two of the major concerns of meat producers are consumer acceptability and good yield, which are influenced by a number of post-mortem factors including pH fall, temperature and duration of storage, tenderness or convertibility to processed products. PSE or “pale, soft, and exudative” condition faced by poultry as well as cattle meat is a pH-fall related acute problem.[Citation1,] The time lag taken by chilling to reach the interior of muscle results in pH fall that denatures proteins, inflicting drip losses and texture changes.[Citation2,Citation3] To improve water holding capacity and modify textural properties of poultry meat, various phosphates and sodium chloride (NaCl) solutions have been frequently used as marinades.[Citation4−Citation6] Inclusion of sodium bicarbonate in marinades of various other salts has the advantage of shifting pH to alkalinity and thus, helping overcome PSE problem and, in turn, lack of juiciness.[Citation7,Citation8] Therefore, sodium bicarbonate has become the salt of preferential use in meat industry during recent years, because at even low concentrations it restores meat pH to desirable levels.[Citation5,Citation6,Citation9] According to some recent reports sodium bicarbonate has a number of other advantages also; for instance, it reduces shear force and improves water holding capacity, textural properties, and yield of the poultry meat.[Citation9−Citation11] NaCl, the other salt used in the present study, has not only been reported to increase yield but enhance the effect of phosphates and carbonates which improve meat quality.[Citation12,Citation13]
Because of applied value, meat or myofibrillar products have been the objects of more intense investigations for water holding capacities, color change, juiciness, and textural properties.[Citation14] However, an intimate correlation can be conceived between functionality of isolated actomyosin and characteristics of muscle as food due to quantitative dominance of myosin and actin, which constitute ˜43 and 22% of the protein contents of muscle mass.[Citation15] It is, therefore, not surprising that most of the fundamental information on functional properties of meat originated from in vitro studies on myosin, its complex with actin (actomyosin) and regulatory and various substructural proteins. Due to decisive roles in defining post-mortem behavior of meat, these proteins have been central in understanding the events during thermal exposure and gelation, frozen storage or processing.[Citation16−Citation19]
Although the basic response of actomyosin complex has been critical to understanding the functionality of muscle as food, a search of literature revealed no published information on in vitro behavior of actomyosin to widely used phosphates or bicarbonates in marination. The present investigations were, therefore, aimed at investigating some basic aspects of the effect of sodium bicarbonate and NaCl on isolated actomyosin of broiler breast muscles (Pectoralis major) and to review them with the published evidence on related aspects of myofibrillar proteins of poultry meat.
MATERIALS AND METHODS
Chemicals
Acrylamide, Bis-acrylamide, Phenyl methane sulfonylfluoride (PMSF), Adenosine 5′-Triphosphate disodium salt (ATP), Ammonium per sulphate and TEMED were procured from Sigma-Aldrich chemicals Pvt. Ltd. 1-Amino-2-naphthol-4-sulphonic acid, 5-5′-dithiobis (2-nitrobenzoic acid; DTNB), Potassium chloride, Bovine serum albumin, and Tris Buffer were purchased from SRL, India. All other chemicals and reagents used were of analytical grade.
Preparation of Actomyosin
Actomyosin was extracted according to Ahmad and Hasnain[Citation20] from breast muscle (Pectoralis major) of freshly sacrificed broiler chickens (n = 4), with slight modifications as per the requirement of present experiment. Instantly after excision, breast muscles (10 gm) were blended under cooling by immersing the probe of homogenizer (Make: Biospec) and the pH value of muscle (= 5.9 ± 0.1) was recorded by immersing electrode of digital pH meter (Bio-Matrix India) in the above aqueous homogenate. The pH meter was calibrated for the pH range of 4.0 to 9.1.
For each actomyosin preparation, breast muscle mince was washed three times with five volumes of sodium phosphate buffer (50 mM, pH 7.2) containing 2 mM phenyl methane sulfonylfluoride (PMSF). Addition of protease inhibitor, PMSF, was used to inactivate the proteases present in the solution. Extraction of actomyosin from washed muscle mince was carried out overnight in 0.6M KCl buffered with 20 mM Tris-maleate (pH 7.2). The viscous extract was filtered through gauze to separate out connective tissue. Actomyosin from filtrate was precipitated in ten volumes of chilled distilled water (0–2°C) and collected after centrifugation at 5000 rpm for 20 min at 4°C, using REMI, C-24 BL centrifuge. The actomyosin, dissolved in 0.6M NaCl (20 mM Tris-maleate, pH 7.2), was kept undisturbed for 30 min at 4°C. Dissolved actomyosin was washed twice with 10 volumes of chilled distilled water before finally dissolving in 0.6M NaCl (20 mM Tris-maleate, pH 7.2). After overnight dialysis with Tris-maleate buffer (20 mM, pH 7.2) containing 0.6M NaCl, the actomyosin was ready to use.
Protein Estimation
Protein content in actomyosin samples was determined by Lowry’s method[Citation21] using bovine serum albumin as the standard. Absorbance (optical density) was recorded at 660 nm on GENESYS 10 UV scanning spectrophotometer.
Treatment with Sodium Bicarbonate
Prior to suspension in sodium bicarbonate, actomyosin dissolved in 0.6M NaCl (Tris-maleate 20mM, pH 7.2) was precipitated with ten volumes of chilled distilled water. After centrifugation at 4000 rpm for 15 min at 4°C, actomyosin pellet was saved and suspended in chilled sodium bicarbonate (NaHCO3) solution (pH 8.4) of different molarity (0.2M, 0.4M, 0.6M, 0.8M, and 1M) along with or without NaCl (3% v/v). Subsequently, after proper mixing, final concentration of all actomyosin solutions was adjusted to 3.5 mg/mL. Before further analysis, the mixtures were kept undisturbed for 30 min at 4°C to allow samples to reach equilibrium.
Measurement of ATPase Activity
Ca2+-ATPase and Mg2+-ATPase activities were determined following the method of Hasnain et al.[Citation22] with slight modifications. Briefly, 2 mL assay mixture at final concentrations contained: 25 mM Tris-HCl buffer (pH 7.5), 5 mM CaCl2 (for Ca2+-ATPase assay)/or 5 mM MgCl2 (for Mg2+-ATPase assay), 1 mM ATP and 0.35 mg/mL proteins. The ionic strength of KCl solution for Mg2+-ATPase and Ca2+-ATPase was 20 and 30 mM, respectively. The reaction was conducted precisely for 4 min at 20°C and terminated by adding equal volume of trichloro acetic acid (15% w/v). The mixture was centrifuged at 1500 rpm for 10 min and liberated inorganic phosphate (Pi) was determined in supernatants by the method of Fiske and Subarrow.[Citation23]
Estimation of Sulfhydryl (-SH) Groups
Sulfhydryl content was determined essentially by Ellman’s method[Citation24] using 5-5′-dithiobis (2-nitrobenzoic acid)/ DTNB with slight modifications. Briefly, 3 mL actomyosin sample was added to the mixture of phosphate buffer (2 mL) and water (5 mL) to make up the final volume 10 mL. From this mixture, 3 mL aliquot was collected and mixed with 20 μL DTNB (39.6 mg DTNB in 10 mL phosphate buffer, pH 7.2). After incubation for 20 min at 40°C, absorbance at 412 nm was recorded on spectrophotometer (GENESYS 10UV Spectrophotometer). Sample blank was prepared by replacing DTNB with water. Sulfhydryl content were calculated according to Ding et al.[Citation25] by using the extinction co-efficient of 13,600 L/mol.cm. C = A/E × D, where C is molarity of –SH, A is optical density at 412 nm, D is dilution factor, and E is extinction co-efficient 13,600 M−1 cm−1.
Determination of Solubility
For determining solubility, actomyosin samples were centrifuged at 10,000 rpm and 4°C for 20 min. Protein concentration in the supernatants was determined by the Lowry’s method[Citation21] as referred above (under Protein estimation) using bovine serum albumin as standard. The solubility was calculated as the ratio of protein concentration in supernatants after centrifugation and total protein concentration of actomyosin.[Citation26]
where, Cs is the protein concentration in the supernatant after centrifugation and Co is total protein concentration of actomyosin.
Measurement of Turbidity
Turbidity of actomyosin samples were measured by reading absorbance at 320 nm against appropriate blank on GENESYS 10 UV scanning spectrophotometer. Absorbance was plotted against increasing sodium bicarbonate concentration.
Electrophoretic Profiling
Sodium dodecyl sulphate polyacrylamide gel electrophoreses (SDS-PAGE) of different actomyosin samples were carried out according to the protocol described by Laemmli[Citation27] with a few minor modifications. Resolving and stacking gels were 10% (w/v) and 4% (w/v) prepared from stock solution containing acrylamide: bis-acrylamide = 29.2: 0.8; respectively. However, resolving gels also contained 15% (v/v) glycerol, while at final concentration all the buffers contained 0.1% SDS. Actomyosin samples were prepared with SDS sample buffer containing 5% β-ME and boiled for 4 min. After loading 10 μL of each sample onto the gel slot, electrophoresis was carried out at constant voltage (20 V and 80 V) using mini vertical electrophoresis unit (Genetix, India). Following electrophoresis, gels were washed overnight in a solution containing 40% methanol and 10% acetic acid. Subsequently, the gels were stained with 0.1% (w/v) Coomassie brilliant blue R-250, in 40% (v/v) methanol and 10% (v/v) glacial acetic acid and destained in 7% acetic acid.
Documentation and Densitometric Assessment of SDS-PAGE Profiles
Stained SDS-PAGE gels were documented using SONY CYBERSHOT digital camera (Zoom-4X, 14.1 Megapixels) and by direct scanning on an all-in-one HP Deskjet (F370) computer setup. Best gel documents were used for densitometric analysis. Densitometry of the selected gel-scans was done using Scion Imaging software program (Scion Corporation; Beta release 4.0).
Statistical Analyses
All experiments were done in quadruplets and analytical measurements of each were made in triplicates. We did not find any significant variations between quantitative experiments of the same biochemical parameter (e.g., ATPase activities, relative solubility, and turbidity) while assessing effects of various concentrations of sodium bicarbonate on actomyosin complex. Student’s t-test was employed for statistical comparisons. Values were considered significant at P < 0.05.
RESULTS AND DISCUSSION
This study demonstrates the effect of increasing ionic concentrations of sodium bicarbonate (NaHCO3) from 0.2–1.0M in the presence or absence of 3% NaCl on biochemical properties described below as actomyosin. Actomyosin was isolated from breast muscle (Pectoralis major) of broiler chicken, with pH 5.9 ± 0.1. On the other hand, pH with various concentrations of bicarbonate was recorded as 8.4.
Changes in Ca2+- and Mg2+- ATPase Activities
The effect of various concentrations of NaHCO3 on Ca2+-ATPase and Mg2+-ATPase activities is shown in . It is quite obvious that, within the molarity range used here, NaHCO3 inactivates ATPase activities. Profiles of either of the ATPase activities have certain features in common: (1) Ca2+- as well as Mg2+-ATPase activity continuously decline with the increasing concentration of NaHCO3. (2) To increasing NaHCO3 levels, Mg2+-ATPase displays more sensitivity than Ca2+-ATPase, as the decline is faster from 0.2–0.6M. In comparison, 0.2–0.6M NaHCO3 minimally affects Ca2+-ATPase and a rapid decrease follows beyond 0.6M. (3) The presence of NaCl enhances the magnitude of decrease in both ATPase activities without affecting the trend of the curves. (4) The trend of decrease in Mg2+-ATPase displays higher sensitivity to NaCl as compared to Ca2+-ATPase activity. The sensitivity of Mg2+-ATPase to NaCl is more prominent at lower concentration of NaHCO3.
FIGURE 1 Changes in relative Mg2+ -ATPase and Ca2+ -ATPase activity (%) of actomyosin of chicken breast muscle (Pectoralis major) treated with increasing concentration of NaHCO3 (0.2M, 0.4M, 0.6M, 0.8M, and 1M) with (solid lines) or without (dotted lines) NaCl (3%). Mg2+-ATPase and Ca2+-ATPase activity of untreated actomyosin taken as control (100%) was 0.98 μmol/mg/min and 1.25 μmol/mg/min), respectively.
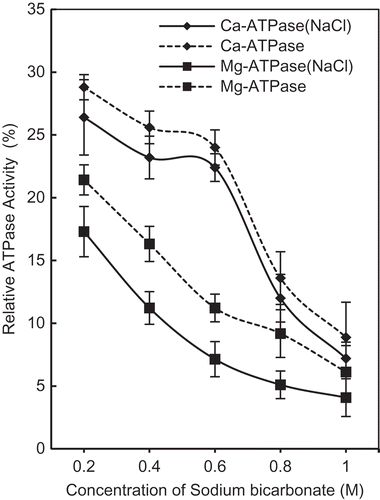
As for functional relevance of ATPase activities, Ca2+-ATPase is recognized as myosin ATPase, while Mg2+-ATPase represents enzymatic activity of actomyosin.[Citation28−Citation30] ATPase is the function of myosin globular head while solubility is determined by rod portion.[Citation31,Citation32] Decline in Ca2+-ATPase activity at higher NaHCO3 concentration may be attributed to the inter- and intra-molecular changes among interacting molecule; specifically myosin and actin. Inhibition of enzyme activity by salts as a consequence of conformational change in myosin head has been previously reported.[Citation33] In addition, ATPase activities are also influenced by factors such as pH, temperature, type of solutes, salt concentrations, or ions present.[Citation34,Citation35] Keeping intimate relationship of Ca2+-ATPase data and myosin () in mind, myosin appears to be more sensitive to NaHCO3 beyond 0.4M, since at high pH value myosin head can undergo irreversible conformational change.[Citation36] Removal of myosin light chain-1 (alkali LC1) at alkaline pH, which is essential for enzymatic activity[Citation37] from actomyosin complex support conformational changes.
Since Mg2+-ATPase is actomyosin ATPase activity and an index of actin-myosin interaction, more rapid decline in Mg2+-ATPase activity at concentrations below 0.6M NaHCO3 is due to decreased actin-myosin interaction (dissociation) and not solely due to changes in myosin conformation, where relative solubility may also have a role. The inference is supported by the fact that except substitution of Mg2+ for Ca2+, all other experimental conditions of ATPase assay were identical. At alkaline pH of NaHCO3, Ca2+- desensitization due to dissociation of regulatory proteins[Citation38] may also affect magnitude of inactivation of Mg2+-ATPase.
The state of myosin and decreased actin-myosin interaction by NaHCO3 (as indicated by Ca2+, Mg2+-ATPases) bring in vitro findings on actomyosin close to their status in marinated muscle where NaHCO3 is a component. More specifically, at elevated ionic strengths actin-myosin interaction is already reported to weaken in pre-rigor muscles.[Citation39,Citation40]
Variation in Solubility
Change in solubility with increasing concentration of NaHCO3 is shown in . Marked increase was observed in the total proteins solubility (˜57%) in the presence of 3% NaCl when NaHCO3 was 0.6M, beyond which protein solubility remained almost unchanged up to 1.0M NaHCO3. However, without NaCl, 0.8M NaHCO3 was required to reach the maximum protein solubility (˜49%), that is 0.2M more NaHCO3 than that needed in the presence of NaCl. Therefore, with NaHCO3 alone, ionic strength compensation is essential, since the ionic strength to achieve highest protein solubility (˜57%) is I = 1.117, that with 3% NaCl is achieved at 0.6M NaHCO3 (). In terms of solubility, effect of NaCl on actomyosin solubility is more pronounced between 0.4M (I = 0.917) and 0.8M (I = 1.317) of NaHCO3. Therefore, most likely reason of solubility variations appears to be the changed surface chemistry of actomyosin constituents at high salt concentrations. It is to reemphasize that myosin is the only high ionic strength soluble component of actomyosin and also the major variable constituent in SDS-PAGE profiles as in obtained SDS-PAGE profiles.
FIGURE 2 Changes in protein solubility (a); and, R-SH contents (b) of chicken breast muscle actomyosin treated with increasing concentration of NaHCO3 (0.2M, 0.4M, 0.6M, 0.8M, and 1M) with or without 3% NaCl. a = compared to control, b = compared to lower treatment, and c = compared to (with NaCl) of respective/same molarity. *p < 0.05.
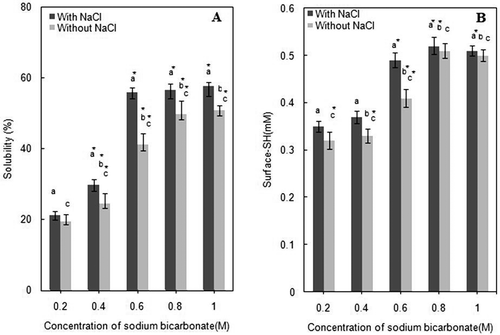
As already pointed out, solubility of muscle proteins also depend on their source, freshness, pH of muscle, type of solvent and, the ionic strengths.[Citation41,Citation42] NaCl is fairly effective in solubilizing substantial amount of major myofibrillar proteins at 2.5% concentration.[Citation42−Citation44] For instance, ˜90% actomyosin proteins were solubilized from Pacific white shrimp at 0.2M NaHCO3.[Citation45] Our data () demonstrates that ˜57% of chicken actomyosin can be solubilized with 0.6M NaHCO3 in the presence of NaCl. Without NaCl, even by the highest molarity of NaHCO3 (1.0M), solubility of actomyosin was rather low (<52%).
FIGURE 3 (a) Changes in turbidity of supernatants of actomyosin obtained after treatment with various molarities of NaHCO3 with or without NaCl. (No adjustment of protein concentration to 0.9 mg/ml was made.) (b) Change in turbidity of supernatants of actomyosin treated with NaHCO3 with or without NaCl. (Protein concentration in all samples were adjusted to 0.9 mg/ml.)
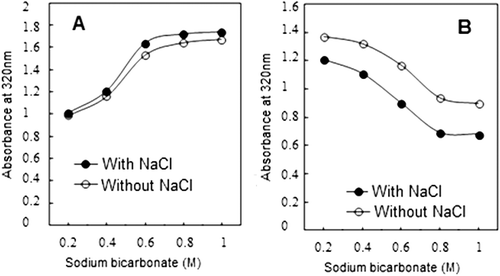
The decreased solubility of myofibrillar proteins has been correlated with pale, soft, and exudative poultry.[Citation46] Solubility, on the other hand, is related to functional properties of food and is an important quality attribute of poultry meat. According to Bertram and co-workers,[Citation47] the use of bicarbonate assists in higher solubilization of myofibrillar proteins in marinated muscle. The amount of soluble actomyosin is important for gelation of myosin, which is the principal participant in the event of meat processing. The statement is corroborated by in vitro studies carried out with different combinations of actomyosin:myosin[Citation48] or with variable ratios of isolated actin:myosin.[Citation43] At alkaline pH, despite a probable increase in protein solubility,[Citation49] a number of other factors such as water absorption, decreased actin-myosin interaction and conformational status of proteins also deserve consideration, as they may influence the biochemical events related to meat quality.
Effect on Sulfhydryl Groups
The trend of reactive sulfhydryls (R-SH) of actomyosin exposed due to treatment with different concentrations of NaHCO3, with or without NaCl, is compared in . R-SH contents showed significant successive increase up to 0.6M NaHCO3, although major increase occurred between 0.4M and 0.6M. The trend displayed by concentration dependence of R-SH profile on NaHCO3 concentration is compatible with solubility profiles of actomyosin from 0.4M to 0.6M of NaHCO3 (). Inclusion of 3% NaCl exposed relatively higher number of R-SH; but the exposure effect of NaCl diminished beyond 0.8M NaHCO3, as no further increase in R-SH occurred whether NaCl is included or not.
Due to greater sensitivity, most of the work on reactive thiols so far has been carried out on fish muscle or myosin with two main objectives: to compare conformational flexibility by demonstrating relative exposure of buried R-SH[Citation22] or their oxidation during frozen storage or thermal treatments.[Citation29,Citation50] The decline in R-SH contents due to disulfide bond formation is widely accepted index of denaturation that has an intimate correlation with the behavior of a specific muscle during storage.[Citation45,Citation47,Citation51] In the present study, increase in R-SH contents is probably caused by the progressive exposure of R-SH groups as a consequence of changed molecular behavior at high ionic strengths and pH.
The increase in R-SH contents with increasing salt concentration has generally been reported for fish myosin.[Citation52] In case of chicken breast muscle myosin, a direct correlation has been reported between oxidation of R-SH and aggregation and gelation, and viscoelastic properties of the formed gel.[Citation53] Since the relative stabilities of muscle proteins (specifically myosin) are major influence of behavior of a muscle, magnitude of R-SH exposure may be lower in chicken.
Variation in Turbidity
Change in turbidity with increasing concentration of NaHCO3 is shown in . Turbidity of actomyosin is highest at 0.2M NaHCO3 that gradually decreases with the increasing concentration of bicarbonate salt. Non-significant differences are observed at 0.8M and 1.0M (P > 0.05). The presence of 3% NaCl showed significant decline in turbidity at lower concentration of NaHCO3. Actomyosin is fairly turbid or opaque protein complex and therefore, decrease in turbidity may indicate dispersion of the actomyosin complex while increase in turbidity indicates aggregation.[Citation54−Citation56] Our results show that at lower concentration of NaHCO3, actomyosin complex is comparatively less soluble () and thereby, more turbid. At higher NaHCO3 concentration, actomyosin solubility is fairly high which seems to be the apparent cause of decline in turbidity. The data on turbidity profiles of supernatants demonstrate increase in turbidity with the molarities of NaHCO3 in presence or absence of NaCl (). However, adjusting the protein concentrations (0.9 mg/mL) reversed the trend of turbidity profile in supernatants indicating that more protein is solubilized by augmented molarities of NaHCO3 in presence or absence of NaCl ().
Electrophoretic Profiling by SDS-PAGE
SDS-PAGE analysis of actomyosin samples treated with various concentrations of NaHCO3 and 3% NaCl did not reveal any comparative change in profiles (data not shown). All the polypeptides of known molecular weight present in the typical profiles of chicken actomyosin were intact. Therefore, similar to turbidity and solubility comparison, we attempted to fractionate actomyosin into soluble supernatant and insoluble sediment (pellet) by centrifugation. Results obtained on fractionated samples are shown in . Various polypeptides corresponding to approximate molecular weights documented in various previous studies were: (Myosin Heavy Chain (˜200 kD), Actin (˜44 kD), Tropomyosin (˜39 kD), Troponin-T (˜35 kD), Troponin-I (˜23 kD), Troponin-C (˜20 kD), and Myosin Light Chains I, II & III (˜25 kD, 18 kD, and 16 kD).
FIGURE 4 SDS-PAGE (10%) profiles of chicken actomyosin treated with 0.2M, 0.4M, 0.6M, 0.8M, and 1M NaHCO3 for 30 min. Treated samples of actomyosin were fractionated into supernatant (a) and pellet (b) and the SDS samples prepared were loaded in equal volume (10 μL) in each lane. (a) Supernatant: Lanes 1–5 supernatants of actomyosin samples treated with 0.2M, 0.4M, 0.6M, 0.8M, and 1M NaHCO3 along with 3% NaCl. MyHC = Myosin Heavy Chain; TM = Tropomyosin; TN = Troponin; MLCI = Myosin Light Chain I; TN-I = Troponin-I; TN-C = Troponin-C; MLC-II = Myosin Light Chain II and MLC-III = Myosin Light Chain-III. (b) Pellet: Lane 1 = Control; Lanes 2–6 pellet of actomyosin samples treated with 0.2M, 0.4M, 0.6M, 0.8M, and 1M NaHCO3 along with 3% NaCl.
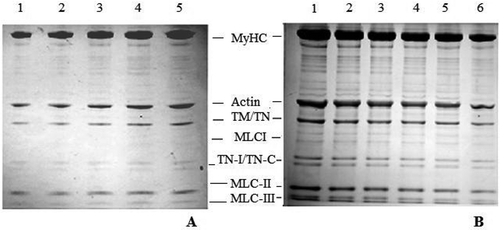
demonstrates that the increasing concentration of NaHCO3 in the presence of NaCl causes an increase in solubility of myosin heavy chain, actin and the regulatory proteins (tropomyosin and troponin-T, troponin-I and troponin-C). Comparison of changed ratios of these proteins in the soluble (supernatant) and insoluble (residual pellet) fractions are made in and with the quantitative representation in and . Quantitation of relative intensities of various bands in SDS-PAGE profiles was made with the help of ScionImage software in terms of integrated density value (IDV). As calculated from IDV, NaHCO3 concentration-dependent major quantitative change occurred in myosin heavy chain or MyHC ( and ). According to IDV analyses gradient of changes in actin, regulatory proteins and light chains including the essential LC1 corresponded to continued increase in NaHCO3 from 0.2M to 1.0M.
FIGURE 5 Densitometry profiles of Myosin Heavy Chain (MyHC) and Actin as stacked in 10% SDS-PAGE profiles of chicken actomyosin treated with 0.2M, 0.4M, 0.6M, 0.8M, and 1M NaHCO3 with 3% NaCl. Integrated density value as relative IDV (%) of MyHC, actin, and the regulatory proteins along with released essential Myosin Light Chain (LC-1) in various supernatants of treated samples are compared in (a). Similarly, IDV data of pellet is compared in (b). Horizontal axis in either case is NaHCO3 concentration. For the sake of clarity and to avoid crowding, IDV changes in other light chains are not shown in IDV diagrams.
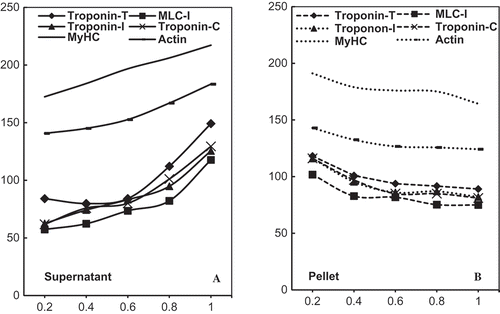
The results thus show that not only the MyHC dimer but LCs and regulatory proteins also undergo dissolution/dissociation in course of NaHCO3 induced changes. Extraction and subsequent solubilization of these polypeptides from chicken myofibrils by phosphates, at higher NaCl concentration, are also reported in earlier studies.[Citation44] However, the most important point is that the extractability and other physicochemical properties as evaluated here remained far below the fraction of actomyosin proteins which could not be solubilized (). ATPase and electrophoretic data also supports this observation, since more of the proteins go with the pellet ().
Although the approach applied here provides new information on the behavior of isolated actomyosin, the solvent-system used caused substantial change in physicochemical properties of actomyosin complex. High concentration of sodium bicarbonate may not be a suitable component of marinades, because of adverse effects on functional properties and integrity of actomyosin. The experimental design, however, is useful to compare differently stored muscle or under different state of rigor-mortis, where solubility and other physicochemical characteristics chosen here are matters of concern.
CONCLUSIONS
Properties of isolated myofibrillar proteins are important in adding to our understanding about their meat source. This study revealed that, under our experimental conditions, it is overall increase in salt molarity (NaHCO3 or NaCl) which is bringing about observed inter- and intra-molecular changes in isolated actomyosin; in particular the myosin molecule. The relevance with myosin is apparent since active site is located in globular head of myosin whereas rod of the molecule determines solubility. Substructural changes, such as dissociation of myosin light chains and regulatory proteins of actomyosin complex also correspond to high molarity of NaHCO3. Moreover, additive effect of NaCl to overall molar concentration explains the conspicuous changes in physicochemical properties of actomyosin even at the lowest concentration of NaHCO3. Although improved solubility of myofibrillar proteins is a positive indicator for processed meat products, high sodium levels are an obvious disadvantage.
ACKNOWLEDGMENTS
The authors thank the Chairman, Department of Zoology, Aligarh Muslim University, Aligarh for providing necessary laboratory facilities. Help extended by Ms. Areeba Ahmad is also gratefully acknowledged.
FUNDING
The work was done out of the financial assistance from MRP by the UGC, New Delhi [F. No. 39-581/2010 (SR)] to AH and from UGC-Non-NET Fellowship to RS.
Additional information
Funding
REFERENCES
- Barbut, S.; Zhang, L.; Marcone, M. Effects of pale, normal, and dark chicken breast meat on microstructure, extractable proteins, and cooking of marinated fillets. Poultry Science 2005, 84 (5), 797–802.
- Van Laack, R.L.; Liu, C.H.; Smith, M.O.; Loveday, H.D. Characteristics of pale, soft, exudative broiler breast meat. Poultry Science 2000, 79 (7), 1057–1061.
- Fletcher, D.L. Poultry meat quality. World’s Poultry Science Journal 2002, 58, 131–145.
- Xiong, Y.L.; Lou, X.; Wang, C.; Moody, W.G.; Harmon, R.J. Protein extraction from chicken myofibrils irrigated with various polyphosphate and NaCl solutions. Journal of Food Science 2000, 65 (1), 96–100.
- Woelfel, R.L.; Sams, A.R. Marination performance of pale broiler breast meat. Poultry Science 2001, 80, 1519–1522.
- Alvarado, C.Z.; Sams, A.R. Injection marination strategies for remediation of pale, exudative broiler breast meat. Poultry Science 2003, 82, 1332–1336.
- Kauffman, R.G.; Van Laack, R.L.; Russell, R.L.; Pospiech, E.; Cornelius, C.A.; Suckow, C.E.; Greaser, M.L. Can pale, soft, exudative pork be prevented by postmortem sodium bicarbonate injection? Journal of Animal Science 1998, 76 (12), 3010–3015.
- Wynveen, E.J.; Bowker, B.C.; Grant, A.L.; Lamkey, J.W.; Fennewald, K.J.; Henson, L.; Gerrard, D.E. Pork quality is affected by early postmortem phosphate and bicarbonate injection. Journal of Food Science 2001, 66 (6), 886–891.
- Sen, A.R.; Naveena, B.M.; Muthukumar, M.; Babji, Y.; Murthy, T.R.K. Effect of chilling, polyphosphate, and bicarbonate on quality characteristics of broiler breast meat. British Poultry Science 2005, 46, 451–456.
- Petracci, M.; Laghi, L.; Rocculi, P.; Rimini, S.; Panarese, V.; Cremonini, M.A.; Cavani, C. The use of sodium bicarbonate for marination of broiler breast meat. Poultry Science 2012, 91, 526–534.
- Petracci, M.; Laghi, L.; Rimini, S.; Rocculi, P.; Capozzi, F.; Cavani, C. Chicken breast meat marinated with increasing levels of sodium bicarbonate. The Journal of Poultry Science 2013, DOI:http://dx.doi.org/10.2141/jpsa.0130079.
- Xiong, Y.L.; Kupski, D.R. Time-dependent marinade absorption and retention, cooking yield, and palatability of chicken filets marinated in various phosphate solutions. Poultry Science 1999, 78, 1053–1059.
- Lopez, K.; Schilling, M.W.; Armstrong, T.W.; Smith, B.S.; Corzo, A. Sodium chloride concentration affects yield, quality, and sensory acceptability of vacuum-tumbled marinated broiler breast fillets. Poultry science 2012, 91 (5), 1186–1194.
- Acton, J.C.; Ziegler, G.R.; Burge, J.D.L.; Froning, G.W. Functionality of muscle constituents in the processing of comminuted meat products. Critical Reviews in Food Science and Nutrition 1982, 18 (2), 99–121.
- Xiong, Y.L. Structure-function relationships of muscle proteins. Food Science and Technology-New York-Marcel Dekker 1997, 341–392.
- Samejima, K.; Ishioroshi, M.; Yasui, T. Relative roles of the head and tail portions of the molecule in heat‐induced gelation of myosin. Journal of Food Science 1981, 46 (5), 1412–1418.
- Cofrades, S.; Careche, M.; Carballo, J.; Colmenero, F.J. Freezing and frozen storage of actomyosin from different species. Zeitschrift für Lebensmittel-Untersuchung und Forschung 1996, 203, 316–319.
- Bertram, H.C.; Kristensen, M.; Andersen, H.J. Functionality of myofibrillar proteins as affected by pH, ionic strength, and heat treatment—A low-field NM study. Meat Science 2004, 68, 249–256.
- Xiang, D.S.; Holley, R.A. Factors influencing gel formation by myofibrillar proteins in muscle foods. Comprehensive Reviews in Food Safety 2011, 10, 33–51.
- Ahmad, R.; Hasnain, A. Correlation between biochemical properties and adaptive diversity of skeletal muscle myofibrils and myosin of some air-breathing teleosts. Indian Journal of Biochemistry and Biophysics 2006, 43 (4), 217.
- Lowry, O.H.; Rosebrough, N.J.; Farr, A.L.; Randall, R.J. Protein measurement with the folin phenol reagent. Journal of Biological Chemistry 1951, 193, 265–275.
- Hasnain, A.; Samejima, K.; Takahashi, K.; Yasui, T. Molecular adaptability of carp myosin: A study of some physico-chemical properties and their comparison with those of rabbit myosin. Archives of Physiology and Biochemistry 1979, 87 (4), 643–662.
- Fiske, C.H.; Subbarow, Y. The colorimetric determination of phosphorus. Journal of Biological Chemistry 1925, 66 (2), 375–400.
- Ellman, G.L. Tissue sulfhydryl groups. Archives of Biochemistry and Biophysics 1959, 82 (1), 70–77.
- Ding, Y.; Wei-guo, S.; Yan, C. Comparative studies on the biochemical characteristics of natural actomyosin isolated from PSE and normal pork. Journal of Zhejiang University Science 2004, 5 (6), 684–688.
- Liu, R.; Zhao, S.M.; Yang, H.; Li, D.D.; Xiong, S.B.; Xie, B.J. Comparative study on the stability of fish actomyosin and pork actomyosin. Meat Science 2011, 88 (2), 234–240.
- Laemmli, U.K. Cleavage of structural proteins during the assembly of the head of bacteriophage T4. Nature 1970, 227, 680–685.
- Matsuura, M.; Arai, K. Effect of pH on filament-forming ability and biochemical activity of fish myosin. Nippon Suisan Gakkaishi 1986, 52 (9), 1657–1663.
- Roura, S.I.; Crupkin, M. Biochemical and functional properties of myofibrils from pre- and post-spawned hake (Merluccius hubbsi marini) stored on ice. Journal of Food Science 1995, 60, 269–272.
- Ahmad, R.; Hasnain, A. Peptide mapping of polymorphic myosin heavy chain isoforms in different muscle types of some freshwater teleosts. Fish Physiology and Biochemistry 2013, 39, 721–731.
- Rayment, I. The structural basis of the myosin ATPase activity. Journal of Biological Chemistry 1996, 271 (27), 15850–15853.
- Harrington, W.F.; Rodgers, M.E. Myosin. Annual review of biochemistry 1984, 53 (1), 35–73.
- Warren, J.C., Stowring, L.; Morales, M.F. The effect of structure-disrupting ions on the activity of myosin and other enzymes. Journal of Biological Chemistry 1966, 241 (2), 309–316.
- Yasui, T.; Gotoh, T.; Morita, J. Influence of pH and temperature on properties of myosin A in glycerol-treated fiber bundles. Journal of Agricultural and Food Chemistry 1973, 21 (2), 241–246.
- Watabe, S.; Hashimoto, K.; Watanabe, S. The pH-dependency of myosin ATPases from yellowtail ordinary and dark muscles. Journal of Biochemistry 1983, 94 (6), 1867–1875.
- Kawakami, H.; Morita, J.I.; Takahashi, K.; Yasui, T. Thermal denaturation of myosin, heavy meromyosin and subfragment-1. Journal of Biochemistry 1971, 70 (4), 635–648.
- Sweeney, H.L. Function of the N terminus of the myosin essential light chain of vertebrate striated muscle. Biophysical Journal 1995, 68 (4 Suppl), 112S.
- Ebashi, S. Regulatory mechanism of muscle contraction with special reference to the Ca-troponin-tropomyosin system. Essays in Biochemistry 1974, 10, 1.
- Yu, L.P.; Lee, Y.B. Effects of postmortem pH and temperature muscle structure and meat tenderness. Journal of Food Science 1986, 51 (3), 774–780.
- Wu, F.Y.; Smith, S.B. Ionic strength and myofibrillar protein solubilization. Journal of Animal Science 1987, 65 (2), 597–608.
- Lan, Y.H.; Novakofski, J.; Carr, T.R.; McKeith, F.K. Assay and storage conditions affect yield of salt soluble protein from muscle. Journal of Food Science 1993, 58 (5), 963–967.
- Chang, H.S.; Hultin, H.O.; Dagher, S.M. Effect of MgCl2/sodium pyrophosphate on chicken breast muscle myosin solubilization and gelation. Journal of Food Biochemistry 2001, 25 (5), 459–474.
- Wang, S.F.; Smith, D.M. Gelation of chicken breast muscle actomyosin as influenced by weight ratio of actin to myosin. Journal of Agricultural and Food Chemistry 1995, 43 (2), 331–336.
- Xiong, Y.L.; Lou, X.; Harmon, R.J.; Wang, C.; Moody, W.G. Salt- and pyrophosphate-induced structural changes in myofibrils from chicken red and white muscles. Journal of Science and Food Agriculture 2000, 80, 1176–1182.
- Chantarasuwan, C.; Benjakul, S.; Visessanguan, W. The effects of sodium bicarbonate on conformational changes of natural actomyosin from Pacific white shrimp (Litopenaeus vannamei). Food Chemistry 2011, 129, 1636–1643.
- Pietrzak, M.; Greaser, M.L.; Sosnicki, A.A. Effect of rapid rigor mortis processes on protein functionality in pectoralis major muscle of domestic turkeys. Journal of Animal Science 1997, 75 (8), 2106–2116.
- Bertram, H.C.; Meyer, R.L.; Wu, Z.; Zhou, X.; Andersen, H.J. Water distribution and microstructure in enhanced structure in pork. Journal of Agricultural and Food Chemistry 2008, 56 (16), 7201–7207.
- Yasui, T.; Ishioroshi, M.; Samejima, K. Effect of actomyosin on heat gelation of myosin. Agricultural and Biological Chemistry 1982, 46 (4), 1049–1059.
- Mohan, M.; Ramachandran, D.; Sankar, T.V.; Anandan, R. Influence of pH on the solubility and conformational characteristics of muscle proteins from mullet (Mugil cephalus). Process Biochemistry 2007, 42 (7), 1056–1062.
- Wen-Ching, K.; Yu, C.C.; Hsu, K.C. Changes in conformation and sulfhydryl groups of tilapia actomyosin by thermal treatment. LWT-Food Science and Technology 2007, 40 (8), 1316–1320.
- Benjakul, S.; Visessanguan, W.; Ishizaki, S., Tanaka, M. Differences in gelation characteristics of natural actomyosin from two species of bigeye snapper, Priacanthus tayenus, and Priacanthus macracanthus. Journal of Food Science 2001, 66 (9), 1311–1318.
- Tein, L.M.; Jae, W.P. Solubility of salmon myosin as affected by conformational changes at various ionic strengths and pH. Journal of Food Science 1998, 63 (2). 215–218.
- Smyth, A.B.; Smith, D.M.; O’Neill, E. Disulfide bonds influence the heat-induced gel properties of chicken breast muscle myosin. Journal of Food Science 1998, 63 (4), 584–587.
- Lymn, R.W.; Taylor, E.W. Mechanism of adenosine triphosphate hydrolysis by actomyosin. Biochemistry 1971, 10 (25), 4617–4624.
- Juan, Y.; Pan, J.; Shen, H.; Luo, Y. Changes in physicochemical properties of bighead carp (Aristichthys mobilis) actomyosin by thermal treatment. International Journal of Food Properties 2012, 15 (6), 1276–1285.
- Mothershaw, A.N.; Gaffer, T.; Kadim, I.; Guizani, N.; Al-Amri, I.; Mahgoub, O.; Al-Bahry S. Quality characteristics of broiler chicken meat on salt at different temperatures. International Journal of Food Properties 2009, 12 (3), 681–690.