Abstract
In order to purify proteinase from Lactobacillus delbrueckii subsp. bulgaricus, chromatography methods including ion-exchange and hydrophobic interaction were combined. Chromatographic conditions were structured and optimized. The final sample volume, flow rate, and length of gradient for anion exchange chromatography were 20 mL, 5 mL/min and 10 column volume length of gradient, in that order. Besides, the flow rate and length of gradient for hydrophobic interaction chromatography were 1 mL/min and 10 column volume. Proteinase was purified up to 43-fold with a specific activity of 54.4 U/mg protein, and the recovery of 46.6%. The molecular weight of the purified proteinase was about 40 kDa.
INTRODUCTION
Lactic acid bacteria (LAB) such as Streptococcus thermophilus, Lactobacillus lactis, Lactobacillus helveticus, and Lactobacillus delbrueckii subsp. bulgaricus have been widely used as dairy starter cultures and are of utmost economic importance.[Citation1] LAB proteinases are known to contribute positively to the texture and flavor formation of many fermented dairy products.[Citation2,−Citation4] The proteolytic activity of LAB strains has been reported to exert a beneficial effect on the formation and stability of the milk protein gel,[Citation5] and enzymatic hydrolysis of casein by proteinases can present yogurt with different firmness, viscosity, and the degree of syneresis.[Citation6,Citation7]
As fastidious microorganisms, LAB require many free amino acids which are not sufficient for sustaining growth in milk. Thus, their progress in milk is depending on the presence of a complex proteolytic system allowing hydrolysis of milk caseins.[Citation8] The proteolytic system of LAB is mainly composed of a proteinase involved in the initial cleavage of casein, peptidases that can hydrolyze the large peptides formed from casein and transport systems translocating oligopeptides across the bacterial membrane which are involved in the uptake of small peptides and amino acids.[Citation2,Citation9] The proteolytic system of Lb. lactis was investigated to the extent that a complete framework model for casein breakdown, transport, degradation of casein-derived peptides[Citation10−Citation12] and regulation thereof, was established.[Citation13−Citation15] The cell-envelope proteinase of LAB is a key enzyme in the proteolytic system as it primarily degrades casein for the rapid growth of LAB in milk. In recent years, cell-envelope proteinases from different strains of LAB have been purified and characterized. These include proteinase from Lb. casei subsp. casei IFPL731 (by ammonium sulfate precipitation, hydrophobic interaction and ion-exchange chromatography),[Citation16] Lb. delbrueckii subsp. bulgaricus CNRZ397 (by ultra-filtration and ion-exchange chromatography),[Citation17] and Lb. casei DI-1 (by ammonium sulfate precipitation and chromatograph on DEAE-Sephadex A-25 and Sephadex G-100).[Citation18]
In the present study, Lb. delbrueckii subsp. bulgaricus CH9-3 4.0 was selected to purify its proteinase for its technological characteristic. The purpose of this study was to optimize the purification of LAB proteinase. The purified proteinase with high activity is currently underlying mechanism on how proteinase improves the quality of yogurt.
MATERIALS AND METHODS
Microorganism and Culture
The strain of Lb. delbrueckii subsp. bulgaricus CH9-3 4.0 was isolated from the Chinese traditional fermented dairy product by our group.[Citation19] It was routinely propagated in the Man-Rogosa-Sharpe (MRS) broth[Citation20] and kept frozen (–80°C) in MRS broth supplemented with 20% (vol/vol) glycerol. The strain has been preserved at the School of Food Science and Engineering (Harbin Institute of Technology, Harbin, China). It was subcultured twice successively in reconstituted skim milk (Nestlé, Harbin, China) at 37°C for 18 h before use.
Measurement of Proteinase Activity
The assay for proteolytic activity was carried out as described by Christen and Marshall.[Citation21] Briefly, it involved combining 0.3 mL of the azocasein (Sigma-Aldrich, MO, USA) solution (50 mmol/L phosphate buffer, pH 7.0) with 0.3 mL enzyme solution. The content of the tubes was mixed and incubated at 37°C for 1 h. The reaction was stopped by the addition of 0.6 mL of 12% (wt/vol) trichloroacetic acid (TCA). The absorbance of the supernatant was measured at 345 nm using a UV spectrophotometer (Ultrospec 1100 pro, GE, NJ, USA). One unit of enzyme activity (U) was defined as the amount of enzyme enhancing the absorbance of 0.01 per hour at 37°C. The concentration of protein was estimated by the Bradford method,[Citation22] using the Coomassie protein assay. Bovine serum albumin was used as a standard.
Ultra-Filtration of Cell-Free Extract
The strain of Lb. delbrueckii subsp. bulgaricus CH9-3 4.0 was first grown in MRS broth at 37°C for 20 h. The cells were then harvested by centrifugation at 4000 g for 10 min at 4°C, and washed three times with 50 mmol/L Tris–HCl buffer (pH 7.0). The washed cells were re-suspended in the same buffer containing 1 mg/mL lysozyme, and incubated for 3 h at 37°C. The cell debris was removed by centrifugation at 10,000 g for 15 min at 4°C. The clear supernatant was passed through a 0.22 μm filter membrane to remove the bacteria. The filtered solution was immediately concentrated by ultra-filtration using a Millipore Lab-scale TFF System (Millipore, MA, USA) with a 10 kDa cut-off.[Citation23]
Ion-Exchange Chromatography
Concentrated cell-free extracts (10, 20, and 30 mL) were loaded on a DEAE-52 column (1.6 × 10 cm; GE, NJ, USA), previously equilibrated with 50 mmol/L Tris-HCl buffer (pH 7.0). The column was washed extensively with the same buffer and eluted with a linear gradient of 1.0 mol/L of NaCl in the same buffer for 5, 10, and 15 column volume (CV) length of gradient at flow rate of 1, 3, and 5 mL/min. Fractions of 5 mL were collected and assayed for enzyme activity. The purification system was an ÄKTA purifier 100 system (GE, NJ, USA), which was equipped with a P-900 series pump, an UV-900 monitor, a pH/C-900 monitor, a M-925 mixer, a complete set of motor valves, a Frac-950 fraction collector, an A-900 auto-sampler, and an AD-900 analog/digital converter connected to a 10A refractive index detector.
TABLE 1 Summary of the purification steps
Hydrophobic Interaction Chromatography
The enzyme solution after DEAE cellulose chromatography was loaded onto a Hitrap Butyl FF column (1 mL; GE, NJ, USA) equilibrated with 1.0 mol/L (NH4)2SO4 in 50 mmol/L phosphate buffer (pH 7.0) which was used as a starting buffer. The non-interacting proteins were eluted with the starting buffer, and the column was eluted with a linear gradient of 1.0 mol/L of (NH4)2SO4 by 50 mmol/L phosphate buffer (eluting buffer) for 10, 15, and 20 CV length of gradient at flow rate of 0.5, 1.0, and 1.5 mL/min. Fractions with enzyme activity were collected, and then the active fraction was diafiltered in an ultra-filtration cup with a 10 kDa cut-off. All purification steps were performed at 4°C.
Sodium Dodecyl Sulfate-Polyacrylamide Gel Electrophoresis (SDS-PAGE)
SDS-PAGE was carried out for the determination of both the enzyme purity and its molecular weight as described by Laemmli[Citation24] with a 5% (wt/vol) acrylamide stacking gel and a 12% (wt/vol) acrylamide running gel. Protein in the gel was stained with Coomassie Blue R250. The molecular weight of proteinase was determined by a molecular weight calibration kit as a marker.
RESULTS AND DISCUSSION
Ultra-Filtration of Cell-Free Extract
The cell-free extract was concentrated to 20% of the original volume by ultra-filtration with a 10 kDa cut-off, and the recovered activity was about 105.8% (). Compared to other studies, the recovery activity in this study was higher than that of the proteinase from Lb. delbrueckii subsp. bulgaricus CNRZ 397 by ultra-filtration (recovery of 90%),[Citation17] and much higher than using the ammonium sulfate precipitation method (recovery of 42%),[Citation25] thereby suggesting that it was appropriate to concentrate the cell-free extract.
Ion-Exchange Chromatography
The DEAE-52 chromatography led to the separation of two substantial fractions. The first fraction showed the highest enzyme activity at sample volume of 10, 20, and 30 mL (--), respectively. As for estimation, 20 mL of concentrated cell-free extract was initially loaded on a DEAE-52 column and eluted for 5 CV length of gradient, and the yield of proteinase activity had a maximum of 35.5% at a flow rate of 5 mL/min (). Thereafter, optimization was done to elute for 10 CV length of gradient, which yielded the enzyme activity of 70.6% at the optimum flow rate (). Compared to sample volume of 10 and 30 mL (), it was clearly seen that the sample volume of 20 mL gave the highest yield of 70.6% enzyme activity. The optimum flow rate in this study was much higher than the flow rate of 23 mL/h by using DEAE-Sephadex A-25,[Citation25] or at 1 mL/min with a Mono Q chromatography.[Citation17] However, little has been done on how the length of gradient and sample volume of chromatography affects the yield of proteinase activity.
FIGURE 1 Effects of different sample volume on DEAE cellulose DE-52 column chromatography. (a–c) Elution curves of DEAE cellulose chromatography with sample volume of 10, 20, and 30 mL.
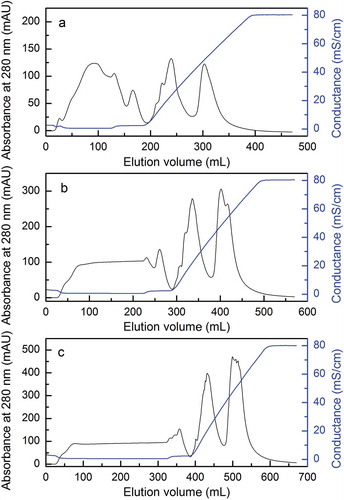
FIGURE 2 The yield of proteinase activity with different flow rates, length of gradient, and sample volume of DEAE cellulose DE-52 column chromatography: (a) yield of proteinase activity with flow rates of 1, 3, and 5 mL/min; (b) yield of proteinase activity with 5, 10, and 15 CV length of gradient; (c) yield of proteinase activity with sample volume of 10, 20, and 30 mL.
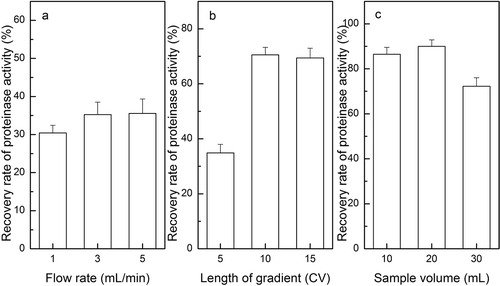
Hydrophobic Interaction Chromatography
The second peak on the Hitrap Butyl FF chromatography in showed the proteinase activity after initial purification by the DEAE-52 chromatography. As shown in , the elution curves of hydrophobic interaction chromatography seemed similar with the 10, 15, and 20 CV length of gradient (--). It was demonstrated that the yield of enzyme activity was high about 69.5% eluted at a flow rate of 1 mL/min (). Meanwhile, optimization was done to elute for 10 CV length of gradient, which yielded the enzyme activity of 74.1% at the optimum flow rate and the yield of enzyme activity decreased with the increased length of gradient (). Our result on recovery enzyme activity was fairly similar to that of Lb. helveticus L89 reported by other research on a Fractogel TSK butyl-650 column,[Citation26] and significantly higher than that of Lb. casei subsp. casei IFPL 731 which was purified on Phenyl-Superose HR 5/5 column.[Citation27] These results indicated that the butyl of low hydrophobic chromatography was more suitable for the purification of LAB proteinases.
Proteinase Purification
There were many kinds of protein with a large variety of molecular weight in the crude enzyme after ultra-filtration (Lane 1 in ). However, the proteinase was extracted after the DEAE chromatography step and the content of the proteinase was much higher (Lane 2 in ). The enzyme obtained from the final Hitrap Butyl FF chromatograph operation showed a single protein band by SDS-PAGE (Lane 3 in ) and its molecular weight was about 40 kDa quantified with Quantity One Software (Bio-Rad). On the other hand, the molecular weight for the cell envelope proteinase of Lb. delbrueckii subsp. bulgaricus reported by others was higher to 170 kDa.[Citation17] The specific enzyme activity observed with azo-casein as a substrate after each purification step was summarized in . The enzyme was purified about 43-fold from the cell-free extract by ultra-filtration, two steps of column chromatography. The recovery of proteinase activity was about 46.6%.
CONCLUSION
Proteinase produced by Lb. delbrueckii subsp. bulgaricus CH9-3 4.0 was purified. The purification of the enzyme was achieved by ultra-filtration, ion-exchange chromatography on DEAE-52, and hydrophobic interaction chromatography on Hitrap Butyl FF column. After the final step of purification, the enzyme was purified 43-fold with a specific activity of 54.4 U/mg, and fair recovery of 46.6%. The purified enzyme was homogenous on SDS-PAGE, and its molecular weight was estimated to be about 40 kDa. The purified proteinase is currently under further research to investigate the mechanisms of improving yogurt quality during curding.
FUNDING
This work was supported financially by the National Natural Science Foundation of China (31271906/C200204), National High-Tech R&D Program Grants from the Ministry of Science and Technology of the People’s Republic of China (2011AA100902), and Special Financial Grant from China Postdoctoral Science Foundation (2012T50346 and 2013M541397).
Additional information
Funding
REFERENCES
- Savijoki, K.; Ingmer, H.; Varmanen, P. Proteolytic systems of lactic acid bacteria. Applied Microbiology and Biotechnology 2006, 71, 394–406.
- Law, J.; Haandrikman, A. Proteolytic enzymes of lactic acid bacteria. International Dairy Journal 1997, 7, 1–11.
- Sadat-Mekmene, L.; Jardin, J.; Corre, C.; Molle, D.; Richoux, R.; Delage, M.M.; Lortal, S.; Gagnaire, V. Simultaneous presence of PrtH and PrtH2 proteinases in Lactobacillus helveticus strains improves breakdown of the pure alphas1-casein. Applied and Environmental Microbiology 2011, 77, 179–186.
- Hayaloglu, A.A.; Karabulut, I. Primary and secondary proteolysis in eleven Turkish cheese varieties. International Journal of Food Properties 2013, 16, 1663–1675.
- Laws, A.P.; Marshall, V.M. The relevance of exopolysaccharides to the rheological properties in milk fermented with ropy strains of lactic acid bacteria. International Dairy Journal 2001, 11, 709–721.
- Gassem, M.A.; Frank, J.F. Physical properties of yogurt made from milk treated with proteolytic enzymes. Journal of Dairy Science 1991, 74, 1503–1511.
- Ruas-Madiedo, P.; Alting, A.C.; Zoon, P. Effect of exopolysaccharides and proteolytic activity of Lactococcus lactis subsp. cremoris strains on the viscosity and structure of fermented milks. International Dairy Journal 2005, 15, 155–164.
- Sadat-Mekmene, L.; Genay, M.; Atlan, D.; Lortal, S.; Gagnaire, V. Original features of cell-envelope proteinases of Lactobacillus helveticus. A review. International Journal of Food Microbiology 2011, 146, 1–13.
- Kunji, E.R.; Mierau, I.; Hagting, A.; Poolman, B.; Konings, W.N. The proteotytic systems of lactic acid bacteria. Antonie van Leeuwenhoek 1996, 70, 187–221.
- Foucaud, C.; Kunji, E.; Hagting, A.; Richard, J.; Konings, W.N.; Desmazeaud, M.; Poolman, B. Specificity of peptide transport systems in Lactococcus lactis: Evidence for a third system which transports hydrophobic di-and tripeptides. Journal of Bacteriology 1995, 177, 4652–4657.
- Hagting, A.; Kunji, E.; Leenhouts, K.J.; Poolman, B.; Konings, W.N. The di-and tripeptide transport protein of Lactococcus lactis. A new type of bacterial peptide transporter. Journal of Biological Chemistry 1994, 269, 11391–11399.
- Tynkkynen, S.; Buist, G.; Kunji, E.; Kok, J.; Poolman, B.; Venema, G.; Haandrikman, A. Genetic and biochemical characterization of the oligopeptide transport system of Lactococcus lactis. Journal of Bacteriology 1993, 175, 7523–7532.
- Detmers, F.J.M.; Kunji, E.R.S.; Lanfermeijer, F.C.; Poolman, B.; Konings, W.N. Kinetics and specificity of peptide uptake by the oligopeptide transport system of Lactococcus lactis. Biochemistry 1998, 37, 16671–16679.
- den Hengst, C.D.; Curley, P.; Larsen, R.; Buist, G.; Nauta, A.; van Sinderen, D.; … Kok, J. Probing direct interactions between CodY and the oppD promoter of Lactococcus lactis. Journal of Bacteriology 2005, 187, 512–521.
- Guédon, E.; Renault, P.; Ehrlich, S.D.; Delorme, C. Transcriptional pattern of genes coding for the proteolytic system of Lactococcus lactis and evidence for coordinated regulation of key enzymes by peptide supply. Journal of Bacteriology 2001, 183, 3614–3622.
- Fernández de Palencia, P.; Pelaez, C.; Romero, C.; Martin-Hernandez, M. Purification and characterization of the cell wall proteinase of Lactobacillus casei subsp. casei IFPL 731 isolated from raw goat’s milk cheese. Journal of Agricultural and Food Chemistry 1997, 45, 3401–3405.
- Laloi, P.; Atlan, D.; Blanc, B.; Gilbert, C.; Portalier, R. Cell-wall-associated proteinase of Lactobacillus delbrueckii subsp. bulgaricus CNRZ 397: Differential extraction, purification, and properties of the enzyme. Applied Microbiology and Biotechnology 1991, 36, 196–204.
- Xing, G.Y.; Pan, D.D.; Tong, M.; Zeng, X.Q. Purification and characterization of cell-envelopeproteinase from Lactobacillus casei DI-1. African Journal of Biotechnology 2012, 11, 15060–15067.
- Han, X.; Zhang, L.W.; Yu, P.; Yi, H.X.; Zhang, Y.C. Potential of LAB starter culture isolated from Chinese traditional fermented foods for yoghurt production. International Dairy Journal 2014, 34, 247–251.
- De Man, J.C.; Rogosa, M.; Sharpe, M.E. A medium for the cultivation of lactobacilli. Journal of Applied Microbiology 1960, 23, 130–135.
- Christen, G.L.; Marshall, R.T. Selected properties of lipase and protease of Pseudomonas fluorescens 27 produced in four media. Journal of Dairy Science 1984, 67, 1680–1687.
- Bradford, M.M. A rapid and sensitive method for the quantitation of microgram quantities of protein utilizing the principle of protein-dye binding. Analytical Biochemistry 1976, 72, 248–254.
- Gu, X.; Hou, Y.K.; Li, D.; Wang, J.Z.; Wang, F.J. Separation, purification, and identification of angiotensin I–converting enzyme inhibitory peptides from walnut (Juglans regia L.) hydrolyzate. International Journal of Food Properties 2015, 2, 266–276.
- Laemmli, U.K. Cleavage of structural proteins during the assembly of the head of bacteriophage T4. Nature 1970, 227, 680–685.
- Guo, Y.X.; Pan, D.D.; Zeng, X.Q.; Tanokura, M. Purification and characterization of CEP from Lactococcus lactis ssp. lactis. Food Chemistry 2009, 112, 533–538.
- Martín-Hernández, M.C.; Alting, A.C.; Exterkate, F.A. Purification and characterization of the mature membrane-associated cell-envelope proteinase of Lactobacillus helveticus L89. Applied Microbiology and Biotechnology 1994, 40, 828–834.
- Fernández de Palencia, P.; Peláez, C.; Romero, C.; Martín-Hernández, M.C. Purification and characterization of the cell wall proteinase of Lactobacillus casei subsp. casei IFPL 731 isolated from raw goat’s milk cheese. Journal of Agricultural and Food Chemistry 1997, 45, 3401–3405.