Abstract
The foaming properties of commercial soy protein isolate subjected to different temperatures (20–90°C) were assessed. The results revealed that the solubility and surface hydrophobicity of a 5% (w/v) commercial soy protein isolate suspension increased with increasing temperature, which increased foaming capacity and reduced foaming stability. Commercial soy protein isolate supernatant (i.e., soluble fraction) had higher foaming capacity at low temperatures (20–50°C). A high content of commercial soy protein isolate soluble fraction increased foaming capacity but decreased foaming stability. The SDS-PAGE patterns and molecular weight distribution of commercial soy protein isolate revealed that there were soluble, large molecular weight aggregates (>400 kDa) formed mainly from A and B-11S polypeptides of commercial soy protein isolate via disulfide bonds. Additionally, some aggregates also dissociated into small polypeptides and subunits after heat treatment. Commercial soy protein isolate precipitate (i.e., insoluble fraction) had a high content of proline and cysteine, which probably contributed to the foaming stability of commercial soy protein isolate.
INTRODUCTION
Soy protein isolate (SPI) is a high-quality plant protein that has been used in some food products because of its good nutritional value and functional properties.[Citation1] The main globulins in SPI are β-conglycinin (7S) and glycinin (11S).[Citation2,Citation3] The functional properties of SPI are closely associated with the composition and structure of the 7S and 11S globulins.[Citation4] There are two types of SPI products: native and commercial soy protein isolate (CSPI). Native SPI, which is highly soluble, is often used as a soy isolate reference because there is no protein denaturation during its preparation.[Citation5] On the other hand, during the industrial processing/preparation of CSPI, protein is easily denatured as a result of acid precipitation and high temperatures; consequently, CSPI products have variable solubility and denaturation degrees (low or partially denatured).[Citation5] The denaturation degree of SPI affects its solubility and functional properties.[Citation2] Additionally, the solubility of SPI is very important to the food industry when evaluating and screening other physicochemical and functional properties.[Citation6] CSPI, which has poor solubility and functional properties, is characterized by protein denaturation, aggregation, and precipitation, which are induced by heat and/or chemical treatments. In the food industry, CSPI has very limited applications.[Citation7–Citation9] Therefore, it is of utmost importance to assess appropriate chemical and/or physical methods to improve the solubility and functionalities of CSPI. To the best of the authors knowledge, few studies have focused on appropriate modification methods for CSPI.
Foaming properties (e.g., foaming capacity [FC] and foaming stability [FS]) are important functionalities of SPI. The foaming properties of native SPI are limited and unsuitable for food applications because of its quaternary and compact tertiary structure.[Citation10−Citation14] Several studies have reported that the heat-induced structural modification of SPI improves several of the SPI functionalities including its foaming properties.[Citation13,Citation15,Citation16] Sorgentini et al.[Citation4] reported that the FC of 5% (w/w) SPI increased slightly after heat treatment at 80°C for 30 min, whereas the FC and stability of 8–15% (w/w) SPI increased significantly after heat treatment at 100°C for 30 min. Researchers have reported that the effect of heat treatment under different conditions (e.g., temperature, time, protein concentration, pH, and ionic strength) on the foaming properties and solubility of SPI depends on the degree of dissociation, denaturation, and aggregation of the 7S and 11S globulins.[Citation4,Citation17,Citation18]
SPI has both soluble and insoluble fractions. Studies have reported that the insoluble fraction of the heat-treated SPI has the greatest functional properties.[Citation19] Heat treatment of SPI results in soluble and insoluble aggregates from 7S and 11S globulins, thereby affecting SPI functionalities.[Citation4,Citation20] The effect of heat-induced aggregation on SPI functionalities, including foaming properties, is not very clear. Heat treatment can be used to modify CSPI and thereby, to improve its solubility and foaming properties. In addition, the dissociation, aggregation, and foaming properties of soluble and insoluble protein fractions of CSPI during heat treatment need to be thoroughly understood.
The objective of this study was to assess the effect of heat-induced dissociation and aggregation on the foaming properties (FC and FS) of 5% (w/v) CSPI. The relationship between heat-induced protein aggregation and solubility, surface hydrophobicity, and foaming properties of CSPI were also studied.
MATERIALS AND METHODS
Materials
CSPI with a protein content of 86.6% (w/w, dry basis) was purchased from Guanghe Biotechnology Co., Ltd. (Nantong, Jiangsu, China); 1-anilino-8-naphthalene-sulfonate (ANS) was obtained from Sigma Chemical Co. (St. Louis, MO, USA). All other chemicals, which were of analytical grade, were purchased from Sinopharm Chemical Reagent Co., Ltd. (Shanghai, China). Distilled deionized water was used throughout the experiments.
Heat Treatment and Protein Solubility
CSPI (10 g) was suspended in 200 mL of distilled water and magnetically stirred at 20–90°C for 60 min. The heated suspension was cooled to 20°C and centrifuged at 12,000 g for 15 min at 4°C. The soluble protein content in the supernatant (i.e., soluble fraction) and the total protein content in the suspension were determined by the Kjeldahl method (N × 6.25).[Citation21] Protein solubility (%) was calculated as the protein content in the supernatant relative to the total protein content in the suspension.[Citation2] CSPI supernatant and precipitate (i.e., insoluble fraction) were stored separately for further assays.
Foaming Properties
Foaming properties were evaluated according to the method reported by Wang et al.[Citation22] and Adebowale, Schwarzenbolz, and Henle[Citation23] with minor modification. In this experiment, 100 mL of 5% heat-treated (w/v) CSPI suspensions and their supernatants were separately placed in a 500 mL graduated flask and homogenized (T18 basic ULTRA-TURRAX® Dispersers Homogenizer, IKA Corporation, Germany) at 17,500 rpm for 2 min. The volume of the foam was recorded at 0 min (V0) and 30 min (V30) after homogenization. FC and FS were calculated using Eqs. (1) and (2), respectively:
Surface Hydrophobicity
CSPI surface hydrophobicity was measured using the ANS fluorescence probe according to the method reported by Kato and Nakai.[Citation24] The heat-treated CSPI suspension was diluted with 0.01 mol/L phosphate buffer (pH 7.0) resulting in final CSPI suspension concentrations of 0.02, 0.04, 0.06, 0.08, and 0.1 mg/mL. Fluorescence intensity (FI) was measured with an emission wavelength of 520 nm and an excitation wavelength of 365 nm in a Hitachi 650–60 fluorescence spectrophotometer (Hitachi Ltd., Tokyo, Japan), using a slit width of 5 nm. The initial slope of FI versus protein concentration (calculated by linear regression analysis) was used as an index of protein hydrophobicity.
Electrophoresis
Sodium dodecyl sulfate-polyacrylamide gel electrophoresis (SDS-PAGE) was performed according to the procedure reported by Liu and Xiong[Citation25] with slight modifications. The stacking and resolving gels consisted of 4 and 12% acrylamide, respectively. Samples for SDS-PAGE were prepared with and without β-mercaptoethanol (5%, v/v). In this experiment, 1 mmol/L N-ethylmaleimide was added to the samples without β-mercaptoethanol to prevent the formation of disulfide compounds. To quantify the protein bands, the gel was subjected to densitometric analyses using a Molecular Analyst/PC software (Bio-Rad’s Image Analysis Systems Version 1.5). The relative percentages of CSPI subunits/polypeptides corresponding to individual bands in the gel were determined as the density area of subunits/polypeptides bands relative to the total band density area in the densitogram.
Molecular Weight (MW) Distribution
The MW distribution of CSPI suspension was determined by HPLC equipped with a gel permeation chromatographic (GPC) column (Shodex Protein KW-84 column; 8 mm I.D. × 30 cm, Shodex Co., Tokyo, Japan) and a Waters 2487 dual λ absorbance detector (Waters Co., USA). The elution solvents consisted of 50 mmol/L phosphate buffer (pH 7.0) and 0.03 mol/L sodium chloride; the flow rate was 0.8 mL/min and the column oven temperature was 30°C. Carbonic anhydrase (MW of 29 kDa), bovine serum albumin (MW of 66 kDa), alcohol dehydrogenase (MW of 150 kDa), β-amylase (MW of 200 kDa), apoferritin (MW of 443 kDa), and bovine thyroglobulin (MW of 669 kDa) were used as MW standards.
Total Amino Acid Analysis
The amino acid composition of the CSPI precipitates was determined by HPLC using the OPA derivation method.[Citation26–Citation28] The precipitates were digested with 6 mol/L HCl at 110°C for 24 h. Total amino acid analysis was performed using an Agilent HP1100 amino acid analyzer (Agilent Co., Palo Alto, CA, USA) equipped with a C18 column (4.6 mm × 125 mm) for amino acid separation. Pre-treatment with phthalic dicarboxaldehyde (OPA) yielded amino acid derivatives. The concentrations of the specific amino acids were determined from their absorption intensities, which were calibrated using amino acid standards.
Statistical Analyses
All experiments were replicated three times and triplicate sample analyses were performed for each replication to obtain the mean value. The results were expressed as mean value ± standard deviation (SD) in this study. Data were analyzed by one-way analysis of variance (ANOVA) with Tukey’s test using the Origin 7.5 statistical program (OriginLab Corporation, Northampton, MA, USA) to determine the statistical differences. Differences between treatment means were considered significant with p < 0.05.
RESULTS AND DISCUSSION
Solubility of CSPI at Different Temperatures
Solubility is one of the most important characteristics of food proteins because it affects other functionalities such as foaming and emulsifying properties.[Citation18] The solubility of 5% (w/v) CSPI subjected to different temperatures is shown in . The solubility of the 5% CSPI suspension at 20°C was approximately 72.3% and gradually increased from 20 to 90°C. The solubility of CSPI (the temperature was 20°C) increased to 91.8% when heat-treated at 90°C for 1 h, suggesting that CSPI solubility can be further improved by heat treatment.
FIGURE 1 Characteristics of CSPI subjected to different temperatures (20–90°C): (a) solubility of 5% (w/v) CSPI suspension; (b) surface hydrophobicity of 5% (w/v) CSPI suspension; (c) foaming properties of 5% (w/v) CSPI suspension; (d) foaming properties of CSPI supernatant obtained from 5% (w/v) CSPI suspension. Different letters (a–e) labeled on the same curve indicate significant difference (p < 0.05).
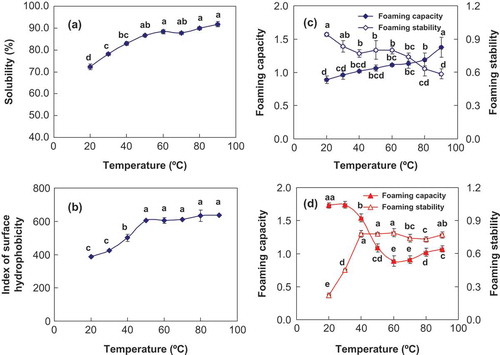
As temperatures reached 80–90°C, which is the denaturation temperature of 7S and 11S globulins, the solubility of native SPI decreased as a result of polypeptide chain unfolding and exposure of hydrophobic groups buried inside the proteins. However, in this study, the solubility of CSPI, which is a low or partially denatured protein, increased with heat treatment probably as a result of the dissociation of insoluble aggregates and the formation of soluble aggregates.[Citation2,Citation29]
Surface Hydrophobicity of the CSPI Suspension at Different Temperatures
The surface hydrophobicity of 5% CSPI suspension subjected to different temperatures was correlated with its change in solubility (). As temperature increased, the globular proteins unfolded and the hydrophobic groups were exposed, thereby increasing CSPI surface hydrophobicity. At 20–50°C, surface hydrophobicity of the CSPI suspension increased significantly (p < 0.05) with increasing temperatures, whereas it increased slightly (p > 0.05) at 50–90°C. According to Berli, Deiber, and Añón,[Citation30] when temperatures are >70°C, denatured globulins aggregate and form soluble, large micelles, which could explain the slight change in surface hydrophobicity at 50–90°C.
Foaming Properties of the CSPI Supernatant and CSPI Suspension
FC and FS of the 5% (w/v) CSPI suspension and its supernatant are shown in and . The foaming properties of the CSPI supernatant had different tendencies than those of the CSPI suspension subjected to different heat treatments. When temperature was <50°C, FC of the CSPI supernatant was higher than that of the CSPI suspension, which decreased rapidly (p < 0.05) with increasing temperatures (). FS of the 5% CSPI suspension decreased gradually at 20–90°C (), whereas FS of the CSPI supernatant increased sharply (p < 0.05) at <40°C and tended to be stable at 40–90°C.
The results obtained indicate that FC and FS of CSPI are related to the soluble protein content of the CSPI suspension and its conformation in aqueous solution. FC requires a rapid adsorption of protein at the air-water interface and rapid conformational changes and rearrangements at the interface. FS requires the presence of a thick, elastic, cohesive, continuous, and air-permeable protein film around each gas bubble.[Citation16] As shown in and , when temperature was <50°C, FC of the CSPI supernatant was higher than that of the CSPI suspension, which indicates that the insoluble protein fraction of the 5% CSPI suspension might have impaired FC of CSPI suspension. At temperatures >50°C, FC of the CSPI supernatant was lower than that of the CSPI suspension. The insoluble fraction of CSPI suspension decreased gradually and the soluble protein content of the 5% CSPI suspension increased, which indicated that a high proportion of CSPI soluble fractions increased FC of CSPI suspension. However, when temperatures were 80–90°C, FS of the CSPI supernatant was higher than that of the CSPI suspension, suggesting that a high proportion of CSPI soluble fractions did not increase FS of CSPI suspension.
SDS-PAGE of the CSPI Supernatant and CSPI Suspension
shows the SDS-PAGE patterns of the 5% CSPI suspension and CSPI supernatant subjected to different temperatures in the absence () and presence of 5% β-mercaptoethanol (). β-mercaptoethanol, which is a compound that breaks disulfide bonds, is used to assess the role of S-S linkages in the formation of protein aggregates.[Citation30,Citation31] The densitometric analysis of the main protein bands in SDS-PAGE are shown in . As shown in and , the protein profiles of the CSPI suspension and supernatant including β-conglycinin subunits (α′, α, and β) and glycinin polypeptides (A and B) were not different at 20–90°C under non-reducing conditions (i.e., without β-mercaptoethanol). As shown in and , high MW bands corresponding to protein aggregates were obtained for the CSPI suspension and CSPI supernatant; however, the percentage of aggregates in the CSPI supernatant (soluble fraction) was slightly lower than that in CSPI suspension, which indicated the presence of insoluble protein aggregates in CSPI. The percentages of aggregates of high MW in the CSPI suspension and CSPI supernatant increased gradually as temperatures increased from 20 to 80°C and decreased significantly (p < 0.05) when temperatures
TABLE 1 Relative compositions (%) of 5% CSPI suspensions and CSPI supernatants subjected to different temperatures
Under reducing conditions (i.e., with β-mercaptoethanol), the original bands of high MW (>116 KD) of the CSPI suspension and CSPI supernatant became fainter; the A and B-11S polypeptides appeared as two intense bands in the SDS-PAGE patterns of all samples (). The percentage of aggregates in the CSPI suspension and CSPI supernatant decreased, whereas the percentage of the A and B-11S polypeptides increased following the dissociation of disulfide bonds (). These results revealed that high MW polymers and aggregates of CSPI were formed mainly from A and B-11S polypeptides via disulfide bonds.
MW Distribution of the CSPI Supernatant
shows the MW distribution of the CSPI supernatant obtained from the 5% CSPI suspension heat-treated at 20–90°C. As shown in , the chromatographic patterns of the CSPI supernatant were similar. According to the MW standard curve, the chromatographic patterns of the CSPI supernatant consisted of four major fractions: (A), which corresponded to protein aggregates (MW > 400 kDa; retention time of 5.2–9.0 min);[Citation32] (B), which corresponded to 11S and 7S of SPI (MW of 100–400 KDa; retention time of 9.0–10.2 min); (C), which corresponded to 7S and 11S subunits (MW of 10–100 KDa; retention time of 10.2–12.5 min); and (D), which corresponded to polypeptides of small MW (MW < 10 KDa; retention time of more than 12.5 min). The relative areas of these four protein fractions were different under different temperatures (). At 20–90°C, the relative area of fraction A (MW > 400 kDa) increased significantly (from 47.9 to 66.8%) and then decreased slightly at temperatures >80°C. The relative area of fractions B, C, and D, which had lower MWs, decreased gradually from 20 to 80°C and increased from 80 to 90°C, which indicated that (1) CSPI polypeptides and subunits formed soluble protein aggregates and polymers of large MWs after heat treatment and (2) some CSPI aggregates dissociated into low MW polypeptides and subunits when temperatures were >90°C. The MW distribution of SPI subjected to different heat treatments was in accordance with its SDS-PAGE patterns ( and ).
FIGURE 3 Molecular weight distribution of CSPI supernatant obtained from 5% (w/v) CSPI suspension subjected to different temperatures. (a) High performance size exclusion chromatographic profiles of CSPI supernatants (A: >400 kDa; B: 100–400 kDa; C: 10–100 kDa; D: <10 kDa). The equation of the standard curve was y = – 0.5009x + 10.086; R2 = 0.9185. (b) Relative areas (%) of the four protein fractions (A, B, C, and D) of CSPI supernatants.
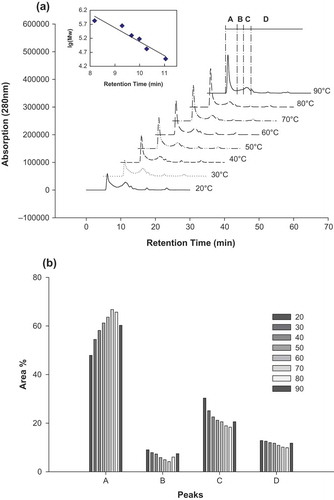
The results revealed that there were good correlations between soluble aggregate contents and solubility, surface hydrophobicity, and FC of the 5% CSPI suspension after heat treatment ( and ). FC, solubility and surface hydrophobicity of CSPI suspension increased following the increase of soluble aggregates formed. However, the formation of soluble aggregates induced by heat treatment was negatively correlated with FS of CSPI, due to a gradual reduction in large MW insoluble aggregates, which are involved in FS.
Amino Acid Composition of the CSPI Precipitate
shows the amino acid composition of the precipitate (i.e., insoluble fraction) obtained from the 5% CSPI suspension subjected to different temperatures. Of a total of 17 amino acids, Asp, Glu, Arg, Leu, and Pro were the most abundant. With the exception of Pro and Cys, most of the amino acids contents changed no significantly (p > 0.05) during heat treatment. At 40–60°C, the content of Pro and Cys in the CSPI precipitate was the highest and then decreased slightly with increasing temperatures (60–90°C). The foaming properties of the CSPI supernatant had similar tendencies (); 40–60°C was the turning point for FC and FS of the CSPI supernatant. FC of the CSPI supernatant decreased and FS increased slightly at 40–60°C.
TABLE 2 The amino acid composition of CSPI precipitates obtained from 5% CSPI suspensions subjected to different temperatures
FS of the CSPI suspension decreased gradually at 60–90°C (), which was in accordance with the reduction in Pro and Cys content in the CSPI precipitate. It was reported that both Pro and Cys have significant effects on stabilizing the secondary structure of proteins, owing to the N-atom in the pyrrolidine ring of Pro and S-S bonds of Cys which can reduce the flexibility of peptide backbone, enhance the hydrophobic interaction, and make the protein structure more stable.[Citation33] Therefore, a high content of Pro and Cys in CSPI precipitates probably could stabilize CSPI foams.
CONCLUSIONS
The results of this study revealed that heat treatment at 20–90°C of a 5% CSPI suspension increased its solubility, hydrophobicity, and FC but decreased its FS. Foaming properties of CSPI are associated with the amount of the soluble CSPI fraction; the CSPI soluble fraction improved FC but did not affect FS. The SDS-PAGE patterns and MW distribution of CSPI revealed that large MW aggregates and polymers were formed mainly from the A and B-11S polypeptides of CSPI via disulfide bonds. Additionally, some aggregates also dissociated into polypeptides and subunits of low MW during heat treatment. The insoluble CSPI fraction (i.e., precipitates) had a high content of Pro and Cys, which probably could favor FS. These heat-induced changes in CSPI could improve its foaming properties, which would benefit the further application of CSPI products in the food industry.
FUNDING
This work was financially supported by the Natural Science Foundation of China (No. 31000751, No. 20976071), the Doctoral Funds from the Ministry of Education (No. 20100093120005), and the Fundamental Research Funds for the Central Universities (No. JUSRP21108).
Additional information
Funding
REFERENCES
- Martínez, K.D.; Ganesan, V.; Pilosof, A.M.R.; Harte, F.M. Effect of dynamic high-pressure treatment on the interfacial and foaming properties of soy protein isolate-hydroxypropylmethylcelluloses systems. Food Hydrocolloid 2011, 25, 1640–1645.
- Arrese, E.L.; Sorgentini, D.A.; Wagner, J.R.; Añón, M.C. Electrophoretic, solubility, and functional properties of commercial soy protein isolates. Journal of Agricultural and Food Chemistry 1991, 39, 1029–1032.
- Ortiz, S.E.M.; Wagner, J.R. Hydrolysates of native and modified soy protein isolates: Structural characteristics, solubility, and foaming properties. Food Research International 2002, 35, 511–518.
- Sorgentini, D.A.; Wagner, J.R.; Añón, M.C. Effects of thermal treatment of soy protein isolate on the characteristics and structure-function relationship of soluble and insoluble fractions. Journal of Agricultural and Food Chemistry 1995, 43, 2471–2479.
- Wagner, J.R.; Sorgentini, D.A.; Añón, M.C. Relation between solubility and surface hydrophobicity as an indicator of modifications during preparation processes of commercial and laboratory-prepared soy protein isolates. Journal of Agricultural and Food Chemistry 2000, 48, 3159–3165.
- Lee, K.H.; Ryu, H.S.; Rhee, K.C. Protein solubility characteristics of commercial soy protein products. Journal of the American Oil Chemists Society 2003, 80, 84–90.
- Tang, C.H.; Wang, X.Y.; Yang, X.Q.; Li, L. Formation of soluble aggregates from insoluble commercial soy protein isolate by means of ultrasonic treatment and their gelling properties. Journal of Food Engineering 2009, 92, 432–437.
- Chronakis, I.S.; Kasapisis, S.; Richardson, R.K.; Doxastakis, G. Characteristation of a commercial soy isolate by physical techniques. Journal of Texture Studies 1995, 26, 371–389.
- Wagner, J.R.; Sorgentini, D.A.; Añón, M.C. Effect of physical and chemical factors on rheological behavior of commercial soy protein isolates: Protein concentration, water imbibing capacity, salt addition, and thermal treatment. Journal of Agricultural and Food Chemistry 1992, 40, 1930–1937.
- Kinsella, J.E. Functional properties of soy proteins. Journal of the American Oil Chemists Society 1979, 56, 242–258.
- Utsumi, S.; Matsumura, Y.; Mori, T. Structure-Function Relationships of Soy Protein. In Food Proteins and Their Application; Damodaran, S., Paraf, A., Eds.; Marcel Dekker: New York, 1997; 257–291.
- Yu, M.A.; Damodaran, S. Kinetics of destabilization of soy proteins foams. Journal of Agricultural and Food Chemistry 1991, 39, 1563–1567.
- Martínez, K.D.; Sánchez, C.C.; Patino, J.M.R.; Pilosof, A.M.R. Interfacial and foaming properties of soy protein and their hydrolysates. Food Hydrocolloid 2009, 23, 2149–2157.
- Martínez, K.D.; Farías, M.E.; Pilosof, A.M.R. Effects of soy protein hydrolysis and polysaccharides addition on foaming properties studied by cluster analysis. Food Hydrocolloid 2011, 25, 1667–1676.
- Yamauchi, F.; Yamagishi, T.; Iwabuchi, S. Molecular understanding of heat-induced phenomena of soybean protein. Food Review International 1991, 7, 283–322.
- Were, L.; Hettiarachchy, N.S.; Kalapathy, U. Modified soy proteins with improved foaming and water hydration properties. Journal of Food Science 1997, 62, 821–824.
- Martin, A.H.; Grolle, K.; Bos, M.A.; Stuart, M.A.C.; Vliet, T. Network forming properties of various proteins adsorbed at the air/water interface in relation to foam stability. Journal of Colloid and Interface Science 2002, 254, 175–183.
- Ruíz-Henestrosa, V.P.; Sánchez, C.C.; Escobar, M.M.Y.; Jiménez, J.J.P.; Rodríguez, F.M.; Patino, J.M.R. Interfacial and foaming characteristics of soy globulins as a function of pH and ionic strength. Colloids and Surfaces A. Physicochemical and Engineering Aspects 2007, 309, 202–215.
- Sorgentini, D.A.; Wagner, J.R.; Arrese, E.L.; Añón, M.C. Water imbibing capacity of soy protein isolates: Influence of protein denaturation. Journal of Agricultural and Food Chemistry 1991, 39, 1386–1391.
- Utsumi, S.; Damodaran, S.; Kinsella, J.E. Heat-induced interactions between soybean proteins: Preferential association of 11s-basic subunits and β-subunit of 7S. Journal of Agricultural and Food Chemistry 1984, 32, 1406–1412.
- AOAC. Official methods of analysis of AOAC International, 14th Ed; Association of Official Analytical Chemists: Arlington, VA, 1984.
- Wang, B.; Jiang, Y.L.; Liu, J.; Zhang, W.Q.; Pan, S.K.; Mao, Y. Physicochemical and functional properties of tea protein. International Journal of Food Properties 2014, 17, 2275–2283.
- Adebowale, Y.A.; Schwarzenbolz, U.; Henle, T. Protein isolates from bambara groundnut (voandzeia subterranean L.): Chemical characterization and functional properties. International Journal of Food Properties 2011, 14, 758–775.
- Kato, A.; Nakai, S. Hydrophobicity determined by a fluorescence probe methods and its correlation with surface properties of proteins. Biochimica et Biophysica Acta (BBA)- Protein Structure 1980, 624, 13–20.
- Liu, G.; Xiong, Y.L. Electrophoretic pattern, thermal denaturation, and in vitro digestibility of oxidized myosin. Journal of Agricultural and Food Chemistry 2000, 48, 624–630.
- Zhu, L.J.; Chen, J.; Tang, X.Y.; Xiong, Y.L. Reducing, radical scavenging, and chelation properties of in vitro digests of Alcalasetreated zein hydrolysate. Journal of Agricultural and Food Chemistry 2008, 56, 2714–2721.
- Amadou, I.; Le, G.W.; Shi, Y.H. Evaluation of antimicrobial, antioxidant activities, and nutritional values of fermented foxtail millet extracts by lactobacillus paracasei Fn032. International Journal of Food Properties 2013, 16, 1179–1190.
- Li, F.; Wu, X.Y.; Zhao, T.; Li, F.; Zhao, J.L.; Yang, L.Q. Extraction, physicochemical, and functional properties of proteins from milk thistle silybum marianum L. gaernt seeds. International Journal of Food Properties 2013, 16, 1750–1763.
- Petruccelli, S.; Añón, M.C. Thermal aggregation of soy protein isolates. Journal of Agricultural and Food Chemistry 1995, 43, 3035–3041.
- Berli, C.L.A.; Deiber, J.A.; Añón, M.C. Heat-induced phenomena in soy protein suspensions rheometric data and theoretical interpretation. Journal of Agricultural and Food Chemistry 1999, 47, 893–900.
- Jiang, J.; Chen, J.; Xiong, Y.L. Structural and emulsifying properties of soy protein isolate subjected to acid and alkaline pH shifting processes. Journal of Agricultural and Food Chemistry 2009, 57, 7576–7583.
- Li, X.H.; Li, Y.; Hua, Y.F.; Qiu, A.Y.; Yang, C.; Cui, S. Effect of concentration, ionic strength, and freeze-drying on the heat-induced aggregation of soy proteins. Food Chemistry 2007, 104, 1410–1417.
- Zhu, G.P.; Teng, M.K.; Wang, Y.Z. Contribution of prolines to protein thermostability. Progress in Biotechnology 2000, 20, 48–51.