Abstract
Gelatin-calcium carbonate films incorporated with different natural antioxidants (sodium ascorbate, d-sodium erythorbate, and tea polyphenols) were investigated in this study. The results indicated that all of the films with antioxidants displayed promoted tensile strength and higher thickness than that of the control (p < 0.05), and most films had higher values of elongation at break. Furthermore, the addition of antioxidants favored the reduction of water vapor permeability and water solubility in comparison with the control (p < 0.05). The alternations of light transmission could vary depending on the type and concentration of antioxidants. In addition, the surface properties of films were characterized by atomic force microscopy, indicating that antioxidants caused the formation of gelatin-calcium carbonate aggregates and clusters. Moreover, Fourier transform infrared spectroscopy spectra demonstrated that antioxidants facilitated the conformational changes of the secondary structure and functional groups of gelatin in films. Gelatin-calcium carbonate films containing different natural antioxidants exhibited improved 2, 2-diphenyl-1-picryl hydrazyl radical scavenging capacities, compared to the control (p < 0.05).
INTRODUCTION
Sustainable edible and/or biodegradable films derived from food resources have evoked much interest due to the environmental concerns caused by the vast majority of disposable petroleum-based packaging materials,[Citation1,Citation2] consisting of polysaccharides,[Citation3−Citation5] proteins,[Citation6−Citation10] and lipids.[Citation11−Citation14] Gelatin is a sort of flavorless and colorless mixture of polypeptide and protein obtained from different animal by-products, possessing excellent film-forming features, emulsifying properties, and high water-binding capacity. Furthermore, gelatin is cost-effective, highly available, and biodegradable. These strengths enable its wide applications in the food, pharmaceutical, and cosmetic industries as a stabilizer, thickener, or texturizer.[Citation15,Citation16] In particular, gelatin has been utilized in the preparation of gelatin-based composite films by blending with chitosan,[Citation17] whey protein and soy protein,[Citation18] nanotube,[Citation19] clay nanocomposite,[Citation20] and montmorillonite.[Citation21]
Calcium carbonate, a stable and safe natural material, is mainly achieved from shells of marine organisms, rocks, and eggshells, acting as the physical barrier for hindering the harmful organisms and microbiology from the living environment.[Citation22−Citation24] Conventionally, it is used as biodegradable fillers or supporters from natural origins.[Citation25] In addition, calcium carbonate is generally considered as a dietary supplement since calcium is crucial for heart, nervous system, muscles, and healthy bones.[Citation26] Recently, calcium carbonate has been applied to fabricate the microcapsules,[Citation22] nanotablets,[Citation27] and composite films.[Citation28,Citation29]
Oxidation and microbial spoilage are fundamentally responsible for the deterioration of quality in foods. Antioxidants are greatly associated with the minimization of oxidative deterioration by preventing the radical chain reactions of oxidation, and thus, prolonging the shelf life and remaining the nutritional properties, along with sensory quality. Synthetic antioxidants such as butylated hydroxytoluene (BHT) or butylated hydroxyanisole (BHA) are conventionally employed to inhibit oxidative process in foods. However, the employment of synthetic antioxidants leads to safety concerns.[Citation30] Natural-based antioxidants are viewed as ideal alternatives to chemical-based preservatives, and their applications in the food industries satisfy consumer demands for healthy diet without compromising on the quality of foods.[Citation31,Citation32] It is well documented that sodium ascorbate (vitamin C, obtained from fruits and vegetables), d-sodium erythorbate (sodium salt of erythorbic acid, achieved from microbial fermentation of beets, sugar cane, and corn) are antioxidants commonly used in meats, seafood, fruit juices, and beverages for decelerating the oxidation process.[Citation33] Tea polyphenols are also potent food-functional antioxidative polyphenols widely distributed in herbs and tea, respectively.[Citation34] Nevertheless, gelatin-mineral films containing natural antioxidants are rarely reported in literature. Therefore, in this study, several plant-based antioxidants (sodium ascorbate, d-sodium erythorbate, and tea polyphenols) were incorporated into the gelatin-calcium carbonate composite films. The effects of plant-based antioxidants on the physiochemical properties of films, such as thickness, color, light transmittance, tensile strength (TS), water vapor permeability (WVP), gel strength, elongation at break (EAB), water solubility (WS) were investigated. Moreover, roughness parameters and surface morphology of films containing plant-based antioxidants were studied by atomic force microscopy (AFM). Furthermore, the antioxidant capacities of films combining with different plant-based antioxidants were also evaluated and compared in this manuscript.
MATERIAL AND METHODS
Chemicals
The commercial type A bovine-hide gelatin (Bloom 220) was purchased from Qingdao Tianxin food additives company. CaCO3 (analytical grade), glycerin (analytical grade), Ascorbic acid (analytical grade), D-sodium erythorbate (analytical grade), tea polyphenols (analytical grade), and 2, 2-diphenyl-1-picryl hydrazyl (DPPH; analytical grade) were obtained from Sigma-aldrich (Shanghai, China). All commercial chemicals were of analytical grade and used without further purification.
Films Preparation
The films were prepared using the casting method. Initially, gelatin was gently mixed with distilled water at a ratio of 5% (w/v) under mechanical stirring until it was completely dissolved. Afterward, CaCO3 with 10.0% of gelatin (w/w) was added into the solution in the presence of glycerol with 40% of gelatin (w/w) as a plasticizer in all films. Meanwhile, antioxidant was incorporated into the stock solution for the preparation of the film at a given concentration, containing sodium ascorbate (0.1 and 0.4 mg/mL), D-sodium erythorbate (0.1 and 0.4 mg/mL), and tea polyphenols (0.1 and 0.4 mg/mL). All mixtures were stirred at 45°C for 30 min to obtain a homogeneous solution followed by placing a ventilated oven (25 ± 0.5°C) for 10 h. Before performing determinations, the dried films were conditioned in desiccator over a saturated solution of MgNO3•6H2O (50% relative humidity) at 25 ± 0.5°C.
Characterization
Film thickness
Film thickness was determined using a hand-held micrometer (Mitutoyo No. 293-766, Tokyo, Japan) with a precision of 0.001 mm. Measurements were carried out at ten different film locations and the mean thickness value was used to calculate the permeability and mechanical properties of the films.
Mechanical properties
A texture analyzer (Stable Micro Systems Ltd, UK) was employed to measure the mechanical properties, following the published protocol[Citation35] with minor modifications. The TSs and elongations to break of the films were measured. The films were cut into rectangular test strips with a length of 70 mm and a width of 25 mm, and the cross-head speed was set at 20 mm/s. At least two replications were used to determine the ultimate TS, elongation to break. TS was calculated by dividing the maximum load at break by the area of cross-section and expressed in MPa. EAB (E, %) was expressed as:
WVP
The WVP of the films was measured using the method reported by.[Citation36] The gelatin film was cut to 2 cm in diameter, and the film was used to seal a testing cup containing anhydrous calcium sulfate. The films were allowed to equilibrate for 1 h before the cells were initially weighed. Thereafter, the weight of the cup was measured intermittently at intervals of 24 h, up to 96 h. WVP of the films was calculated as follows:
WS
The WS of the film was evaluated following the method in literature with slight modification.[Citation37] The film samples were cut to a square piece of 20 mm × 40 mm and accurately weighed to give the dried film (W0). The films placed into test beakers with 300 mL distilled water. The samples were immersed for 24 h at 25°C. After that period, the remained pieces of film were then filtered and dried in a hot air oven at 105°C until a final constant weight was obtained (W1). The percentage of solubility of the film was calculated according to the equation:
Color
Color of film samples was determined using an Automatic color measurement colorimeter (TCP2, Ao Yi Ke photoelectric instrument company, China). Color of the film was expressed as L* (lightness/brightness), a* (redness/greenness), and b* (yellowness/blueness) values. Total difference in color (ΔE*) was calculated according to the following equation:
Light Transmission and Transparency
The barrier properties of gelatin films against ultraviolet (UV) and visible light were measured at selected wavelengths between 200 and 800 nm, using a UV-visible recording spectrophotometer according to a published method.[Citation38] The transparency value of the film was calculated by the following equation:
AFM
Before testing, samples were preconditioned at 50% RH and at room temperature for at least 48 h. The AFM was carried out by an Autoprobe CP Research instrument (Veeco Instruments, NY, USA) in contact mode with a 20 μm × 20 μm scan size and a 5 μm vertical range. In this section, silicon probes (Veeco probes, NY, USA) were used, with a spring constant of 0.5 nm−1. The data were transformed into a 3D image. Measurements were taken from several areas of the film surface (20 μm × 20 μm). The following two statistical parameters related with sample roughness were calculated: average roughness (Ra: average of the absolute value of the height deviations from a mean surface), root-mean-square roughness (Rq: root-mean-square average of height deviations taken from the mean data plane). A minimum of three replicates were considered to obtain these parameters.
Fourier Transform Infrared Spectroscopy (FTIR)
FTIR spectra were generated from pellets of films examined with a Thermo Nicolet Avatar 370 Fourier transform infrared spectrometer equipped with a DTGS KBr detector. The films were subjected to 16 scans per sample at 1.9 cm−1 resolution from 4000 to 600 cm−1. Data processing was conducted with the Omnic software package where peak maxima were determined manually after examination of absorbance values.
DPPH Free Radical Scavenging Activity
The antioxidant activity of the films was evaluated by assaying the scavenging of free radical of DPPH following the method of various authors[Citation32,Citation38−Citation40] with slight modification. DPPH assay solution was prepared by mixing 6 mL of the film stock solution with 2 mL of methanolic solution of DPPH (0.7 mm/L). After shaken in an oscillator for 1 min, the mixture was incubated in the dark at room temperature for 30 min. The UV absorbance of the DPPH assay solution at 517 nm was measured. DPPH scavenging activity was calculated as follows:
Statistical Analysis
All determinations were performed in three replicates. Data are expressed as mean ± SD. Analysis of variance (ANOVA) was used for analysis of the test results (least significant difference) at the significance level of p-value < 0.05 using the SAS software (SAS Institute Inc., Cary, NC, USA).
RESULTS AND DISCUSSION
Physical Properties of Gelatin-Calcium Carbonate Films Containing Different Plant-Based Antioxidants
Thickness
All films containing natural antioxidants exhibited higher thickness than that of the control, regardless of the type and concentration of antioxidants (p < 0.05) in . In particular, the higher concentrations of natural antioxidants favored the higher thicknesses of films than that of the lower concentrations of natural antioxidants (p < 0.05). It was postulated that the interaction between natural antioxidants and peptide chains of gelatin along with calcium carbonate could affect the ordered alignment of the structure of the films. Consequently, the compact and convex network was formed, resulting in the increased thickness. The identical trend was observed for fish gelatin films incorporated with leaf essential oils (lemongrass, basil, citronella, and kaffir lime).[Citation38]
TABLE 1 Physical properties of gelatin-calcium carbonate films containing different plant-based antioxidants
TS and EAB
TS and EAB are central physical properties of the gelatin-related products since it is an indicator for the specific application of products.[Citation41] The TS is defined as the maximum strength of a film for the resistance of tensile stress and percent elongation represents the stretch ability of a film. TS and EAB of gelatin-calcium carbonate films containing various natural antioxidants are depicted in . Compared to the control, natural antioxidants significantly improved TS of the films (p < 0.05) regardless of the concentration (p > 0.05). For gelatin-based films, the weak bond including hydrogen bond and hydrophobic interaction mainly contribute to the stabilization of the structures of films.[Citation42] The presences of natural antioxidants may heighten the intermolecular interactions of polypeptide chains. It was suggested that the hydrophobic groups of polyphenols could interact with gelatin by hydrophobic interaction, while hydroxyl group of polyphenol was able to interact with gelatin via hydrogen bonds, thereby resulting in the increase of TS.[Citation38] It was observed that TS of the pure gelatin film was reduced when some natural antioxidants were introduced into the film. This result might be attributed to the different types and concentrations of natural antioxidants. Moreover, the occurrence of calcium carbonate substantially improved the TS of films,[Citation43] probably contributing to the higher TS than that of the pure gelatin film (15.5 ± 3.9).[Citation44] Compared to the control, EAB was significantly enhanced (p < 0.05) after adding natural antioxidants, other than sodium ascorbate with a lower concentration. EAB values are primarily influenced by plasticizers, which penetrate into biopolymer matrix, thereby promoting the volume of spaces between polypeptide chains by the hydrophobic interaction and leading to the reduction of film brittleness and the increase of the mobility of molecules.[Citation9] Given that calcium carbonate has insignificant impact on EAB, the different interactions between natural antioxidants and plasticizers caused different extents of the changes in EAB.[Citation43] The same trends for TS and EAB of chitosan film incorporated with green tea extract.[Citation45]
WVP and WS
WVP is a critical indicator of the water-barrier properties of the food-packaging material. In general, the moisture transfer between foods and the storage environment is suggested to be minimized. Hence, WVP should be controlled at a low level. As illustrated in , the WVPs of gelatin films were significantly decreased when natural antioxidants were added into the films (p < 0.05). This could be attributed to the fact that natural antioxidants might strengthen the cross-linking of gelatin and reduce the free volume of the polymeric matrix, thus increasing the tortuous paths within the composite films. These results slowed the diffusion rate of water molecules through the films, which leaded to the lower WVP of the films.[Citation46] Moreover, calcium carbonate acted as a water barrier, resulting in the low WVP of gelatin-calcium carbonate films.[Citation47] It was observed that the WVP of gelatin films were significantly decreased when chitosan was added into the gelatin films (p < 0.05).[Citation48] Furthermore, the additions of essential oils could intensify the intensity of hydrophobicity of films, thereby causing the reduction of the water vapor migration through the film.[Citation49]
The WS of gelatin-calcium carbonate films significantly reduced (p < 0.05) with antioxidants, rather than the low concentration of sodium ascorbate, as demonstrated in . Gelatin films with a higher amount of hydrophilic amino acids enabled water uptake from the external environment.[Citation50] As stated previously, natural antioxidants rendered the entanglement of gelatin polypeptide chains within the film matrix by electrostatic forces, hydrogen bonding, etc. Plus, calcium carbonate generated remarkable physical shield for water molecular. Therefore, it hindered the ability of gelatin to interact with water molecules, which was mainly responsible for weakening the WS in the matrix of films. The WS of the chitosan film has been significantly improved after adding carvacrol or grape seed extract into the films.[Citation51]
Color
The effects of natural antioxidants with different concentrations on film color (L*, a*, b*, ΔE) are summarized in . Compared to the control, the incorporations of natural antioxidants (sodium ascorbate and d-sodium erythorbate) into the films have no significant impact on L* (lightness/darkness) between groups (p > 0.05) regardless of the concentration, other than tea polyphenols. The films including tea polyphenols tended to be darker than that of other groups (p < 0.05). a* (redness/greenness) values of the surfaces of films have been significantly modified by the additions of sodium ascorbate (higher concentration), d-sodium erythorbate, and tea polyphenols (p < 0.05). In particular, the presence of natural antioxidant (sodium ascorbate, d-sodium erythorbate, and tea polyphenols) significantly resulted in an increase in b* (yellowness/blueness) values of the films, indicating the tendency toward the yellowish color (p < 0.05), except for the film containing a low concentration of sodium ascorbate. Furthermore, the occurrences of natural antioxidants could significantly influence the values of total differences in colors (p < 0.05) of the films with the exception of the group encompassing a lower concentration of sodium ascorbate. In literature, it was found that the higher concentration of green tea extract could cause an increase in the intensity of the yellowness of the chitosan films.[Citation52] Wheat starch-chitosan films containing α-tocopherol exhibited the promoted intense of yellow color.[Citation53]
TABLE 2 The color properties of gelatin-calcium carbonate films containing different plant-based antioxidants
Light Transmittance and Transparency
Light transmittance and transparency of the film is associated with the capability of preventing foods from the of UV radiation.[Citation54] The results of spectroscopic scanning of all samples at wavelengths ranging from 200 to 800 nm are shown in . The changes of light transmission were different depending on the type and concentration of antioxidants. The incorporation of sodium ascorbate, and d-sodium (the lower concentration) into the films facilitated the higher light transmission. In contrast, the presence of tea polyphenols and d-sodium erythorbate (the higher concentration) slightly lowered the light transmission. Compared to the pure gelatin film in literature,[Citation54] the gelatin-calcium carbonate has substantially lower light transmission because calcium carbonate displayed UV-shielding properties.[Citation55] Zhang et al. reported that the gelatin film with gingko leaf extract had the lowest value of light transmission in UV light when comparing with films containing green tea extract, grape seed extract, and ginger extract.[Citation54] In addition, the differences in light transmission were also varied with types of essential oils and surfactants used in fish gelation.[Citation38]
TABLE 3 Light transmittance and transparency values of gelatin-calcium carbonate films containing different plant-based antioxidants
AFM
To gain a more comprehensively visible understanding of the surface structures of the samples, gelatin films comprising natural antioxidants were also investigated using AFM. represents the surface morphologies for the films, corresponding to results of roughness parameters—the average roughness (Ra) and root-mean-square roughness (Rq) in . The pure gelatin-calcium carbonate film with a smooth surface () showed Ra and Rq values of 20.90 and 27.03, respectively, with a height of 643 nm. However, the surfaces of the films became rougher than the control when adding natural antioxidants into the films on the basis of the AFM images (b–g). Antioxidants induced the increases of Ra and Rq values in . The heights of the gelatin-calcium carbonate films were listed: sodium ascorbate (low concentration: 1.0 μm; high concentration: 1.6 μm), d-sodium erythorbate (low concentration: 799 nm; high concentration 1.5 μm), and tea polyphenols (low concentration: 1.1 μm; high concentration: 1.9 μm). The maximal value was achieved in the occurrence of tea polyphenols, which is in agreement with the results of Ra and Rq. It appeared that antioxidants enabled gelatin molecules and calcium carbonate to assemble into gelatin-calcium carbonate aggregates and clusters presumably due to the formation of bundles of intermolecular triple helices instead of individual helical junction zones.[Citation15] The parallel trend was observed that addition of pectin into gelatin films resulted in the formation of gelatin clusters with heterogeneous structures through ionic interactions.[Citation56] Likewise, green tea extract induced the generation of compact-structure gelatin films compared with the pure gelatin film due to increased amount of protein-polyphenol interactions.[Citation57]
TABLE 4 Roughness of gelatin-calcium carbonate films containing different plant-based antioxidants
FTIR
FTIR spectra of gelatin-calcium carbonate films incorporated with antioxidants at low and high concentrations were depicted in –. The spectra of all films exhibited the major bands in amide region. Gelatin-calcium carbonate films displayed the amide I band, indicating C=O stretching/hydrogen bonding coupled with COO, at the wavenumbers of 1600–1700 cm−1 for all of the samples, which are consistent with the gelatin films from the skin of fish.[Citation37,Citation49,Citation57] In particular, slight decreases in the intensity of absorption bands detected in the presence of antioxidants regardless of the type. Amide II representing the bending vibrations of N-H groups and stretching vibrations of C-N groups is always shown at 1560–1500 cm−1.[Citation49,Citation58] In this study, it appeared that some vibrations in absorption amplitude at 1560–1500 cm−1 were observed for the gelatin-calcium carbonate films when adding antioxidants into the films. The similar conclusion was achieved from gelatin films containing other antioxidants, for instance BHT and green tea extract.[Citation57] Amide-III, illustrating the vibrations of C-N and N-H groups of bound amide or vibrations of CH2 groups of glycine in gelatin films was found at the wavenumbers of 1200–1250 cm−1.[Citation59] The bands located at the wavenumber of 1030–1035 cm−1 were recorded in all film samples, corresponding to the OH group of glycerol serving as a plasticizer.[Citation60]
FIGURE 2 FTIR spectra of gelatin-calcium carbonate films containing different plant-based antioxidants with the low concentration group (0.1 mg/mL) and the high concentration group (0.4 mg/mL): (a) sodium ascorbate; (b) d-sodium erythorbate; (c) tea polyphenols.
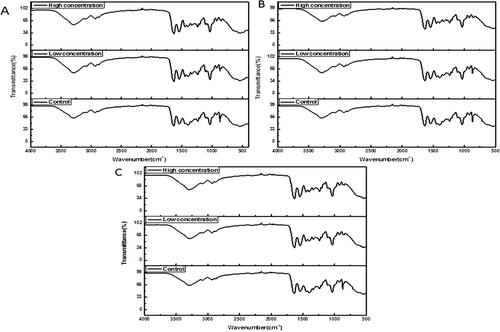
Moreover, amide A peaks at 3280–3290 cm−1, reflected NH-stretching coupled with hydrogen bonding.[Citation37] The wavenumber of amide-A peak shifted from 3289.98 cm−1 (the control) to 3283.75 cm−1 for the film containing the higher concentration of antioxidant irrespective of the type. The amide B bands, corresponding to the asymmetric stretching vibration of =C-H and NH3+, were observed at 3095 cm−1, for all the samples, without noticeable changes in amplitude with the type and the concentration of antioxidant.
summarized the effects of antioxidants on the secondary structures of gelatin-calcium carbonate films. Compared to the control, the secondary structures of all films were significantly changed (p < 0.05). In particular, the occurrences of antioxidants leaded to the significant decreases of α-Helix, and the increases of β-sheet as well as the decreases of random-coil conformation in gelatin films (p < 0.05), except from the films containing tea polyphenols. For the samples with tea polyphenols, the percentage of random-coil conformations was significantly improved regardless of the concentration (p < 0.05). Thus, functional groups and secondary structures of gelatins in films were substantially impacted by antioxidants. In literature, it was found that the secondary structures of gelatin were pronouncedly affected by pH value. Acidic condition enhanced the formation random coils and beta-turn while decreasing the amount of a-helix. Meanwhile, alkali condition increased the amounts of random coil and a-helix.[Citation61]
TABLE 5 Summary of FTIR amide I deconvolution of gelatin-calcium carbonate films containing different plant-based antioxidants
DPPH Radical Scavenging Assay
As one of major categories of bioactive packaging films, the film enriching antioxidants is a rather promising approach for extending the shelf life of food products without sacrificing nutritional and organoleptic properties.[Citation62] As one of the most popular and frequently methods used in the evaluation of compounds to act as free radical scavengers or hydrogen donors, the DPPH radical scavenging capacity is a central method to evaluate the antioxidant capacity of a product. The DPPH radical is viewed as a sort of durable organic nitrogen radical with a deep purple color. Compared to other scavenging assays, it is commercially available and convenient since it has no requirement for being generated immediately prior to the assay.[Citation63]
demonstrated the DPPH radical scavenging activity of gelatin-based films in the presence of different antioxidants. The results revealed that the addition of the higher concentration of natural antioxidants enabled the strongest scavenging activity against DPPH radical, which is significantly higher than of the control and the lower concentration group, respectively (p < 0.05). Concurrently, the film with the lower concentration of natural antioxidants exhibited the significantly higher DPPH radical scavenging activity than that of the control (p < 0.05), although the pure gelatin film possessed antioxidant activities. At the lower concentration group, the order of the antioxidant capacities of films was listed as: sodium ascorbate > d-sodium erythorbate > tea polyphenols. As a matter of fact, the sequence of the higher concentration group was expressed as: sodium ascorbate > d-sodium erythorbate= tea polyphenols. Compared to all of the antioxidants in the low concentration group, 30.3% of the increment of the antioxidant capacity of tea polyphenols in the high concentration group was significant than other two antioxidants (14.6% for the d-sodium erythorbate group; 8.1% for the sodium ascorbate group). These results confirmed that the increase in the concentration of the antioxidant favors its antioxidant capacity at a fixed range.
FIGURE 3 DPPH scavenging efficiency of gelatin-calcium carbonate films containing different natural antioxidants with the low concentration group (0.1 mg/mL) and the high concentration group (0.4 mg/mL); The control: the gelatin-calcium carbonate film in the absence of the antioxidants.
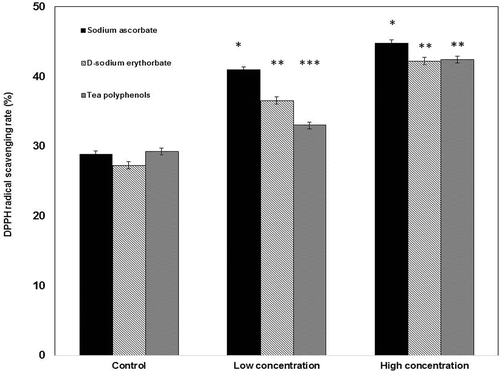
Alternatively, antioxidant capacity appears to be concentration-dependent. Indeed, the enhanced magnitude of the antioxidant capacity depends on its inherent property. The results of DPPH radical scavenging activity of sodium ascorbate are consistent with those in literature.[Citation64] For sodium erythorbate, it could be affected sensitively in the occurrence of oxygen, metal ions, and light,[Citation33] including the release of electrons absorbed by the erythorbate molecule.[Citation33] These reactions allowed for the prevention of those chain reactions oxidizing other compounds in foods. The antioxidant activity of tea polyphenols is greatly associated with the number and position of phenolic hydroxyl groups.[Citation34,Citation65] Therefore, different chemical structures of antioxidants leaded to the different antioxidant capabilities.
CONCLUSION
In this study, different natural antioxidants were successfully incorporated into gelatin-calcium carbonate composite films. Antioxidants enabled the promotion of mechanical and water-barrier properties of films, and modification of the surface morphology of the films. In addition, all films containing antioxidants exhibited the significant antioxidant activities. The results illustrate the potential application of gelatin-calcium carbonate composite films in food packages.
FUNDING
Financial support was kindly supplied by grants from the Foundation of National 863 Plan (No. 2013AA102204) and Ministry of Agriculture of China (No. 201303082-3).
Additional information
Funding
REFERENCES
- Wu, X.; Wang, S. Regulating MC3T3-E1 cells on deformable poly (ϵ-caprolactone) honeycomb films prepared using a surfactant-free breath figure method in a water-miscible solvent. ACS Applied Materials & Interfaces 2012, 4, 4966–4975.
- Wu, X.; Wang, S. Integration of photo-crosslinking and breath figures to fabricate biodegradable polymer substrates with tunable pores that regulate cellular behavior. Polymer 2014, 55, 1756–1762.
- Feng, T.; Ye, R.; Zhuang, H.; Rong, Z.; Fang, Z.; Wang, Y.; Gu, Z.; Jin, Z. Physicochemical properties and sensory evaluation of Mesona Blumes gum/rice starch mixed gels as fat-substitutes in Chinese Cantonese-style sausage. Food Research International 2013, 50, 85–93.
- Feng, T.; Ye, R.; Zhuang, H.; Fang, Z.; Chen, H. Thermal behavior and gelling interactions of Mesona Blumes gum and rice starch mixture. Carbohydrate Polymers 2012, 90, 667–674.
- Feng, T.; Su, Q.; Zhuang, H.; Ye, R.; Gu, Z.; Jin, Z. Ghost structures, pasting, rheological and textural properties between mesona blumes gum and various starches. Journal of Food Quality 2014, 37, 73–82.
- Ye, R.; Harte, F. Casein maps: Effect of ethanol, pH, temperature, and CaCl2 on the particle size of reconstituted casein micelles. Journal of Dairy Science 2013, 96, 799–805.
- Chen, Y.; Ye, R.; Li, X.; Wang, J. Preparation and characterization of extruded thermoplastic zein-poly (propylene carbonate) film. Industrial Crops and Products 2013, 49, 81–87.
- Chen, Y.; Ye, R.; Liu, J. Understanding of dispersion and aggregation of suspensions of zein nanoparticles in aqueous alcohol solutions after thermal treatment. Industrial Crops and Products 2013, 50, 764–770.
- Chen, Y.; Ye, R.; Liu, J. Effects of different concentrations of ethanol and isopropanol on physicochemical properties of zein-based films. Industrial Crops and Products 2014, 53, 140–147.
- Feng, T.; Ye, R. Rheological behavior of biopolymer systems, handbook of biopolymer-based materials. From Blends and Composites to Gels and Complex Networks 2013, 673–698.
- Hayes, D.G.; Mannam, V.K.; Ye, R.; Zhao, H.; Ortega, S.; Montiel, M.C. Modification of oligo-ricinoleic acid and its derivatives with 10-undecenoic acid via lipase-catalyzed esterification, Polymers 2012, 4, 1037–1055.
- Ye, R.; Pyo, S.-H.; Hayes, D.G. Lipase-catalyzed synthesis of saccharide-fatty acid esters using suspensions of saccharide crystals in solvent-free media. Journal of the American Oil Chemists’ Society 2010, 87, 281–293.
- Ye, R.; Hayes, D.G. Solvent-free lipase-catalysed synthesis of saccharide-fatty acid esters: Closed-loop bioreactor system with in situ formation of metastable suspensions. Biocatalysis and Biotransformation 2012, 30, 209–216.
- Ye, R.; Hayes, D.G. Lipase-catalyzed synthesis of saccharide-fatty acid esters utilizing solvent-free suspensions: Effect of acyl donors and acceptors, and enzyme activity retention. Journal of the American Oil Chemists’ Society 2012, 89, 455–463.
- Mackie, A.; Gunning, A.; Ridout, M.; Morris, V. Gelation of gelatin observation in the bulk and at the air‐water interface. Biopolymers 1998, 46, 245–252.
- Fu, Y.; Zhao, X.-H. Utilization of chum salmon (Oncorhynchus keta) skin gelatin hydrolysates to attenuate hydrogen peroxide-induced oxidative injury in rat hepatocyte BRL cell model. Journal of Aquatic Food Product Technology 2014, DOI:10.1080/10498850.2013.804141.
- Nowzari, F.; Shábanpour, B.; Ojagh, S.M. Comparison of chitosan-gelatin composite and bilayer coating and film effect on the quality of refrigerated rainbow trout. Food Chemistry 2013, 141, 1667–1672.
- Harper, B.; Barbut, S.; Lim, L.-T.; Marcone, M. Characterization of “wet” alginate and composite films containing gelatin, whey, or soy protein. Food Research International 2013, 52, 452–459.
- Ortiz-Zarama, M.; Jiménez-Aparicio, A.; Perea-Flores, M.; Solorza-Feria, J. Barrier, mechanical, and morpho-structural properties of gelatin films with carbon nanotubes addition. Journal of Food Engineering 2014, 120, 223–232.
- Farahnaky, A.; Dadfar, S.M.M.; Shahbazi, M. Physical and mechanical properties of gelatin-clay nanocomposite. Journal of Food Engineering 2014, 122, 78–83.
- Jorge, M.F.C.; Caicedo Flaker, C.H.; Nassar, S.F.; Moraes, I.C.F.; Bittante, A.M.Q.B.; do Amaral Sobral, P.J. Viscoelastic and rheological properties of nanocomposite-forming solutions based on gelatin and montmorillonite. Journal of Food Engineering 2014, 120, 81–87.
- Long, Y.; Song, K.; York, D.; Zhang, Z.; Preece, J.A. Engineering the mechanical and physical properties of organic-inorganic composite microcapsules. Colloids and Surfaces A: Physicochemical and Engineering Aspects 2013, 433, 30–36.
- Wu, X.; Wei, J.; Lu, X.; Lv, Y.; Chen, F.; Zhang, Y.; Liu, C. Chemical characteristics and hemostatic performances of ordered mesoporous calcium-doped silica xerogels. Biomedical Materials 2010, 5, 035006.
- Zhou, H.; Wei, J.; Wu, X.; Shi, J.; Liu, C.; Jia, J.; Dai, C.; Gan, Q. The bio-functional role of calcium in mesoporous silica xerogels on the responses of osteoblasts in vitro. Journal of Materials Science: Materials in Medicine 2010, 21, 2175–2185.
- Wu, X.; Wang, S. Biomimetic calcium carbonate concentric microgrooves with tunable widths for promoting MC3T3‐E1 cell functions. Advanced Healthcare Materials 2010, 2, 326–333.
- Umaretiya, P.J.; Thacher, T.D.; Fischer, P.R.; Cha, S.S.; Pettifor, J.M. Bone mineral density in Nigerian children after discontinuation of calcium supplementation. Bone 2013, 55, 64–68.
- Li, X.Q.; Zeng, H.C. Calcium carbonate nanotablets: Bridging artificial to natural nacre. Advanced Materials 2012, 24, 6277–6282.
- Dongya, Y.; Hanqing, Z.; Fengxian, Q.; Li, H. Investigation of polyurethane (urea)/modified nano‐calcium carbonate hybrid aqueous dispersions and their films. Journal of Applied Polymer Science 2012, 125, 2896–2901.
- Wang, Y.; Liu, A.; Ye, R.; Wang, W.; Li, X. Transglutaminase-induced crosslinking of gelatin-calcium carbonate composite films. Food Chemistry 2015, 166, 414–422.
- Pokorný, J. Are natural antioxidants better—and safer—than synthetic antioxidants? European Journal of Lipid Science and Technology 2007, 109, 629–642.
- Burt, S. Essential oils: Their antibacterial properties and potential applications in foods—A review. International Journal of Food Microbiology 2004, 94, 223–253.
- Zhang, Y.; Zhang, H.; Wang, L.; Guo, X.; Qi, X.; Qian, H. Antioxidant properties and radical-scavenging activity of ethanol extract of defatted peanut meal. International Journal of Food Properties 2012, 22, 201–207.
- Karpińska-Tymoszczyk, M. The effect of sage, sodium erythorbate, and a mixture of sage and sodium erythorbate on the quality of turkey meatballs stored under vacuum and modified atmosphere conditions. British Poultry Science 2010, 51, 745–759.
- Fujimoto, A.; Masuda, T. Antioxidation mechanism of rosmarinic acid, identification of an unstable quinone derivative by the addition of odourless thiol. Food Chemistry 2012, 132, 901–906.
- Jeya Shakila, R.; Jeevithan, E.; Varatharajakumar, A.; Jeyasekaran, G.; Sukumar, D. Comparison of the properties of multi-composite fish gelatin films with that of mammalian gelatin films. Food Chemistry 2012, 135, 2260–2267.
- Chiou, B.-S.; Avena-Bustillos, R.J.; Bechtel, P.J.; Imam, S.H.; Glenn, G.M.; Orts, W.J. Effects of drying temperature on barrier and mechanical properties of cold-water fish gelatin films. Journal of Food Engineering 2009, 95, 327–331.
- Jridi, M.; Souissi, N.; Mbarek, A.; Chadeyron, G.; Kammoun, M.; Nasri, M. Comparative study of physico-mechanical and antioxidant properties of edible gelatin films from the skin of cuttlefish. International Journal of Biological Macromolecules 2013, 61, 17–25.
- Tongnuanchan, P.; Benjakul, S.; Prodpran, T. Characteristics and antioxidant activity of leaf essential oil-incorporated fish gelatin films as affected by surfactants. International Journal of Food Science & Technology 2013, 48, 2143–2149.
- Wang, J.; Gu, H.; Zhao, Z.; Sheng, X.; Yu, H.; Huang, W. Characterization of the appearance, health-promoting compounds, and antioxidant capacity of the florets of the loose-curd cauliflower. International Journal of Food Properties 2015, 18, 392–402.
- Adnan, L.; Osman, A.; Abdul Hamid, A. Antioxidant activity of different extracts of red pitaya (Hylocereus polyrhizus) seed. International Journal of Food Properties 2011, 14, 1171–1181.
- Cho, S.; Gu, Y.; Kim, S. Extracting optimization and physical properties of yellowfin tuna (Thunnus albacares) skin gelatin compared to mammalian gelatins. Food Hydrocolloids 2005, 19, 221–229.
- Jongjareonrak, A.; Benjakul, S.; Visessanguan, W.; Prodpran, T.; Tanaka, M. Characterization of edible films from skin gelatin of brownstripe red snapper and bigeye snapper. Food Hydrocolloids 2006, 20, 492–501.
- Manroshan, S.; Baharin, A. Effect of nanosized calcium carbonate on the mechanical properties of latex films. Journal of Applied Polymer Science 2005, 96, 1550–1556.
- Kanmani, P.; Rhim, J.-W. Physical, mechanical, and antimicrobial properties of gelatin based active nanocomposite films containing AgNPs and nanoclay. Food Hydrocolloids 2014, 35, 644–652.
- Siripatrawan, U.; Noipha, S. Active film from chitosan incorporating green tea extract for shelf life extension of pork sausages. Food Hydrocolloids 2012, 27, 102–108.
- Cao, N.; Fu, Y.; He, J. Preparation and physical properties of soy protein isolate and gelatin composite films. Food Hydrocolloids 2007, 21, 1153–1162.
- Hale, W.; Dohrer, K.; Tant, M.; Sand, I. A diffusion model for water vapor transmission through microporous polyethylene/CaCO3 films. Colloids and Surfaces A: Physicochemical and Engineering Aspects 2001, 187, 483–491.
- Fakhreddin Hosseini, S.; Rezaei, M.; Zandi, M.; Ghavi, F.F. Preparation and functional properties of fish gelatin-chitosan blend edible films. Food Chemistry 2013, 136, 1490–1495.
- Tongnuanchan, P.; Benjakul, S.; Prodpran, T. Physico-chemical properties, morphology, and antioxidant activity of film from fish skin gelatin incorporated with root essential oils. Journal of Food Engineering 2013, 117, 350–360.
- McHugh, T.H.; Avena‐Bustillos, R.; Krochta, J. Hydrophilic edible films: Modified procedure for water vapor permeability and explanation of thickness effects. Journal of Food Science 1993, 58, 899–903.
- Rubilar, J.F.; Cruz, R.; Silva, H.D.; Vicente, A.A.; Khmelinskii, I.; Vieira, M.C. Physico-mechanical properties of chitosan films with carvacrol and grape seed extract. Journal of Food Engineering 2013, 115, 466–474.
- Siripatrawan, U.; Harte, B.R. Physical properties and antioxidant activity of an active film from chitosan incorporated with green tea extract. Food Hydrocolloids 2010, 24, 770–775.
- Bonilla. J.; Talón, E.; Atarés, L.; Vargas, M.; Chiralt, A. Effect of the incorporation of antioxidants on physicochemical and antioxidant properties of wheat starch-chitosan films. Journal of Food Engineering 2013, 118, 271–278.
- Li, J.-H.; Miao, J.; Wu, J.-L.; Chen, S.-F.; Zhang, Q.-Q. Preparation and characterization of active gelatin-based films incorporated with natural antioxidants. Food Hydrocolloids 2014, 37, 166–173.
- Pal, M.K.; Singh, B.; Gautam, J. Thermal stability and UV-shielding properties of polymethyl methacrylate and polystyrene modified with calcium carbonate nanoparticles. Journal of Thermal Analysis and Calorimetry 2012, 107, 85–96.
- Farris, S.; Schaich, K.M.; Liu, L.; Cooke, P.H.; Piergiovanni, L.; Yam, K.L.Gelatin-pectin composite films from polyion-complex hydrogels. Food Hydrocolloids 2011, 25, 61–70.
- Wu, J.; Chen, S.; Ge, S.; Miao, J.; Li, J.; Zhang, Q. Preparation, properties, and antioxidant activity of an active film from silver carp (Hypophthalmichthys molitrix) skin gelatin incorporated with green tea extract. Food Hydrocolloids 2013, 32, 42–51.
- Chen, X.; Ayres, N. Synthesis of novel polymer/urea peptoid conjugates using RAFT polymerization. Macromolecules 2010, 43, 1341–1348.
- Aewsiri, T.; Benjakul, S.; Visessanguan, W. Functional properties of gelatin from cuttlefish (Sepia pharaonis) skin as affected by bleaching using hydrogen peroxide. Food Chemistry 2009, 115, 243–249.
- Bergo, P.; Sobral, P. Effects of plasticizer on physical properties of pigskin gelatin films. Food Hydrocolloids 2007, 21, 1285–1289.
- Liu, H.; Wang, B.; Barrow, C.J.; Adhikari, B. Relating the variation of secondary structure of gelatin at fish oil-water interface to adsorption kinetics, dynamic interfacial tension, and emulsion stability. Food Chemistry 2014, 143, 484–491.
- Guilbert, S.; Gontard, N.; Gorris, L.G. Prolongation of the shelf-life of perishable food products using biodegradable films and coatings. LWT-Food Science and Technology 1996, 29, 10–17.
- Pyrzynska, K.; Pękal, A. Application of free radical diphenylpicrylhydrazyl (DPPH) to estimate the antioxidant capacity of food samples. Analytical Methods 2013, 5, 4288–4295.
- Liu, D.; Shi, J.; Colina Ibarra, A.; Kakuda, Y.; Jun Xue, S. The scavenging capacity and synergistic effects of lycopene, vitamin E, vitamin C, and β-carotene mixtures on the DPPH free radical. LWT-Food Science and Technology 2008, 41, 1344–1349.
- Zhang, Y.; Li, Q.; Xing, H.; Lu, X.; Zhao, L.; Qu, K.; Bi, K. Evaluation of antioxidant activity of ten compounds in different tea samples by means of an on-line HPLC-DPPH assay. Food Research International 2013, 53, 847–856.