Abstract
Peroxidase enzyme was purified for the first time from white cabbage (Brassica oleracea var. capitata f. alba) in a single step using affinity chromatography and some biochemical characteristics of the purified enzyme were determined. The peroxidase was purified 24.7-fold with an overall recovery of 4.3% and a specific activity of 964.5. The molecular weight of the purified peroxidase was approximately 73.2 kDa as calculated by sodium dodecyl sulfate-polyacrylamide gel electrophoresis and it showed maximum activity at pH 6.5 and 30°C. For the guaiacol substrate, the KM and Vmax values were found as 3.19 mM and 0.2 EU/mL, respectively. Additionally, the IC50 and Ki values were determined as 0.517 and 0.994 ± 0.453 mM, respectively, for 4-aminobenzohydrazide. 4-amino benzohydrazide showed non-competitive inhibition.
INTRODUCTION
Cabbage (Brassica oleracea L. var. capitata) is one of the most important vegetables grown worldwide. Many epidemiological studies have reported that the relationship between the consumption of Brassicaceae plants and protection against numerous chronic diseases such as cancer, neurological, and cardiovascular diseases.[Citation1−Citation3] The different cultivated types of cabbage show great variation in respect of size, shape, and color.[Citation4] White cabbage (Brassica oleracea L. var. capitata alba) is one of the most important member of the Brassicaceae family and it is an economically important source of dietary and various bioactive compounds (antioxidant and anticarcinogenic).[Citation3,Citation5,Citation6] Numerous studies have been reported about the chemical components (vitamins A, C, K, vitamin B6 and B3, flavonoids, carotenoids, etc.) of white cabbage (Brassica oleracea var. capitata f. alba),[Citation1,Citation3,Citation4,Citation6] but no attempt has yet been made to purify and characterize peroxidase (POD) enzyme.
PODs (E.C. 1.11.1.7) are found in different kinds of living organisms (plants, vertebrates, fungi, and microorganisms) in nature.[Citation7,Citation8] Particularly in plants, they are involved in a variety of biochemical and physiological functions. Wound healing, synthesis of cell wall components, hormone regulation, general stress response, protection of tissue from physical damage, and control of defense mechanisms against pathogens are some of the proposed functions.[Citation9−Citation13] In addition, PODs play role conserving food quality including undesirable flavor and color changes.[Citation13,Citation14] They catalyze the oxidation reaction of a wide variety of substrates including phenolic compounds in the presence of H2O2[Citation7,Citation8] Due to their high sensitivity, wide substrate specificity, and broader catalytic activity, PODs are becoming increasingly attractive for numerous applications in the biotechnological, chemical, and food industries.[Citation7−Citation9] Therefore, there is great interest in the purification and characterization of PODs from different sources, and numerous studies have been performed to find alternative sources of POD with increased availability, higher stability, and different substrate specificity. Some of these sources are turnip (Brassica rapa L.) roots,[Citation8] Turkish black radish,[Citation7,Citation8] papaya (Carica papaya) fruit,[Citation10] sweet potato tubers,[Citation12] sweet gourd (Cucurbita moschata Lam. Poiret),[Citation13] leaves of chard (Beta vulgaris subspecies cicla),[Citation14] olive (Olea europaea L.),[Citation15] black gram,[Citation16] Leucaena leucocephala,[Citation17] caribbean plant (Euphorbia cotinifolia),[Citation18] cauliflower (Brassica oleracea L. var. botrytis) buds,[Citation19] buckwheat,[Citation20] oil palm (Elaeis guineensis Jacq.) leaf,[Citation21] and cotton (Gossypium hirsutum).[Citation22] As previously mentioned, until now PODs have been purified and characterized from a wide variety of plant sources, but no attempt has yet been made to purify and characterize POD from white cabbage (Brassica oleracea var. capitata f. alba).
4-amino benzohydrazide is a well-known POD inhibitor. For this reason 4-amino benzohydrazide and its derivatives can be used as a ligand for POD purification.[Citation23,] In a recent study, Kalin et al. used 4-amino benzohydrazide affinity chromatography to purify POD from Turkish black radish (Raphanus sativus L.) and turnip (Brassica rapa L.) roots. Similarly, in the present study, the affinity matrix was prepared as described[Citation8] and was used to purify POD enzyme from white cabbage (Brassica oleracea var. capitata f. alba). The aim of the present study was to purify POD enzyme for the first time from white cabbage (Brassica oleracea var. capitata f. alba) in a single step by using 4-amino benzohydrazide affinity chromatography. After purification of the POD enzyme, to determine its physicochemical and kinetic characteristics some biochemical characteristics such as optimum pH, optimum temperature, optimum ionic strength, substrate specificity, and kinetic constants (KM and Vmax), of the purified enzyme were determined. Furthermore, Ki and IC50 values and inhibition types were also determined for 4-amino benzohydrazide.
MATERIALS AND METHODS
Chemicals and Materials
CNBr-activated-Sepharose 4B, L-tyrosine, 4-amino benzohydrazide, guaiacol, H2O2, Coomassie Brilliant Blue R, potassium phosphate (monobasic), glycerol, and all other reagents used in the electrophoresis and protein assay were obtained from Sigma Aldrich. A freezer (Sanyo, Ultra Low, –86°C, Japan) was used to protect the purified enzyme and other chemicals. In the experiments, a UV-Vis spectrophotometer (Beckman Coulter Du 730, USA) was used to monitor the changes in absorbance at 280, 470, and 595 nm, an ultra turrax (Heidolph Silient Crusher M, Germany) was used to obtain plant homogenate, a peristaltic pump (Ismatec) was used to adjust the flow rate of the affinity column, a pH-meter (SCHOTT CG840, Germany) was used to adjust the pH, a temperature controlled centrifuge (HermleZ 323K, Germany) was used to centrifuge and a temperature controlled circulating water bath (Grant LTD 6G –20 to 100°C) was used to control the temperature. A sodium dodecyl sulfate-polyacrylamide gel electrophoresis (SDS-PAGE; Mini-Protean, Bio-Rad, USA) was used to determine purity and molecular weight of purified POD. All chemicals were of analytical grade.
Plant Materials
The fresh white cabbage (Brassica oleracea var. capitata f. alba) used in this work, was obtained from a local market in Erzurum, Turkey. It was washed, drained, and packed. Only the root was used for POD purification. The samples were stored at –20°C until use.
Preparation of the Crude Enzyme Extract
The homogenate was prepared according to the procedure described previously by Kalın et al.[Citation8] For this purpose, 20 g of white cabbage root was cut into small pieces, mixed with liquid nitrogen, and crushed in a mortar. Then 50 mL of KH2PO4 (0.3 M, pH: 7.0), was mixed to a slurry and this was homogenized with an ultra turrax (Heidolph Silient Crusher M) operated at maximum speed for 5 min. After homogenization, the slurry was centrifuged at 16.000 xg for 60 min at 4ºC using a temperature controlled centrifuge (HermleZ 323K). Finally, the pellet was separated and discarded, and the supernatant was stored at –20°C in small aliquots until use.
Purification of POD by 4-Amino-Benzohydrazide Affinity Chromatography
A 4-amino-benzohydrazide affinity matrix and affinity column (1 × 10 cm) were prepared according to the described previously published procedure with a slight modification[Citation8,Citation24] In the study, a cyanogen bromide (CNBr)-activated-Sepharose-4B material was used as a matrix, and L-tyrosine was coupled as the spacer arm while 4-amino benzohydrazide was used as the affinity ligand. After preparation of the affinity column, POD enzyme extract was loaded onto it. Then, the prepared affinity column was washed with a sufficient amount of equilibrating buffer (10 mM; pH: 6.8, 400 mL) and elution of the POD enzyme from the column was performed with an eluting solution (pH: 6.8, 1 M NaCl/25 mM KH2PO4) using a peristaltic pump (Ismatec) at 35 mL h−1 flow rate. The active fractions were collected every 1.5 mL into tubes and the absorbance and activity () measurements of each were performed separately at 280 and 470 nm, respectively. Eluted active fractions were pooled and kept at +4°C for further use.
SDS-PAGE
After purification of the POD, SDS-PAGE was used to assess purification degree as well as to estimation molecular weight (Mw) of purified POD. SDS-PAGE (Mini-Protean, Bio-Rad) was performed as described.[Citation25] Samples containing approximately 20 μL of protein were loaded on the electrophoresis medium after mixing with a sample loading buffer. Standard proteins (Catalog No: 26630 Page Ruler Broad Range Unstained Protein Ladder, 2 × 250 μL) were also applied to the electrophoresis medium. When electrophoresis was completed, the protein bands were stained with silver staining.[Citation26]
Qualitative and Quantitative Protein Determination
Qualitative and quantitative protein determination was performed on the eluates at 280 and 595 nm, respectively, with a UV-VIS spectrophotometer (Beckman Coulter Du 730; ). Protein concentration was determined as described by Bradford[Citation27] using bovine serum albumin (BSA).
POD Activity Assay
POD activity was monitored spectrophotometrically at 470 nm by following the oxidation of guaiacol to 3,3’-Dimethoxy-4,4’-biphenoquinone.[Citation28] For this purpose, a standard reaction medium containing 1 mL of 22.5 mM hydrogen peroxide and 1 mL of 45 mM guaiacol was prepared. Then 900 μL of 0.1 M phosphate buffer (pH: 6.0) solution and 100 μL of enzyme were added to the standard reaction medium. The changes in absorbance were monitored for 3 min at 25°C and one unit of enzyme activity was defined as the amount of enzyme that changes the absorbance by 0.01 per min.[Citation7,Citation8] During the experiments, a temperature controlled circulating water bath was used to control temperature. All experiments were performed in triplicate.
Optimum pH
The effect of pH on the activity of purified POD was investigated in the presence of buffer with different pH levels (0.1 M K2HPO4, pH: 5.0–8.0) in the standard assay mixture.[Citation7,Citation11] As stated previously, the standard assay mixture contained 1 mL of 22.5 mM hydrogen peroxide, 1 mL of 45 mM guaiacol, 100 μL of enzyme, and 900 μL of buffer solution.
Optimum Temperature
The effect of temperature on POD activity was studied at optimum pH by incubating the reaction mixture at various temperatures (0–80°C) at 5°C steps. For this purpose, 900 μL of phosphate buffer solution was added to the standard reaction medium in a test tube, and this reaction mixture was incubated for a fixed time of 5 min in a thermostatic water bath (Grant LTD 6G –20 to 100°C) at the required temperature. Then 100 μL of the enzyme was added to the reaction mixture at the same temperature, and POD activity was determined spectrophotometrically as rapidly as possible.
Stable pH
For pH stability, activity of POD was followed in 10 mM KH2PO4 buffer, with a pH range of pH 5.0 to pH 8.0, for 5 days.
Effect of Ionic Strength
Different concentrations of KH2PO4 buffers (25 mM–1 M) were used to determine the effect of ionic strength on POD activity at the predetermined optimal pH.
Kinetic Constants
The substrate specificity of POD was examined using a well-known POD substrate (guaiacol). To determine the substrate specificity of white cabbage (Brassica oleracea var. capitata f. alba) POD, the POD activity assay was performed spectrophotometrically at 470 nm by following the oxidation of guaiacol to 3,3’-Dimethoxy-4,4’-biphenoquinone.[Citation28] The Vmax and KM values were calculated from a plot of 1/V versus 1/[S] according to the method of Lineweaver and Burk.[Citation29] In the experiments, 0.1 mL of the purified enzyme was incubated with a fixed concentration of H2O2 and five different concentrations of guaiacol or vice versa. For this purpose, different amounts of the substrate stock solution (0.1, 0.2, 0.3, 0.4, 0.5, 0.6, and 0.7 mL) were completed to a final volume of 1.9 mL with the appropriate amount of buffer solution. Then, 1 mL of H2O2 was added to the mixture. Finally, 100 μL of the enzyme solution was added and the enzyme activity was measured as rapidly as possible at 470 nm.
TABLE 1 Levels of purification of white cabbage (Brassica oleracea var. capitata f. alba)
Inhibition Effects of 4-Amino Benzohydrazide
The inhibition kinetics were determined following the procedure of Lineweaver and Burk.[Citation29] To obtain the IC50 value, the POD activity was measured in the presence of different concentrations of inhibitor ([4-aminobenzohydrazide]: 0.114–0.853 mM) for the white cabbage (Brassica oleracea var. capitata f. alba). A control sample without 4-aminobenzohydrazide was used to determine 100% activity, and an Activity-[4-amino benzohydrazide] plot was drawn. To calculate the Ki value, three different inhibitor concentrations ([4-amino benzohydrazide]; 0.114, 0.398, and 0.682 mM) were used. Guaiacol was used as the substrate at five different concentrations (4–8 mM). Lineweaver-Burk plots (1/V–1/[S]) were obtained for the 4-amino benzohydrazide, the Ki value was calculated and the inhibition type was also determined from the graph. All experiments were performed in triplicate and for Ki value, result was given as mean ± SD.
RESULTS AND DISCUSSION
Purification of POD from Affinity Column Chromatography
During the purification of POD from affinity column chromatography, eluates were collected as 1.5 mL fractions and the absorbance and activity () measurements of each eluate were performed separately at 280 and 470 nm, respectively. Quantitative protein determinations and activity measurements were performed on the crude extract and all eluted fractions. To the authors’ knowledge, it is the first successful attempt to purify and characterize POD from white cabbage (Brassica oleracea var. capitata f. alba) by affinity chromatography.
The POD enzyme purification is summarized in . The POD was purified 24.7-fold with overall recovery of 4.3% and with a specific activity of 964.5, from the affinity column. In the literature, there are several studies on the purification of POD from different plant sources. The POD enzyme was purified from Turkish black radish (Raphanus sativus L) with a yield of 2% and a factor of 9.7-fold by ammonium sulphate precipitation, dialysis and CM-Sephadex ion exchange chromatography,[Citation7] from turnip (Brassica rapa L) roots by an affinity chromatography with a yield of 9%, and a factor of 269.13-fold,[Citation8] from sweet gourd (Cucurbita moschata Lam. Poiret) by ammonium sulphate precipitation and CM-Sephadex ion-exchange chromatography with a yield of 12% and a factor of 10.2-fold,[Citation13] from chard (Beta vulgaris subspecies cicla) by ammonium sulphate precipitation, dialyses, and CM-sephadex cation exchange chromatography with a yield of 14.4% and a factor of 36-fold,[Citation14] from cauliflower (Brassica oleracea L.) buds by ammonium sulphate precipitation, dialysis, CM-Sephadex ion-exchange chromatography, and Sephadex G-25 with a yield of 0.2%, and a factor of 19.33-fold,[Citation19] from spinach by ammonium sulphate precipitation and CM-Sephadex ion exchange chromatography with a yield of 4.2% yield and a factor of 22.6-fold.[Citation30]
Determination of Molecular Weight and Purity
A SDS-PAGE image and a Log MW-Rf volume standard graph are shown in and , respectively. As shown in , the POD purified from white cabbage (Brassica oleracea var. capitata f. alba) exhibited only one band on the SDS-PAGE image. The molecular weight of the purified POD was calculated as 73.2 kDa using the formula obtained from the standard graph () according to Laemmli’s method.[Citation25] This molecular weight is in the range of the molecular weights that have been reported in previous studies. Until now, PODs have been purified from several fruits and vegetables with different molecular weights ranging from 35 to 95 kDa, depending on the purified source. To give examples of these studies, in one study, Deepa and Arumughan[Citation21] found that POD purified from oil palm (Elaeis guineensis jacq.) leaf had a molecular weight of 48 kDa. Gülçin and Yildirim[Citation31] reported that POD purified from black cabbage (B. oleracea var. acephala) had a molecular weight of 95 kDa. On the other hand, Köksal and Gülçin[Citation19] found that POD purified from fresh cauliflower (Brassica oleracea L.) buds had a molecular weight of 44 kDa. The molecular weight was found to be 37 kDa in horseradish (Armoracia rusticana) by Lavery et al.[Citation32] In recent study, Kalin et al.[Citation8] reported that the molecular weights of purified POD from turnip (Brassica rapa L.) and Turkish black radish (Raphanus sativus L.) had molecular weights of 65.8 and 67.3 kDa, respectively.
FIGURE 2 (a) SDS-PAGE image of purified POD. Column a: Standard proteins, Column b: Purified peroxidase from Brassica oleracea var. capitata f. alba. The standard proteins used for SDS-PAGE were highly purified proteins ranging from 5 to 250 kDa (Catalog No: 26630 Page Ruler Broad Range Unstained Protein Ladder, 2 × 250 μL). (b) Standard Log MW-Rf graph of POD purified from Brassica oleracea var. capitata f. rubra using SDS-PAGE. The standard proteins used for SDS-PAGE were highly purified proteins ranging from 5 kDa to 250 kDa (Catalog No: 26630 Page Ruler Broad Range Unstained Protein Ladder, 2 × 250 μL).
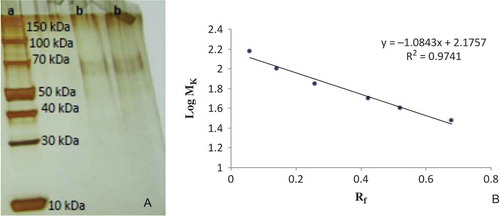
Determination of Biochemical Properties
The pH changes the ionization states of amino acid side chains and the three-dimensional structure of the enzyme. Medium pH also alters the ionization of the substrate. Therefore, pH is one of the most important factors to affect enzymatic activity. As shown in , the POD activity showed a maximum at pH 6.5 in 0.1 M KH2PO4 buffer solution but decreased markedly for higher and lower values of this pH. The optimum pH values of POD have been widely studied in various plant sources such as tomato, coconut, cauliflower buds, rice, papaya fruit, cotton, garlic, Turkish black radish etc., and the optimum pH values of POD purified from these sources are mostly in the range between 4.5 and 7.5. The optimum pH for sweet gourd[Citation13] is 7.2, for olive[Citation15] it is 7.0, for Turkish black radish[Citation8] and strawberry[Citation33] it is 6.0, for leaves of chard,[Citation14] tomato,[Citation34] and soybean[Citation35] it is 5.5, and for rice[Citation36] and cauliflower buds[Citation19] it is 5.0. The optimum pH value of the purified POD is in the range of pH values that have been reported previously. It is known that the optimum pH of an enzyme varies according to source and substrate types. The stability of POD, at various pH values, was also studied ().
FIGURE 3 (a) The effect of pH on POD activity. (b) Stable pH profile of purified POD from white cabbage (Brassica oleracea var. capitata f. alba).The enzyme activity was measured in phosphate buffer (10 mM; pH: 5.0–8.0) once every 12 hours for 96 hours.
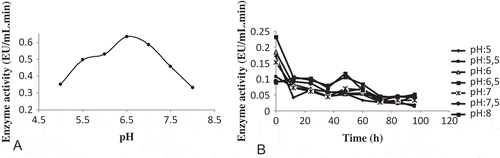
It is reported that POD is one of the most heat resistant enzymes in plants. However, the resistance of an enzyme to heat depends on the assay conditions, pH, substrate nature, and source type. In this study, the optimum temperature was found to be 30ºC for the POD of white cabbage (Brassica oleracea var. capitata f. alba; ). Previous studies on POD have typically determined an optimum temperature in the range of 30 to 70ºC. The optimum temperature for Turkish black radish POD is 30°C,[Citation8] for papaya fruit[Citation10] and leaves of chard[Citation14] it is 40°C, for sweet gourd[Citation13] and edible mushroom[Citation37] it is 50°C, and for green asparagus it is 70°C.[Citation38]
The specific activity levels were measured using a phosphate buffer solution at optimum pH. The concentration of buffers was in the range between 25 mM and 1 M. Based on these activity measurements, the POD activity was the highest at 0.6 M ( and ).
TABLE 2 Kinetic parameters of purified POD and IC50 and Ki values and inhibition type of 4-amino benzohydrazide (All experiments were performed in triplicate and for Ki value, result was given as mean ± SD)
The kinetic parameters of purified POD (Michaelis constant and maximum reaction velocity; KM and Vmax) were determined with guaiacol and hydrogen peroxide. As is known, KM and Vmax are characteristic values for an enzyme. KM shows the stability of the enzyme-substrate complex. When the KM value grows the enzyme-substrate complex is weakened. The higher the KM value, the weaker the enzyme-substrate complex; in other words, a high KM value indicates that the affinity of the enzyme for its substrate is low. On the other hand, Vmax is the maximum rate of an enzyme-catalyzed reaction. For this reason, determination of these characteristic values is important. In this study, the KM and Vmax values were calculated to be 3.19 mM and 0.2 EU/mL min, respectively, by a Lineweaver-Burk plot for the guaiacol substrate ( and ). Numerous studies on the KM and Vmax values of POD purified from different sources have been reported in the literature. In one study, the KM value for the oil palm (Elaeis guineensis jacq.) leaf was found as 3.96 mM, which is similar to the present study.[Citation11] In one study, Turkish black radish POD enzyme had a KM value of 0.036 mM and a Vmax value of 38728.17 EU/mL min for the guaiacol substrate.[Citation7] The KM and Vmax values of POD purified from Turkish black radish (Raphanus sativus L.) were found to be 24.88 mM and 3.23 EU/mL min, respectively, for the guaiacol substrate.[Citation8] Sweet gourd POD had a Vmax value of 15,500 EU/mL min and KM value of 17.1 mM for the same substrate.[Citation13] The KM value of POD purified from cauliflower (Brassica oleracea L.) buds was found to be 141.64 mM, and the Vmax was found to be 7500 EU/mL min.[Citation19] Black cabbage (Brassica oleracea var. acephala) POD had a KM value of 5.5 mM.[Citation31]
FIGURE 6 Lineweaver-Burk plot for POD purified from white cabbage (Brassica oleracea var. capitata f. alba).
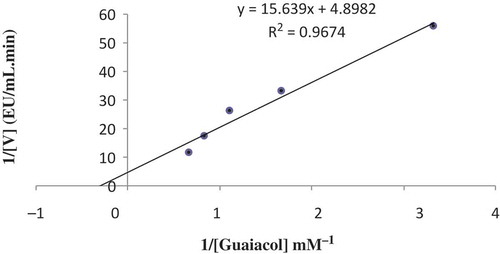
The relationship of Ki and IC50 values for a given compound varies, depending on the inhibitory mechanism and the assay conditions, and there is no significant relationship between these two values. Even so, the Ki and IC50 values are the most suitable parameters to show the effect of inhibition. These values determine the inhibition effect of a compound on a biochemical function. In order to ascertain the inhibitory effect of 4-amino benzohydrazide, the Ki and IC50 values were determined. For this purpose, the purified POD activity was measured in the presence of five different concentrations of 4-aminobenzohydrazide. A control sample without 4-amino benzohydrazide was used to determine 100% activity, and an activity-[4-amino benzohydrazide] plot was drawn. The IC50 value was determined from the graph (). To determine the Ki constant, activity measurements were performed at five different guaiacol concentrations and three different inhibitor concentrations with the purified POD. Lineweaver-Burk graphs (1/V-1/[S]; ) were obtained for the 4-amino benzohydrazide. The IC50 and Ki values for 4-amino benzohydrazide were calculated to be 0.517 mM () and 0.994 ± 0.453 mM () for white cabbage (Brassica oleracea var. capitata f. alba) POD. Furthermore, the inhibition type was determined for 4-amino benzohydrazide and non-competitive inhibition was observed.
CONCLUSION
POD was purified for the first time from white cabbage (Brassica oleracea var. capitata f. rubra) as a new source by affinity chromatography and some biochemical properties were determined. As can be seen from the results, the activity and purification factors of the purified POD are as good as or better than many reported previously in the literature.
REFERENCES
- Verhoeven, D.T.; Goldbohm, R.A.; Poppel, G.; Verhagen, H.; Brandt, P.A. Epidemiological studies on brassica vegetables and cancer risk. Cancer Epidemiology Biomarkers and Prevention 1996, 5, 733–748.
- Podsedek, A. Natural antioxidants and antioxidant capacity of Brassica vegetables: A review. Food Science Technology 2005, 40, 1–11.
- Nosek, M.; Surowka, E.; Cebula, S.; Libik, A.; Goraj, S.; Miszalski, A.K.Z. Distribution pattern of antioxidants in white cabbage heads (Brassica oleracea L. var. capitata f. alba). Acta Physiologiae Plantarum 2011, 33, 2125–2134.
- Singh, J.; Upadhyay, A.K.; Bahadur, A.; Singh, B.; Singh, K.P.; Rai, M. Antioxidant phytochemicals in cabbage (Brassica oleracea L. var. capitata). Scientia Horticulturae 2006, 108, 233–237.
- Singh, J.; Upadhyay, A.K.; Prasad, K.; Bahadur, A.; Rai, M. Variability of carotenes, vitamin C, E, and phenolics in Brassica vegetables. Journal of Food Composition and Analysis 2007, 20, 106–112.
- Šamec, D.; Bogović, M.; Vincek, D.; Martinčić, J.; Salopek-Sondi, B. Assessing the authenticity of the white cabbage (Brassica oleracea var. capitata f. alba) cv. ‘Varaždinski’ by molecular and phytochemical markers. Food Research International 2014, 60, 266–272.
- Şişecioğlu, M.; Gülçin, İ.; Çankaya, M.; Atasever, A.; Şehitoğlu, M.H.; Budak Kaya, H.; Özdemir, H. Purification and characterization of peroxidase from Turkish black radish (Raphanus sativus L.). Journal of Medicinal Plants Research 2010, 12, 1187–1196.
- Kalın, R.; Atasever, A.; Özdemir, H. The single-step purification of peroxidase by 4-aminobenzohydrazide from Turkish blackradish (Raphanus sativus L.) and turnip (Brassica rapa L.) roots. Food Chemistry 2014, 150, 335–340.
- Veitch, N.C. Horseradish peroxidase: A modern view of a classic enzyme. Phytochemistry 2004, 65, 249–259.
- Pandey, V.P.; Singh, S.; Singh, R.; Dwivedi, U.N. Purification and characterization of peroxidase from papaya (Carica papaya) fruit. Applied Biochemistry and Biotechnology 2012, 167, 367–376.
- Sakharov, I.Y.; Leon, J.C.; Areza, J.C.; Galaev, I.Y. Purification and stability of peroxidase of African oil palm elaise guineensi. Bioseparation 2000, 9, 125–132.
- Leon, J.C.; Alpeeva, I.S.; Chubar, T.A.; Galaev, I.Y.; Csorgei, E.; Sakharov, I.Y. Purification and substrate specificity of peroxidase from sweet potato tubers. Plant Science 2002, 163, 1011–1019.
- Köksal, E.; Bursal, E.; Aggul, A.G.; Gulcin, I. Purification and characterization of peroxidase from sweet gourd (Cucurbita moschata Lam. Poiret). International Journal of Food Properties 2012, 15, 1110–1119.
- Bursal, E. Kinetic properties of peroxidase enzyme from chard (Beta vulgaris Subspecies cicla) leaves. International Journal of Food Properties 2013, 16, 1293–1303.
- Saraiva, J.A.; Nunes, C.S.; Coimbra, M.A. Purification and characterization of olive (Olea europaea L.) peroxidase—Evidence for the occurrence of a pectin binding peroxidase. Food Chemistry 2007, 101, 1571–1579.
- Ajila, C.M.; Prasada Rao, U.J.S. Purification and characterization of black gram (Vigna mungo) husk peroxidase. Journal of Molecular Catalysis B: Enzymatic 2009, 60, 36–44.
- Pandey, V.P.; Dwivedi, U.N. Purification and characterization of peroxidase from Leucaena leucocephala, a tree legume. Journal of Molecular Catalysis B: Enzymatic 2011, 68, 168–173.
- Kumar, R.; Singh, K.A.; Singh, V.K.; Jagannadham, M.V. Biochemical characterization of a peroxidase isolated from caribbean plant (Euphorbia cotinifolia). Process Biochemistry 2011, 46, 1350–1357.
- Köksal, E.; Gülçin, İ. Purification and characterization of peroxidase from cauliflower (Brassica oleracea L.) buds. Protein and Peptide Letters 2008, 15, 320–326.
- Suzuki, T.; Honda, Y.; Mukasa, Y.; Kim, S. Characterization of peroxidase in buckwheat seed. Phytochemistry 2006, 67, 219–224.
- Deepa, S.S.; Arumughan, C. Purification and characterization of soluble peroxidase from oil palm (Elaeis guineensis Jacq.) leaf. Phytochemistry 2002, 61, 503–511.
- Triplett, B.A.; Mellon, J.E. Purification and characterization of anionic peroxidases from cotton (Gossypium hirsutum). Plant Science 1992, 81, 147–154.
- Kettle, A.J.; Gedye, C.A.; Winterbourn, C.C. Mechanism of inactivation of myeloperoxidase by 4 amino benzoic acid hydrazide. Biochemical Journal 1997, 321, 503–508.
- Atasever, A.; Özdemir, H.; Gülçin, İ.; Küfrevioğlu, Ö.İ. One-step purification of lactoperoxidase from bovine milk by affinity chromatography. Food Chemistry 2013, 136, 864–870.
- Laemmli, D.K. Clevage of structual proteins during in assembly of the head of bacteriophage T4. Nature 1970, 227, 680–683.
- Chevallet, M.; Luche, S.; Rabilloud, T. Silver staining of proteins in polyacrylamide gels. Nature Protocols 2006, 1, 1852–1858.
- Bradford, M.M. A rapid and sensitive method for the quantitation of microgram quantities of proteins utilizing the principal of protein dye binding. Analytical Biochemistry 1976, 72, 248–254.
- Doerge, D.R.; Divi, R.L.; Churchwell, M.I. Identification of the colored guaiacol oxidation product produced by peroxidases. Analytical Biochemistry 1997, 250, 10–17.
- Lineweaver, H.; Burk, D.J. The determination of enzyme dissociation constants. Journal of the American Chemical Society 1934, 56, 658–666.
- Köksal, E. Peroxidase from leaves of spinach (Spinacia oleracea) partial purification and some biochemical properties. International Journal of Pharmacology 2011, 7, 135–139.
- Gülçin, İ.; Yıldırım, A. Purification and characterization of peroxidase from Brassica oleracea var. acephal. Asian Journal of Chemistry 2005, 17, 2175–2183.
- Lavery, C.B.; Macinnis, M.C.; Macdonald, M.J.; Williams, J.B.; Spencer, C.A.; Burke, A.A., et al. Purification of peroxidase from horseradish (Armoracia rusticana) root. Journal of Agriculture and Food Chemistry 2010, 58, 8471–8476.
- Civello, P.M.; Martinez, G.A.; Chaves, A.R.; Anon, M.C. Peroxidase from strawberry fruit (Fragaria ananassa-Duch) partial-purification and determination of some properties. Journal of Agricultural and Food Chemistry 1995, 43, 2596–2601.
- Jen, J.J.; Seo, A.; Flurkey, W.H. Tomato peroxidases purification via hydrophobic chromatography. Journal of Food Science 1980, 45, 60–63.
- Sessa, D.J.; Anderson, R.L. Soybean peroxidases: Purification and some properties. Food Chemistry 1981, 29, 960–965.
- Ito, H.; Hiraoka, N.; Ohbayashi, A.; Ohashi, Y. Purification and chracterization of rice peroxidases. Agricultural and Biological Chemistry 1991, 55, 2445–2454.
- Chen, M.; Yao, S.; Zhang, H.; Liang, X. Purification and characterization of a versatile peroxidase from edible mushroom Pleurotus eryngii. Chinese Journal of Chemical Engineering 2010, 18, 824–829.
- Rodrigo, C.; Rodriga, M.; Alvarruiz, A.; Frigola, A. Thermal inactivation at high temperatures and regeneration of green asparagus peroxidase. Journal of Food Protection 1996, 19, 1065–1071.