Abstract
Eggplant has a very limited shelf life and, like other vegetables, is susceptible to different types of damage during and after harvest operations. Besides, eggplant is inhomogeneous considering its inner construction point of view. It is therefore important to specify how storage might affect their mechanical properties and how they vary in morphology. In this work, eggplants were divided into three portions along the longitudinal axis and their textural properties were separately investigated using different types of test over a 10-day period of storage. The results showed that the tension strength, rupture force and Young’s modulus of skin tissue decreased with increasing the length of storage period, and they were generally different in the different portions of the fruit that were sampled. The values of Young’s modulus and rupture force of pulp tissue taken from compression tests decreased from 1.466 to 0.821 MPa and 20.70 to 17.66 N for upper section, 0.637 to 0.536 MPa and 15.33 to 13.13 N for middle section and 0.518 to 0.422 MPa and 14.19 to 12.19 N for bottom section, respectively, with the increase in storage period. Similarly, as the samples were stored longer, the Young’s modulus and rupture force of their combined skin and pulp tissues, obtained from penetration tests, decreased from 3.01 to 2.02 MPa and 31.21 to 24.95 N for upper section, 2.59 to 1.66 MPa and 28.64 to 21.66 N for middle section and 1.91 to 1.15 MPa and 23.18 to 17.37 N for bottom section, respectively.
INTRODUCTION
The consumption of eggplant (Solanum melongena L.) is steadily increasing because of greater awareness of the health beneficial effects associated with increased consumption of fruits and vegetables.[Citation1] It is consumed as fresh and a whole product or, more recently, as a minimally processed vegetable, a rising commercial area in the fresh-cut industry.[Citation2] Nevertheless, shelf life of this popular crop in ideal condition for storage is about 10 days[Citation3] so any damage leads to faster deterioration.
Generally, eggplants are harvested carefully by human labor when they have reached a marketable size. The short harvesting season results in a concentrated work in a short period of time and the lack of available labor consequently press the eggplant growers to limit their cultivation area in the first place. Furthermore, the harvesting operation of eggplants is complicated and time-consuming[Citation4] so that Canakci and Akinci[Citation5] reported that energy requirement for eggplant harvesting is significantly greater than that for tomatoes, peppers, and cucumbers. To solve these problems, human workers can be replaced by artificial intelligence robots. A major problem associated with mechanical harvesting is the mechanical stresses[Citation6] which are imposed on fruit during the gripping process by robot fingers.[Citation7] Therefore, measuring the mechanical parameters of eggplant is essential for reducing mechanical damage when grasped by robot’s gripper. Knowledge in the rheological and textural attributes of biomaterials is also necessary for genetic improvement,[Citation8] design and development of specific equipment and structures of post-harvest handling, packaging, peelers, fresh-cut processing, and assessing final product quality.[Citation9Citation10]
During eggplant handling and transportation after harvesting, two main forces are encountered: compression force and puncture force. By and large, the compression force and the puncture force are experienced by the whole fruit and by a specific point on the fruit, respectively.[Citation8] Mechanical injuries and excessive compression are unpleasant for both fresh fruits and vegetables market and industrial processing. These damages cause both immediate and subsequent physiological responses in wounded and adjacent tissues, leading to complex physiological, metabolic, and enzymatic changes,[Citation11] such as increased respiration at the injured sites, increasing general deterioration, excessive tissue softening, browning of the tissue, wilting, discoloration, development of off-flavor, texture breakdown and, as a consequence, decreasing the visual appearance aspects, their commercial value, and the quality of final products.[Citation3Citation7Citation12] Hence, an understanding of the mechanical characteristics of fruits and vegetables can decrease the effects of unwanted mechanical loading, quantity of mass wastage and energy consumption during processing operation like peeling stage.[Citation9]
The mechanical behaviors of biological materials are related to the histological, anatomical, and biochemical specifications of the living cells.[Citation13] Most foodstuffs have a mechanically complex and heterogeneous structure and are frequently anisotropic as well.[Citation14] Thus, the mechanical stresses are distributed inhomogeneous in plant organs.[Citation15] The morphological characteristics of eggplant fruit vary significantly with the type of the eggplant cultivar.[Citation1] The teardrop and elongate-shaped types are commonly grown commercially around the world. Most of these varieties can be divided into three portions along the central axis, namely, the calyx section, the body section, and the blossom end section.[Citation16] The distribution of principal components including exocarp (skin) thickness, mesocarp (flesh), endocarp (seed cavity), placenta (internal walls where seeds are attached), and seed in the structure of these portions is different and can affect on their mechanical properties. On the other hand, the fruit’s mechanical properties vary temporally mainly due to how fast or slow its metabolic changes occur. According to this it is important to assess the magnitude of changes in the variables related to mechanical strength during the fruit storage period.[Citation11]
There were several studies in the literature focusing on characterizing basic mechanical properties as a function of loading location and orientation for some plant materials such as peach,[Citation11] cucumber,[Citation17] pear,[Citation18] carrot,[Citation19] potato,[Citation20] pineapple,[Citation21] pomegranate,[Citation22] and tomato.[Citation23] It is revealed from the literature that so far, the information related to the effect of storage and loading location on mechanical responses of eggplant tissues is lacking. Therefore, the specific objectives of this study were to: (1) measure the mechanical properties of eggplant skin and pulp under tensile, penetration, and compression tests and how they vary among three portions along the central axis of the fruit; (2) quantify the cutting features of stem; and (3) evaluate the effect of storage time on the mechanical properties of eggplant tissue.
MATERIALS AND METHODS
Plant Material and Storage Conditions
Solanum melongena eggplants cv. Siah-e-Mashhad were grown in an experimental farm at Ferdowsi University of Mashhad, Iran. Eggplant fruits were hand-harvested in mid-August 2014 from different plants and various locations of farm, after reaching a mass of 100–150 g and length between 15 and 20 cm and before complete seed development. Fresh eggplants with good quality attributes (dark purple color, smooth, glossy skin, a fresh green calyx, and free from any visual defect) were selected for this research. Upon arrival at the laboratory, the fruits were again inspected to ensure that they were uniform, undamaged and not attacked by pests. Each fruit was weighed, and diameter of the largest cross-section perpendicular to the blossom end-calyx end axis (intermediate diameter), and the distance between the blossom and calyx ends (longitudinal diameter) were measured from images taken with a digital camera (SONY DSC-W35) by applying ImageJ 1.46d software. The physical data of the fruits were: 134 ± 12 g (mass), 181 ± 13 mm (longitudinal diameter), and 49 ± 5 mm (intermediate diameter). Fruits were hand rinsed under a stream of tap water at a temperature of 4°C, to remove field heat, soil particles and to reduce microbial populations on the surface, then rinsed with double distilled water and gently blotted with a paper towel to remove the water.[Citation24] In total, 129 eggplants were used for this experiments, so that 43 samples were used for the tests on the day they were harvested (0 day) and remaining samples were stored in an environmental chamber in optimal conditions (~11°C, ~90% relative humidity [RH]) recommended by Cantwell and Suslow[Citation25] for 5 and 10 days to attain the different storage times.
Determination of Mechanical Properties
To determine the variation of mechanical attributes from the top to the bottom, the eggplant was separated into three sections along the longitudinal axis of the fruit and labeled as upper, middle, and bottom thirds (). All mechanical tests were carried out using a Texture Analyzer (H5KS-1929, SDL ATLAS, UK) and data were acquired and processed by the software Qmat 4.55-Dongle: 4959. At each storage time, before starting a trial samples were allowed to equilibrate to room temperature (20 ± 1°C, 53–55% RH) for approximately 1 h and all tests were conducted in laboratory under the same conditions.[Citation26] As suggested by ASABE Standards,[Citation26] all the mechanical tests were replicated 20 times, thus the mean values with the corresponding standard deviations were estimated, as listed in related tables.
Moisture Content Determination
At each storage day (0, 5, and 10 d) three samples were selected randomly for measurement of moisture content and the remaining were used for mechanical analyses. First, each eggplant was cut into three portions (upper, middle, bottom) with a sharp knife vertical to the longitudinal axis. In the next step, about 5 and 10 g samples of peel and pulp, respectively, were taken and separately kept in a vacuum oven at 70°C and 2.5 kPa for 12 h,[Citation27] and the water content was determined from the difference of initial and final weight of the specimen. The moisture content of stem was also determined by air convection oven drying at 103°C until constant weight was reached.[Citation28] The mean of three replications was reported.
Tensile Testing
Rectangular skin blocks were prepared by placing slicer rings with two parallel razor blades mounted on metal block around the larger circumference of each three portions to ensure constant dimensions (10 mm in width and 50 mm in length). Thereafter, the adhering pulp was removed mechanically by a razor-sharp and hand-scraping from skin layer. The specimen size was decided based on preliminary studies and in reference to Toole et al.[Citation29] Peel thickness was measured using a micrometer with 0.001 mm accuracy. Optical microscope was used immediately for inspecting the absence of small cracks, before mechanical extension tests. The texture analyzer was equipped with suitable specimen holders for one-dimensional tension testing. Each segment was held by standard upper and lower tensile clamps equipped with a thin layer of rubber to avoid slipping during stretching.[Citation12] The clamps were adjusted each time to precisely 30 mm apart, leaving roughly 10 mm of sample clamped at each end. All specimens were stretched at the rate of 10 mm/min[Citation10] by movement of the upper clamp, and each specimen was visually checked during the test; if failure occurred near the clamp the experimentation was terminated and its data were rejected. The force versus deformation data were recorded and rupture force (the maximum force required to propagate the crack), Young’s modulus (slope of the initial linear portion of curve), and tensile strength (dividing the rupture force by the cross-sectional area of the first specimen) were derived.
Compression Testing
Compression tests were performed on the samples provided from the same fruits that were used in the tension test. The pulp samples used in unidirectional compression test were cut by a cork borer with a 25 mm inside diameter. The cylindrical borer was inserted into the eggplant by carefully pushing and rotating it along the longitudinal axis from the blossom end to the calyx. For each section, cylindrical specimen with a height of 25 mm was taken out from the central region of eggplants by using a double-blade cutting tool (). The specimen size was determined based on the literature[Citation30] and our preliminary study on the effect of specimen size on the mechanical properties measurement because the height of the specimen should be equal to or less than its diameter, otherwise reproducibility and reliability of compression tests will decrease due to buckling.[Citation31] The sample was placed on the center of the stationary plate before browning phenomenon and pressed by the moving parallel flat stainless steel circular plate at a loading speed of 10 mm/min (see ).[Citation8Citation26] As the compression began and progressed, a force-displacement curve was plotted automatically in relation to the response of each specimen to compression. Subsequently, rupture force (load peak), deformation at rupture force (deformation at peak), Young’s modulus (the slope of the straight-line region of curve), and toughness (the area under the whole diagram to the point of rupture) were extracted from recorded curve.
Penetration Testing
To design the puncture probe, the stem diameters of eggplants were measured directly after harvest and the average diameter was obtained.[Citation12] As a result of these measurements, a stainless steel plunger with the flat end (10.6 mm diameter) attached to the load cell was used to penetrate the fruit (with its peel) at a crosshead speed of 10 mm/min.[Citation8] In order to do the test, eggplants were first cut into two halves along the longitudinal axis. Each slice was positioned intently under the probe and held lightly between two fingers until probe made perpendicular contact to the surface at the center of each three portions, and then the punch probe was swiftly released. Bio-yield force (the first drop in force on the curve prior to reaching the rupture), deformation at bio-yield, Young’s modulus (stiffness), rupture force, deformation at rupture, and toughness were obtained from the force-distance curve.
Cutting Test
A stainless steel cutting probe with sharpened edge of 30° included angle and 1.5 mm thickness was made and installed to the movable clamp. The stem specimen was placed on the fixture and cut along its thickness at a steady velocity of 20 mm/min[Citation9] until it fractured, and the force-distance data were recorded by computer. The peak force was identified with the help of plot and the area under the curve was measured as the cutting energy. Subsequently, the cutting parameters of stem such as ultimate cutting stress and specific energy were calculated according to Tavakoli et al.[Citation28]
Statistical Evaluation of Data
All statistical analyses were performed using SPSS version 20.0 software (IBM SPSS Statistics, IBM Corporation, Somers, NY, USA). Analysis of variance (ANOVA) and Duncan test (p < 0.05) were conducted to compare mechanical changes in different sections of the fruit among the storage day.
RESULTS AND DISCUSSION
Tension Mechanical Properties
The main data were collected during axial tension tests are presented in . As can be seen from , the rupture force, Young’s modulus and tensile strength of eggplant skin in all three sections had decreasing trend with increase in the number of days of storage. The average values of rupture force, Young’s modulus and tensile strength decreased by 32.11, 25.14, and 23.96% for upper section, 24.22, 23.02, and 18.68% for middle section, and 24.49, 18.63, and 19.92% for bottom section, respectively, over the storage period of 10 days. This indicated that the peel of the eggplant was more prone to mechanical damage at the end of storage than at the beginning of storage. These results demonstrate that the tension characteristics of upper section were dropped with greater intensity in comparison to other sections over 10 days of storage. This could be attributed to the fact that water exchange in eggplant occurs mostly through the calyx,[Citation32] and therefore, it is possible that fruit skin shriveling occurs more rapidly in calyx area during postharvest storage ().
TABLE 1 The results of tension test of eggplant skin from different sections of the fruit during storage
FIGURE 2 A: Changes in skin (S) and pulp (P) moisture content from different sections of the fruit at different storage days. Error bars show one standard deviation from the mean; B: Changes in skin thickness from different sections of the fruit at different storage days. Different letters on bars indicate statistically significant differences (p < 0.05).
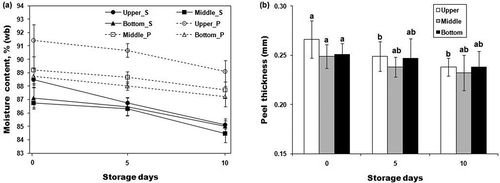
Until now, relatively little information is available on the mechanical properties of eggplant tissues, so it is difficult to make comparisons. However, similar studies have reported the effect of storage time on the skin tensile properties: Harker and Hallett[Citation33] and Singh and Reddy[Citation10] for kiwifruit and orange, respectively. Harker and Hallett[Citation33] reported the tension strength of kiwifruit skin vary from about 190 to 50 N/cm2. Singh and Reddy[Citation10] noted the storage time has not a significant effect on the tensile strength, modulus of elasticity and rupture force of orange peel tissue, and the tensile strength, modulus of elasticity and rupture force vary from 0.173 to 0.138 MPa, 1.57 to 1.03 MPa, and 15.6 to 12.7 N, respectively, over the storage period of 10 days. The explanation for this behavior may be related to the fact that, during storage, adhesion between neighboring cells diminished so that most cells separated from each other without breaking.[Citation33] The other reason can be associated with moisture losses during storage (). Skins with higher moisture content are more extensibility and flexibility as a result of reducing friction between adjacent cellulose micro fibrils, so the drier skins do not extend as far as humid skins and fracture occurs with small amount of force.[Citation34] Moreover, as reflected in , skin thickness (as a factor affecting on skin mechanical properties) decreased with increasing storage duration. The decrease in skin thickness may be attributed to water loss from fruit peel as storage period progressed. However, ANOVA results showed that skin thickness had no significant effect (p > 0.05) on studied properties.
On days 0, 5, and 10, the mean values of rupture force and Young’s modulus in the upper area were higher than those of the other sections and the lowest values was observed in middle section. On day 0, the upper and middle sections with 5.51 and 5.14 MPa had the highest and lowest values of tensile strength, respectively. The experimental data showed that there were no noticeable differences in tensile strength between all studied sections in the middle of the storage (day 5) and end of storage period (day 10). Investigations of Thompson et al.[Citation17] showed that the puncture force of Calypso cucumber skin decrease from stem section to blossom (from 15.8 to 14.9 N) section. Considering the failure modes of fruit tissues are different during penetration and tension but these results demonstrated that the mechanical behavior of skin in various loading locations can be different and varied. The reason of these discrepancies can be explained as follows: The biomechanical properties of skin could be affected by thickness, calcium content and pectin value, cell wall structure and component, quantity and volume of intercellular spaces, etc.[Citation35] As the thickness of skin changes in different places of the product, different values of mechanical properties are expected in different places.[Citation9] The calcium effect can be explained by the formation of a calcium bridge between groups of pectin chains, especially homogalacturnan.[Citation35] Therefore, because several important factors play significant role in eggplant skin’s biomechanical behavior, the determination of main reason for these differences is too complicated. To obtain a complete comprehension in histological view of fracture, a comparison of micro fibrils arrangements and cell geometry along the longitudinal axis of fruit skin using an electronic microscope is indispensable.
Compression Mechanical Properties
The averages and standard deviations of the compression test are tabulated in . The results in this table clearly show that the all investigated parameters, except deformation at rupture point and toughness, decreased monotonically with the increasing of storage time. The compression rupture force, deformation at rupture force, toughness, and Young’s modulus of eggplant pulp ranged from 20.70 to 17.66 N, 11.42 to 14.82 mm, 0.113 to 0.146 N∙m, and 1.466 to 0.821 MPa for the upper section; from 15.33 to 13.13 N, 11.50 to 15.11 mm, 0.092 to 0.118 N∙m, and 0.637 to 0.536 MPa for the middle section; from 14.19 to 12.19 N, 11.75 to 15.50 mm, 0.083 to 0.106 N∙m, and 0.518 to 0.422 MPa for the bottom section, respectively, as the storage time increased from day 0 to day 10. This indicated that the rigidity of the cellular structures had diminished over 10 days of storage due to loss of moisture through transpiration, as well as enzymatic changes, and thus the fruit structure became soft at the end of storage.[Citation36] In fact, hemicelluloses and pectin become more soluble, which resulted in disruption and loosening of the cell walls.[Citation37] As depicts, there were 2.52, 1.67, and 1.69% decreases in the moisture content of upper, middle, and bottom sections of the pulp, respectively, after 10 days of storage, but the decreases were not statistically significant.
TABLE 2 The results of compression test of eggplant pulp from different sections of the fruit during storage
The behavior observed in this study was similar to that found for the pear and apple,[Citation38] orange,[Citation10] potato,[Citation30] and peach.[Citation11] The results of the plate compression test illustrated that, on days 0, 5, and 10, the mean values of rupture force, toughness, and Young’s modulus in the upper section were higher than those of the other sections and the lowest values was observed in bottom section. In contrast, deformation at rupture force increased slightly from the upper to the bottom thirds in each storage time (). The results show that there is less rigidity in the bottom section as compared to the other sections. The different response exhibited by the eggplant tissue as a result of loading location demonstrated that it is a non-homogeneous fruit. The non-homogeneous behavior of the eggplant tissue may be attributed to the shape and arrangement of parenchymal cells and other morphological components.[Citation11] Similarly, Montero-Calderón et al.[Citation21] reported that hardness and hardness work of pineapple flesh decreased from the top to the bottom of the fruit. In addition, Wang[Citation18] showed the failure strain, failure stress, failure energy, and Young’s modulus of pear flesh change from the top (stem) to the bottom (calyx).
Penetration Mechanical Properties
shows the change of the textural parameters obtained by a puncture test. These parameters are the response of the skin and the pulp at a tested point. As can be observed from this table, in all three sections, Young’s modulus, rupture force, and bio-yield force considerably decreased with the increase of storage time. This implies the softening of the fruit. In brief, this phenomenon can be explained by the fact that the cell walls of fruit tissue are rich in protopectin at the day of harvest; the insoluble protopectin content of tissue cell walls gradually decreases with increasing storage duration while the soluble pectin content increases, so the fruit tissue gradually become soft during storage.[Citation23] It is necessary here to mention that the bio-yield point is the point that indicates the initial cell under the skin rupture before the skin is broken.[Citation8] Young’s modulus, rupture force and bio-yield force decreased by 32.89, 20.06, and 16.26% for the upper section, 35.91, 24.37, and 22.85% for the middle section, and 39.79, 25.06, and 25.36% for the bottom section, respectively, as the length of storage time increased. While deformation at rupture force, deformation at bio-yield force, and toughness progressively increased by 13.11, 20.63, and 16.56% for the upper section, 15.65, 19.49, and 25.85% for the middle section, and 11.68, 17.86, and 36.15% for the bottom section, respectively, following storage. The energy of absorption (toughness) indicates the energy required to change the shape of fruit. Therefore, during storage, the fruit could be deformed more under the same force; as the fruit became softer.[Citation8] This result agrees with the work of Singh and Reddy,[Citation10] which showed that the puncture force of the orange fruit decreased during storage. At all storage times, the rupture force, bio-yield force, Young’s modulus and toughness decreased from the upper to the bottom third and on contrary, deformation at rupture force and deformation at bio-yield force increased from the upper to the bottom third of the eggplant. Results indicated that the upper section was the hardest and most rigid in response to the puncture force when comparing to middle and bottom sections (). In this regard, Mansouri et al.[Citation22] reported the puncture energy and firmness of various positions of pomegranate fruit were severely different because of variation in thickness of the peel at various positions. They found that the mechanical properties of the bottom portion were more than the top and middle sections, whereas the middle and top portions had approximately equal values. In other similar research, carrot root was cut into three sections, cross-sectionally, i.e., the base side, the central part, and the top, and exposed to a penetration test by Budrewicz et al.[Citation19] They understood that puncture force and firmness vary among fruit pieces from different sections of the fruit. Likewise, Sadowska et al.[Citation20] showed that puncture force and energy values of potato were usually higher near the stem end of the tuber as compared to other locations (center and bud end) tested.
TABLE 3 The results of penetration test from different sections of the fruit during storage
Cutting Test
The peak cutting force, cutting energy, ultimate cutting stress, and specific energy decreased dramatically with increase in storage time. The reduction ratio of peak cutting force, cutting energy, ultimate cutting stress, and specific energy in the course of storage were 21.87, 43.10, 21.67, and 43.26%, respectively (see ). This behavior can probably be connected to the viscous damping effect of moisture,[Citation28] whereas moisture content of the stem decreased from 85.96 to 75.95% (wet basis) with increase in storage time.
TABLE 4 Cutting mechanical parameters of eggplant stem at different storage days
CONCLUSION
In our study, the mechanical properties of eggplant fruit Siah-e-Mashhad cultivar at three loading positions (bottom, middle, and upper thirds) were assessed over the course of a 10-day storage period. Moisture content of skin and pulp and also skin thickness decreased over time. We found that the water content and skin thickness in the upper section are higher compared to those in the middle and bottom sections. The results obtained from the tensile, penetration, and compression tests demonstrated that textural parameters of eggplant tissues changed from the top to the bottom of the fruit. The average values of the peak cutting force and cutting energy of eggplant stem at the beginning of storage were around 1.3 and 2 times greater than that at the end of storage. Finally, it can be concluded that eggplant has different skin strength and tissue structure in its different sections. To the best of our knowledge, this is the first report of the mechanical properties of the eggplant. Based on the obtained results, recommendations can be made for the better grippers design for robotic eggplant harvesters in a way that the optimal region on the fruit surface can be grasped by robots fingers. Additionally, the findings of this study can be seen as considerations in designing of eggplants’ peeling and packaging systems. Future research needs to be conducted to investigate the effects of loading speed and shape of the probe on the textural properties of eggplants.
ACKNOWLEDGMENTS
The authors would like to express their gratitude to Mrs. Fatemeh Sadeghi and Mrs. Narges Ghanei for their assistance during the measurements.
REFERENCES
- Singh, A.P.; Luthria, D.; Wilson, T.; Vorsa, N.; Singh, V.; Banuelos, G.S.; Pasakdee, S. Polyphenols Content and Antioxidant Capacity of Eggplant Pulp. Food Chemistry 2009, 114, 955–961.
- Barbagallo, R.N.; Chisari, M.; Caputa, G. Effects of Calcium Citrate and Ascorbate As Inhibitors of Browning and Softening in Minimally Processed “Birgah” Eggplants. Postharvest Biology and Technology 2012, 73, 107–114.
- Hu, W.; Jiang, A.; Tian, M.; Liu, C.; Wang, Y. Effect of Ethanol Treatment on Physiological and Quality Attributes of Fresh-Cut Eggplant. Journal of the Science of Food and Agriculture 2010, 90, 1323–1326.
- Hayashi, S.; Ganno, K.; Ishii, Y.; Tanaka, I. Robotic Harvesting System for Eggplants. Japan Agricultural Research Quarterly 2002, 36, 163–168.
- Canakci, M.; Akinci, I. Energy Use Pattern Analyses of Greenhouse Vegetable Production. Energy 2006, 31, 1243–1256.
- Li, Z.; Li, P.; Liu, J. Physical and Mechanical Properties of Tomato Fruits As Related to Robot’s Harvesting. Journal of Food Engineering 2011, 103, 170–178.
- Li, Z.; Li, P.; Liu, J. Effect of Tomato Internal Structure on Its Mechanical Properties and Degree of Mechanical Damage. African Journal of Biotechnology 2010, 9, 1816–1826.
- Sirisomboon, P.; Tanaka, M.; Kojima, T. Evaluation of Tomato Textural Mechanical Properties. Journal of Food Engineering 2012, 111, 618–624.
- Emadi, B.; Kosse, V.; Yarlagadda, P.K.D.V. Mechanical Properties of Pumpkin. International Journal of Food Properties 2005, 8, 277–287.
- Singh, K.K.; Reddy, B.S. Post-Harvest Physico-Mechanical Properties of Orange Peel and Fruit. Journal of Food Engineering 2006, 73, 112–120.
- Pérez-López, A.; Chávez-Franco, S.H.; Villaseñor-Perea, C.A.; Espinosa-Solares, T.; Hernández-Gómez, L.H.; Lobato-Calleros, C. Respiration Rate and Mechanical Properties of Peach Fruit During Storage at Three Maturity Stages. Journal of Food Engineering 2014, 142, 111–117.
- Allende, A.; Desmet, M.; Vanstreels, E.; Verlinden, B.E.; Nicolaï, B.M. Micromechanical and Geometrical Properties of Tomato Skin Related to Differences in Puncture Injury Susceptibility. Postharvest Biology and Technology 2004, 34, 131–141.
- Varela, P.; Salvador, A.; Fiszman, S. Changes in Apple Tissue with Storage Time: Rheological, Textural, and Microstructural Analyses. Journal of Food Engineering 2007, 78, 622–629.
- Li, Z.; Yang, H.; Li, P.; Liu, J.; Wang, J.; Xu, Y. Fruit Biomechanics Based on Anatomy: A Review. International Agrophysics 2013, 27, 97–106.
- Dan, H.; Kohyama, K. Characterization of Cucumber Cultivars by Mechanical Stress Distributions During the Compression Process. Japan Agricultural Research Quarterly 2007, 41, 115–121.
- Chong, V.K.; Kondo, N.; Ninomiya, K.; Nishi, T.; Monta, M.; Namba, K.; Zhang, Q. Features Extraction for Eggplant Fruit Grading System Using Machine Vision. Applied Engineering in Agriculture 2008, 24, 675–684.
- Thompson, R.L.; Fleming, H.P.; Hamann, D.D. Delineation of Puncture Forces for Exocarp and Mesocarp Tissues in Cucumber Fruit. Journal of Texture Studies 1992, 23, 169–184.
- Wang, J. Mechanical Properties of Pear As a Function of Location and Orientation. International Journal of Food Properties 2004, 7, 155–164.
- Budrewicz, G.; Majewska, K.; Borowska, E.J.; Zadernowski, R. Texture Characteristics of Selected Carrot Varieties for the Processing Industry. Polish Journal of Food Nutrition Sciences 2005, 55, 57–62.
- Sadowska, J.; Fornal, J.; Zgórska, K. The Distribution of Mechanical Resistance in Potato Tuber Tissues. Postharvest Biology and Technology 2008, 48, 70–76.
- Montero-Calderón, M.; Rojas-Graü, M.A.; Martín-Belloso, O. Mechanical and Chemical Properties of Gold Cultivar Pineapple Flesh (Ananas Comosus). European Food Research and Technology 2010, 230, 675–686.
- Mansouri, Y.S.; Khazaei, J.; Hassan-Beygi, S.R.; Mohtasebi, S.S. Post Harvest Characteristics of Pomegranate (Punica Granatum L.) Fruit. Cercetări Agronomice în Moldova 2011, 146, 5–16.
- Li, Z.; Li, P.; Yang, H.; Liu, J.; Xu, Y. Mechanical Properties of Tomato Exocarp, Mesocarp, and Locular Gel Tissues. Journal of Food Engineering 2012, 111, 82–91.
- Arivalagan, M.; Gangopadhyay, K.K.; Kumar, G.; Bhardwaj, R.; Prasad, T.V.; Sarkar, S.K.; Roy, A. Variability in Mineral Composition of Indian Eggplant (Solanum Melongena L.) Genotypes. Journal of Food Composition and Analysis 2012, 26, 173–176.
- Cantwell, M.; Suslow, T.V. Eggplant: Recommendations for Maintaining Postharvest Quality. http://postharvest.ucdavis.edu/pfvegetable/Eggplant/ (accessed August 12, 2014).
- ASABE. Standard S368.4: Compression Test of Food Materials of Convex Shape. In ASAE Standards; American Society of Agricultural and Biological Engineers: Chicago, IL, 2008.
- Wu, L.; Orikasa, T.; Tokuyasu, K.; Shiina, T.; Tagawa, A. Applicability of Vacuum-Dehydrofreezing Technique for the Long-Term Preservation of Fresh-Cut Eggplant: Effects of Process Conditions on the Quality Attributes of the Samples. Journal of Food Engineering 2009, 91, 560–565.
- Tavakoli, H.; Mohtasebi, S.S.; Jafari, A. Physical and Mechanical Properties of Wheat Straw As Influenced by Moisture Content. International Agrophysics 2009, 23, 175–181.
- Toole, G.A.; Parker, M.L.; Smith, A.C.; Waldron, K.W. Mechanical properties of lettuce. Journal of Materials Science 2000, 35, 3553–3559.
- Bentini, M.; Caprara, C.; Martelli, R. Physico-Mechanical Properties of Potato Tubers During Cold Storage. Biosystems Engineering 2009, 104, 25–32.
- Shaw, M.C.; Young, E. Rubber Elasticity and Fracture. Journal of Engineering Materials and Technology 1988, 110, 258–265.
- Massolo, J.F.; Concellón, A.; Chaves, A.R.; Vicente, A.R. 1-Methylcyclopropene (1-MCP) Delays Senescence, Maintains Quality and Reduces Browning of Non-Climacteric Eggplant (Solanum Melongena L.) Fruit. Postharvest Biology and Technology 2011, 59, 10–15.
- Harker, F.R.; Hallett, I.C. Physiological and Mechanical Properties of Kiwifruit Tissue Associated with Texture Change During Cool Storage. Journal of the American Society for Horticultural Science 1994, 119, 987–993.
- Bayat, F.; Rezvani, S.M. Effect of Harvesting Time and Moisture on Mechanical Properties of Garlic (Allium Sativum L.) Skin. Agricultural Engineering International: CIGR Journal 2012, 14, 161–167.
- Saei, H.; Mehdi Sharifani, M.; Dehghani, A.; Seifi, E.; Akbarpour, V. Description of Biomechanical Forces and Physiological Parameters of Fruit Cracking in Pomegranate. Scientia Horticulturea 2014, 178, 224–230.
- Cen, H.; Lu, R.; Mendoza, F.; Beaudry, R.M. Relationship of the Optical Absorption and Scattering Properties with Mechanical and Structural Properties of Apple Tissue. Postharvest Biology and Technology 2013, 85, 30–38.
- Arvanitoyannis, I.S.; Khah, E.M.; Christakou, E.C.; Bletsos, F.A. Effect of Grafting and Modified Atmosphere Packaging on Eggplant Quality Parameters During Storage. International Journal of Food Science and Technology 2005, 40, 311–322.
- Yurtlu, Y.B.; Erdoğan, D. Effect of Storage Time on Some Mechanical Properties and Bruise Susceptibility of Pears and Apples. Turkish Journal of Agriculture and Forestry 2005, 29, 469–482.