Abstract
Dielectric property at high microwave frequency region has been utilized for possible rapid detection and screening of different types of meat, especially for halal authentication. This investigation focused on both raw and sterilized (processed) beef, pork, and chicken samples. Dielectric response that consists of dielectric constant and dielectric loss factor was measured over the range of 0.5 to 50 GHz. All raw and sterilized meat samples could be differentiated by the dielectric values. Two distinct peaks were observed only for both raw and sterilized pork samples at the frequency around 7.43 and 31.19 GHz. These peaks can potentially be linked to compounds that exist only in pork such as DNA, microbes, enzyme, proteins, amino acids, and many others. Dielectric values for sterilized samples were lower than raw samples due to molecule structural changes that occurred in the samples. The dielectric results promise a great potential of utilizing dielectric properties as a rapid on-site detection approach prior to subsequent laborious analysis.
Introduction
Meat is defined as the edible flesh of animals for human consumption that is composed of muscle fibrecella or lean tissue, fat, and connective tissue. Raw meat quality can be affected in different ways by three major components of meat, namely fat, lean (myofibrillar component) and connective tissue.[Citation1] Subsequently, the quality of meat characterization becomes more complicated when it is subjected to the process of cooking, curing, or preservation in the food industry. For instance, when meat or meat products are heated, the solubility of these substances such as protein in water or in buffer solutions is reduced. Heat-treated meat produces coagulated protein that has an irregular three-dimensional network of polypeptide chains overlapping one another.[Citation2,Citation3] Meanwhile, using solvents can adversely change the meat chemical structure permanently.
Authentication and adulteration are among the important issues in the food industry and can lead to the major economic loss to the parties involved. In the case of meat and meat products, the identification and detection of the species of the origin of meat samples are vital for consumers in relation to fraudulent adulteration, dietary requirement, medical need for specific allergies, as well as religious reasons that prohibits consumption of certain products.[Citation4] For instance, Muslim consumers are prohibited to consume pork-based products and byproducts otherwise known as “haram.”[Citation5]
Many methods are available for determining the species of origin of components in a food product derived from animals, which include immunochemical,[Citation6,Citation7] electrophoretic,[Citation8,Citation9] and polymerase chain reaction (PCR).[Citation4,Citation10–Citation13] PCR is a widely used molecular biology method. It is especially good since the DNA can provide additional information due to the degeneracy of the genetic code and the presence of many non-coding regions. Normally, amplified DNA fragments need subsequent analysis to identify the species of origin. However, these methods sometimes suffer from unsatisfactory results due to the transformation of the food product that experienced structural and chemical changes during processing. Some of the currently existing methods are expensive, unsuitable for routine analysis, and complex to perform, especially for the “halal” or non-prohibited products for Muslim consumers’ authentication.[Citation14–Citation16] The overpriced analysis is probably due to the equipment price and cost of analysis that are laborious, tedious, and time consuming.
Continuous efforts have been made in finding alternative methods. Dielectric technique or electromagnetic radiation in a microwave (MW) range is a rapid, easy to use, and low-cost detection not only for food items, but also for non-food materials.[Citation17] The extensive applications of dielectric technique in the food industry have attracted a lot of interest from researchers to conduct studies on meat and meat products. Although dielectric is not a new technology, earlier work focused mostly on the dielectric properties characterization of a meat in the low range of radio frequency (RF) and not in the “halal” authentication.[Citation18–Citation22] Abidin et al. explored the use of dielectric technique for the detection of alcoholic content in a solution for halal authentication and found it to be promising.[Citation17Citation22] The aim of this work was to establish the suitability and feasibility of using the dielectric method over the MW frequency range of 0.5 GHz–50 GHz, i.e., higher frequency range region as a potential rapid on-site detection method and discrimination of meat as a whole, particularly for halal and non-halal meat. The characterization of dielectric response focused on three types of raw meat, namely beef, chicken, and pork.
Material and Methods
Theoretical Background of Debye Model
Dielectric permittivity, ε* is an intrinsic property that describes the interaction of materials in food with the electromagnetic energy. Debye relation of dielectric permittivity, ε*, is a frequency-dependent parameter that consists of two important parts, which are known as dielectric constant, ε′ (dimensionless)and dielectric loss factor, ε″ (dimensionless).[Citation36] The response of a material to electromagnetic radiation is related to its electrical permittivity, which can be represented by Eq. (1).
where j is defined as j = (–1)½
Dielectric constant, ε′, describes the ability of a material to store energy in response to an applied electric field.[Citation23] In other words, dielectric constant determines the amount of energy reflected from the product and transmitted into the product. Dielectric loss factor, ε″, describes the ability of a material to dissipate energy in response to an applied electric field that typically results in heat generation. The amount of thermal energy converted in the food is proportional to the value of the loss factor.[Citation24]
Based on Eq. (1), the dielectric function of Debye model is written as in Eq. (2), while the dielectric constant and dielectric loss factor are given by Eqs. (3) and (4), respectively.[Citation25]
where
ω = Angular frequency (ω=2πƒ) in rad/s;
ε′∞ = The real permittivity at very high frequency (dimensionless);
ε′s = The real static permittivity (dimensionless);
τ = Debye relaxation time;
ƒ = Frequency
The dynamic dielectric behavior of molecules can be analyzed by studying the relaxation mechanisms. Relaxation usually means the return of a perturbed system into equilibrium. Relaxation time, τ, is a measure of the molecules’ (dipole) mobility that exists in a material and acts as a parameter to characterize the relaxation process. It describes the time necessary for approximately 1/e (e = 2.718) of the dipoles to relax under the given conditions like the composition and the solution environment, in which relaxation time is strongly dependent on both conditions.[Citation26]
Samples and Preparation
In this work, three types of fresh raw meat with specified parts (beef loin, pork loin, and chicken breast) were purchased from a local butcher in Seri Kembangan, Malaysia. Beef and chicken represent halal meat samples that have undergone ritual (Islam) slaughtering process, while pork meat represents the haram sample. Excess visible fat, skin, and bones were removed from the meats.
The preparation of raw meat samples was divided into two parts. First, each different meat type (beef, pork, and chicken) was ground individually using a household blender (Model Pensonic PB-323) mill attachment for 1 min. Second, all meats were mixed evenly in a beaker prior to being ground into a mixture of minced meat. The mixed meat proportion was 1:1:1.Once all the samples were prepared, ground beef, pork, chicken, and a mixture of ground meat were subjected to the Performance Network Analyzer (PNA-X) for dielectric measurement.
The sterilized samples were then prepared by placing some amount of all types of the ground raw meats in 500 mL beakers individually. The top of the beakers were covered with aluminium foil that was pricked with tiny holes to allow ventilation of the samples. Then, the samples were placed inside an autoclave (SX-500 High-pressure Steam Sterilizer) and the sterilization proceeded at 121°C. After the sterilization process was completed, the samples were left to cool at room temperature (20–22°C) about 45 min before dielectric measurement was recorded.
Meat Samples Characterization—Moisture, Protein, and Fat Content Determination
The moisture content of the samples was determined by drying a known amount of each meat sample for 48 h using an oven at 100°C. Then, the weight loss was determined.[Citation27] Meanwhile, the content of protein in the meat samples was determined by using the Kjeldahl method[Citation28] through a Fully Automated Protein Determination System with Model of Foss Kjeltec™ 2400 combined with a Foss Digestion Unit. The extraction of the total fat in the meat samples was performed using Hydrolizing Unit 1047 in combination with Foss Model of Soxtex™ 2050 Auto Fat Extraction System.
Dielectric Properties Measurement
After preparing all the meat samples, the dielectric response was measured using Agilent[Citation29] N5245A PNA-X. The system was stabilized before any measurement was taken. This PNA-X system consists of a signal source, a receiver, a screen that displays both amplitude and phase properties, as well as software to analyze the data.
Open-ended probe technique was used for the dielectric measurement work. The probes used were performance probes that are available in the Agilent 85070E probe kit. This probe is suitable for food characterization since it is more durable to measure semi-solid samples. Previous studies that used this probe kit have shown positive findings.[Citation30] In this work, automatic calibration was done in seconds just before each measurement was made by using automated Agilent N4693A Electronic Calibration Module. Calibration procedures at the tip of the probe were performed in order to remove systematic (repeatable) errors from measurement.
The meat samples were allowed to reach equilibrium prior to measurement. Each sample was placed in respective 100 mL beaker and then the probe was attached slowly to the sample to measure the complex permittivity. Air bubbles on the tip of the probe should be eliminated to avoid inaccuracy of the measurement results. The receiver detected the reflected and transmitted signals from the material over the frequency range of 0.5 GHz–50 GHz, and the measured response generated phase and magnitude data.[Citation29] All measurements were collected in 50 points within the specified frequency range. The experiments were carried out in an air-conditioned room at the temperature of 20–22°C under MW frequency of 0.5–50 GHz range. The values of dielectric constant (ε′) and dielectric loss factor (ε″) were calculated by the system and displayed as figures of data of permittivity against frequency. Each sample measurement was repeated at least three times until consistent data of dielectric properties was obtained. The limited accuracy is about ±5%, and this is considered adequate for most MW heating research.[Citation31]
Results and Discussion
Four samples of raw beef, raw chicken meat, raw pork, and raw mixed meat (proportion 1:1:1) were subjected to dielectric measurement under the frequency range of 0.5–50 GHz. shows the dielectric constant values for these meat samples. Generally, the values of the electrical permittivity decreased with increasing frequency for all samples. The ε′ values of the meat samples were slightly from one to another. The ε′ values for chicken and pork were slightly higher compared to that of raw beef when tested at low frequency range (below 10 GHz).On the other hand, mixed meat posed the highest dielectric constant value because it was composed of all types of meats tested. Hence, the obtained ε′ was the accumulated ε′ values of all meats. At frequency higher than 15 GHz, raw chicken meat had a slightly higher ε′ values than those of pork and beef. Fluctuations of waves were quite significant in the frequency range of 30 to 50 GHz. However, only pork sample showed fluctuation behavior at low frequency range (below 20 GHz). In addition, two distinct peaks were observed within the range of frequencies studied and this present only for pork sample. The peaks appeared at frequency values of 7.43 GHz (ε′ = 53.2128) and 31.19 GHz (ε′ = 6.1291) and were labeled as Peaks A and B in , respectively. In addition, the spectrum of mixed meat also showed one of these peaks at 31.19 GHz and ε′ of 18.7528.
FIGURE 1 The spectra of dielectric constant for different types of ground raw meats over frequencies up to 50 GHz.
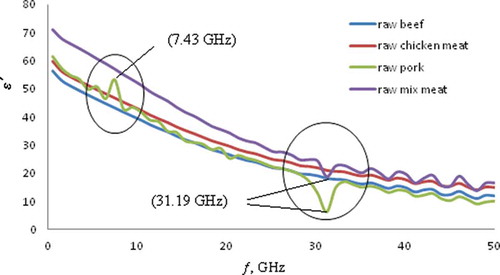
depicts the spectrum of all raw meats for dielectric loss factor (ε″). All spectra of meats exhibited a sharp decline dielectric loss factor at low frequency (0.5–1.5 GHz). The ε″ values then increased until the frequency reached 20 GHz. Once the samples were subjected to higher frequencies (more than 20 GHz), the ε″ values were slowly decreased. The ε″ value for mixed meat sample was higher compared to that of other raw meats sample for all frequencies. In general, the spectrum of mixed meat has a similar pattern to the spectrum of pork sample. Again, both samples exhibited big fluctuation waves above 20 GHz compared to beef and chicken. This large fluctuation behavior in pork and mixed meat spectra hindered the identification of peak at 31.19 GHz. The loss factor spectrum of pork also showed two peaks at approximately 7.43 and 31.19 GHz. Similar to , only one peak was noticeable at approximately31 GHz for mixed meat sample.
FIGURE 2 The spectra of dielectric loss factor for different types of ground raw meats over frequencies up to 50 GHz.
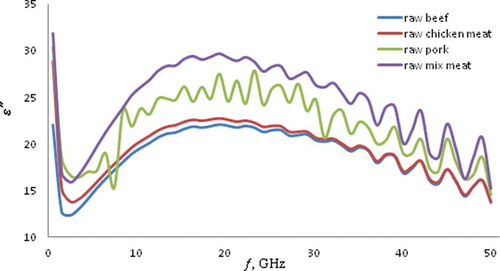
lists the dielectric values of ε′ and ε˝ particularly at the frequencies of 7.43 and 31.19 GHz. The samples used here were raw meats including raw mixed meat. At low frequency around 7 GHz, all meats posed high values of ε′ which were ranked in the order of mixed meat > pork > chicken > beef. Pork showed a slightly higher ε′ and ε˝ values than those of beef and chicken due to high polarization effects that occurred in molecules of pork. Generally, in the range of frequency studied, interface, ionic, and dipolar (orientation) polarization are dominant. Ionic polarization typically occurs at the frequencies between ~109 and 1013 Hz, while dipolar (orientation) polarization involving movement of molecules happens below 109 Hz. At a lower frequency below 109 Hz, interface or space charge polarization occurs. Some or all of these mechanisms may act simultaneously. Some of these mechanisms can be superimposed on other mechanisms.[Citation31–Citation34]
TABLE 1 Dielectric properties of beef, chicken, pork, and mixed meats at frequencies of 7.43 and 31.19 GHz
At a low frequency region, particularly at 7 GHz, ionic and dipolar polarization may be more dominant than others. Some of the potential molecules, such as salt, protein, and carbohydrates, may contribute to these polarization effects. However, as the frequency increases, the dipolar polarization is even slower and ionic polarization becomes effective. The polar molecules are difficult to reorient themselves in a solid or semi-solid (meat) structure as compared to a liquid since the structures of meat are more complex and complicated than liquid.[Citation35,Citation36] This explains why beef, chicken, and pork showed low values of ε′ at the frequency of 31.19 GHz, and the ε′ value of pork was much lower than those of beef and chicken as reflected by the peak. The phenomena were observed to be opposite for dielectric loss factor values at both frequency regions.
shows moisture, protein, and fat contents by percentage of dry weight of the three types of raw ground meats. Other analysis such ash and salt contents were neglected since the average percentages were less than 1.0%.[Citation37]
TABLE 2 Composition of meat samples used in percentage value
Beef, chicken meat, and pork have moisture content in the range of 69.92–70.40%. These values are slightly lower than that reported by Lyng et al.,[Citation36] where the meats used had moisture around 71.50–73.90%. However, the order of the moisture values (pork > chicken > beef) is slightly parallel to the dielectric results presented by them. The difference between both ranges might be due to the heat development or accumulation during grinding of the meat. The grinding process of meat muscle tissue can lead to a local rise of temperature and can reduce water binding capacity.
Chicken had a higher content of protein (23.08%), followed by beef (22.03%), and pork (21.11%). The selection of sample from breast part of chicken instead of the leg, wing, or thigh was to facilitate sample preparation and measurement. It did not affect the protein content since chicken as a whole has higher content of protein compared to beef and pork. Most of the animal meats including beef, chicken, and pork have all the essential amino acids.[Citation16] Amino acids particularly in primary structure possess polarity properties, in which the peptide bonds are linking them in the same orientation. This polarity to some extent is believed to contribute to the polarization effects in dielectric signal.[Citation32] Furthermore, the peptide bonds that hold proteins together can be broken when there is a force exerted to the meat structure. An example of changes in protein molecules is denaturation process. Protein denaturation is defined as irreversible conformational changes in all parts of their molecules, in which the polypeptide chains arrangement become random and lead to the complete loss of biological activity, as well as natural functionality.[Citation38] Denaturation may occur at several stages in the meat processing, such as during local overheating when grinding meat, heat treatment, and dehydration or freeze-drying.[Citation39]
The fat content of meat is an unreliable indicator of dielectric signal since fat (lipid) is generally considered inert dielectrically.[Citation40] This is particularly applicable for unprocessed (raw) meat samples. However, the influence of fat content to dielectric values may be changed if the samples are subjected to any process treatment that involves the transformation of fat to polarized molecules like phospholipids.
It is believed that the insignificant difference of moisture, protein, and fat contents for all the meat samples implied that the two peaks signal observed in the pork sample might originate from other influencing factors such as molecules, compounds, enzymes or foreign matters that were not analyzed here.
The spectra of dielectric constant measurement at 0.5 to 50 GHz for sterilized samples of beef, chicken, and pork is presented in . The other three spectra of raw meat samples were used as comparisons. In general, ε′ values of sterilized pork and sterilized chicken decreased with frequency and chicken exhibited the highest dielectric values. For chicken meat, there was no significant change on dielectric constant values between raw and sterilized samples except at high frequency (>30 GHz), in which small fluctuations that appeared in raw chicken spectrum diminished in sterilized chicken spectrum. Meanwhile, for sterilized beef spectrum, it could be observed that the sample experienced large changes from the sterilization process. This is signified by the reduction of ε′ values at all frequencies when compared to the raw samples.
FIGURE 3 Dielectric constant of sterilized beef, chicken, and pork at the frequencies of 0.5 to 50 GHz.
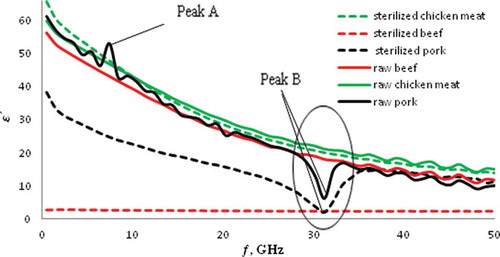
A sudden decrease in the dielectric spectra of sterilized pork was observed at 31–32 GHz, and then started to increase until 38.12 GHz and slowly decreased again until 50 GHz. The decrement values at that frequency formed a broad downward peak similar to Peak B. However, Peak A that appeared at the frequency of 7.43 GHz for raw meat samples vanished through the sterilization process. Apart from that, the fluctuations occurred on the raw pork spectrum also disappeared, and only a linear spectrum could be seen for the sterilized sample.
The almost consistent lower values of sterilized beef (0.1–0.5) could be noticed in the loss factor values (). This is due to the weak interaction between the components or substances of the sterilized beef with the MW radiation for dissipation energy.[Citation41] The fluctuation wave showed earlier in the raw samples data did not appear in the sterilized sample. Similar to the observation in dielectric constant spectra, loss factor of pork samples showed that only Peak B remained present in the spectrum of the sterilized pork.
FIGURE 4 Dielectric loss factor of sterilized beef, chicken, and pork at the frequencies of 0.5 to 50 GHz.
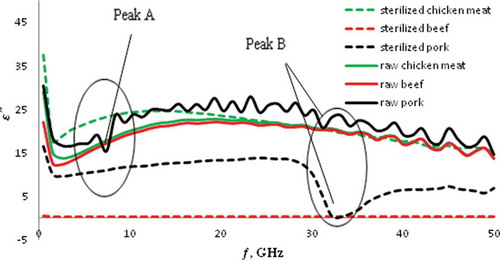
In the canned food industry, most of the foods undergo sterilization treatment before the final products are produced. Normally, for canned meat, sterilization is the process meant to destroy and kill all contaminating pathogenic microorganisms such as bacteria. In this study, although the samples were subjected to high temperature (121°C) during sterilization, the dielectric method was still able to detect some of the specific dielectric properties of the samples especially those that is pertinent to the pork sample only.
Beef experienced substantial changes in their properties after being sterilized compared to chicken and pork. This could be due to the nature of the meat, where beef and pork are considered as red meat, whereas chicken is considered as white meat. One of the determinants of the nutritional definition of the colour of meat is the concentration of myoglobin. Beef has higher myoglobin (1.5–2.0%) compared to white chicken (<0.05%) and pork (0.1–0.3%). Myoglobin is a richly pigmented protein. A higher content of myoglobin in the cells will increase the intensity of the red color and thus, lead to darker meat. Red meat also contains large amounts of iron, creatinine, minerals, such as zinc and phosphorus, and B-vitamins like niacin, vitamin B12, thiamine, and riboflavin. Red meat contains the richest source of lipoic acid, a powerful antioxidant and small amounts of vitamin D. White meat (chicken) is made up of muscles with fibers. It is able to supply fast energy from the glycogen and can be used for quick bursts of activity. Meanwhile, red or dark meat (beef and pork) is made up of muscles with fibers. The protein that they supplied are consistent energy that can be used for extended periods of activity. These constituents of meats, especially polarized molecules myoglobin and other nutrients, may undergo molecular structural changes after the sterilization process. This explains the reason for substantial reduction of the dielectric properties values of the sterilized red meat samples compared to those of raw sample. Moreover, this may be attributed by higher myoglobin content in raw beef and pork compared to other meat types.
Bircan and Barringer[Citation42] reported that the cooking process of meat will cause changes in dielectric properties, and this can be attributed to the denaturation of proteins. The denaturation affects the availability of water for polarization or ionic conduction, and causes permanent loss of protein structure. During sterilization, water in muscle meat is released, while the proteins denatured and reduced the dielectric values of meat. This is also associated with the disappearance of fluctuations at all frequency points.
For pork sample, Peak B at 31.19 GHz persisted even after high-temperature sterilization treatment. This is believed to correspond to polarize molecules that can sustain high temperature since at the frequency in which peak B appeared, ionic polarization dominates. In overall, two distinct peaks appeared in pork and mixed meat spectra at 7.43 and 31.19 GHz, respectively. These peaks can be the potential biomarker to determine and discriminate the species of origin of different types of meat. The potential biomarker can be attributed to some of the constituents such as cytochrome b (cyt b) gene of mitochondrial DNA, aldehyde compounds (heptanal and nonanal), iron content and protein (amino acids and gelatin) in pork which were not destroyed even after the pork samples were subjected to high-temperature sterilization treatment.[Citation14,Citation15,Citation43–Citation46] Jorfi et al.[Citation16] also concluded that there are some discriminative amino acids that differentiate pork and other meats, such as valine, histidine, serine, alanine, and arginine. Trichina worm and Japanese Encephalitis are among foodborne pathogens that present only in pigs and porcine enzymes, which can also be the potential biomarker candidates.
Conclusion
This work has indicated highly promising initial results for discriminating different types of meat and their species of origin. Prominent peaks could be observed at the frequencies of 7 GHz (Peak A) and 30 GHz (Peak B) for the pork sample, and these peaks can be the potential biomarkers to discriminate these samples. The suspected biomarkers can be compounds that are not destroyed when meat is subjected to high-temperature treatment. This research study indicates that the dielectric method has proven to be non-laborious, rapid on-site detection, and easy to handle, which promises clear distinctive results. The usage of MW frequency until 50 GHz offers broad detection range and appears to be effective on products containing pork species as reflected by the results in this work. Due to its simplicity, this technique can be utilized as a support and initial screening before proceeding to detailed analysis using other time-consuming methods. For future recommendations, more products related to pork origin such as lard, tallow, whey, and sausages could be characterized under a similar frequency range. An in-depth study of detecting the constituents in meats can be undertaken in order to correlate the dielectric data with prohibited species, especially in pork.
Funding
The researchers would like to thank Universiti Putra Malaysia (UPM) for funding the work (05-05-10-1079RU) and also Halal Product Research Institute, UPM for their kind assistance.
Nomenclature
GHz | = | Gigahertz |
MW | = | Microwave |
RF | = | Radio frequency |
ε* | = | Debye relation of dielectric permittivity |
ε′ | = | Dielectric constant |
ε″ | = | Dielectric loss factor |
ω | = | Angular frequency (ω=2πƒ) in rad/s |
Acknowledgements
The authors would like to thank Agilent for the PNA machine, as well as Prof. Gerard Markx for useful discussion.
Additional information
Funding
REFERENCES
- Miller, R.K. Factors Affecting the Quality of Raw Meat. In Meat Processing, Improving Quality; Kerry, J.; Kerry, J.; Ledward, D.; Eds.; Woodhead Publishing Limited: Cambridge, England, 2002; 27–57 pp.
- Tornberg, E. Effects of Heat on Meat Proteins—Implications on Structure and Quality of Meat Products. Meat Science 2005, 70(3), 493–508.
- Martens, H.; Stabursvik, E.; Martens, M. Texture and Colour Changes in Meat During Cooking Related to Thermal Denaturation of Muscle Proteins. Journal of Texture Studies 1982, 13, 291–309.
- Rodriguez, M.A.; García, T.; González, I.; Asensio, L.; Hernández, P.E.; Martín, R. PCR Identification of Beef, Sheep, Goat, and Pork in Raw and Heat-Treated Meat Mixture. Journal of Food Protection 2004, 67(1), 172–177.
- Al-Qaradawi, Y. The Lawful and the Prohibited in Islam; Islamic Book Trust: Kuala Lumpur, 1995.
- Berger, R.G.; Mageau, R.P.; Schwap, B.; Johnston, R.W. Detection of Poultry and Pork in Cooked and Canned Meat Foods by Enzyme Linked Immunosorbent Assays. Journal Association of Official Analytical Chemists 1988, 71(2), 406–409.
- Swanson, M.C.; Boiano, J.M.; Galson, S.K.; Grauvogel, L.W.; Reed, C.E. Immunochemical Quantification and Particle Size Distribution of Airborne Papain in a Meat Portioning Facility. American Industrial Hygiene Association Journal 1992, 53(1), 1–5.
- Hofmann, K. Fundamental Problems in Identifying the Animal Species of Muscle Meat Using Electrophoretic Methods. Fleischwirtshaft 1987, 67, 820–826.
- Slattery, W.J.; Sinclair, J. Differentiation of Meat According to Species by the Electrophoretic Separation of Muscle Lactate Dehydrogenase and Esterase Isoenzymes and Isoelectric Focusing of Soluble Muscle Proteins. Australian Veterinary Journal 1983, 60(2), 47–51.
- Fei, S.; Okayama, T.; Yamanoue, M.; Nishikawa, I.; Mannen, H.; Tsuji, S. Species Identification of Meats and Meat Products by PCR. Animal Science and Technology, (Jpn) 1996, 67, 900–905.
- Miguel, A.R.; Teresa, G.; Isabel, G.; Luis, A.; Pablo, E.H.; Rosario, M. Identification of Beef, Sheep, Goat, and Pork in Raw and Heat Treated Meat Mixtures. Journal of Food Protection 2004, 67, 172–177.
- Che Man, Y.B.; Aida, A.A.; Raha, A.R.; Son, R. Identification of Pork Derivatives in Food Products by Species-Specific Polymerase Chain Reaction (PCR) for Halal Verification. Food Control 2007, 18, 885–889.
- Matsunaga, T.; Chikuni, K.; Tanabe, R.; Muroya, S.; Shibata, K.; Yamada, J.; Shinmura, Y. A Quick and Simple Method for Identification of Meat Species and Meat Products by PCR Assay. Meat Science 1999, 51, 143–148.
- Murugaiah, C.; Noor, Z.M.; Mastakim, M.; Bilung, L.M.; Selamat, J.; Radu, S. Meat Species Identification and Halal Authentication Analysis Using Mitochondrial DNA. Meat Science 2009, 83, 57–61.
- Nurjuliana, M.; Che Man, Y.B.; Hashim, D.M.; Mohamed, A.K.S. Rapid Identification of Pork for Halal Authentication Using the Electronic Nose and Gas Chromatography Mass Spectrometer with Headspace Analyzer. Meat Science 2011, 88, 638–644.
- Jofri, R.; Mustafa, S.; Che Man, Y.; Mat Hashim, D.; Sazili, A.Q.; Farjam, A.S.; Nateghi, L.; Kashiani, P. Differentiation of Pork from Beef, Chicken, Mutton, and Chevron According to Their Primary Amino Acids Content for Halal Authentication. African Journal of Biotechnology 2012, 11(32), 8160–8166.
- BIBLIOGRAPHY Abidin, Z.Z.; Omar, F.N.; Awang Biak, D.R. Dielectric Characterization of Ethanol and Sugar Aqueous Solutions for Potential Halal Authentication. International Journal of Microwave and Optical Technology 2014a, 9(1), 39–43.
- Bengtsson, N.E.; Melin, J.; Remi, K.; Soderlind, S. Measurement of Dielectric Properties of Frozen and Defrosted Meat and Fish in the Frequency Range 10–200 MHz. Journal of the Science of Food Agriculture 1963, 14, 592–604.
- Zhang, L.; Lyng, J.G.; Brunton, N.; Morgan, D.; McKenna, B. Dielectric and Thermophysical Properties of Meat Batters over a Temperature Range of 5–85°C. Meat Science 2004, 68, 173–184.
- Castro-Giráldez, M.; Toldrá, F.; Fito, P. Low Frequency Dielectric Measurements to Assess Post-Mortem Ageing of Pork Meat. Food Science and Technology 2011, 44, 1465–1472.
- Brunton, N.P.; Lyng, J.G.; Zhang, L.; Jacquier, J.C. The Use of Dielectric Properties and Other Physical Analyses for Assessing Protein Denaturation in Beef Biceps Femoris Muscle During Cooking from 5 to 85°C. Meat Science 2006, 72, 236–244.
- Abidin, Z.Z.; Omar, F.N.; Awang Biak, D.R. Dielectric Characterization of Liquid Containing Low Alcoholic Content for Potential Halal Authentication on the 0.5–50 GHz Range. American Journal of Applied Science 2014b, 11(7), 1104–1112.
- Wang, M.L.; Choong, Y.M.; Su, N.W.; Lee, M.H. A Rapid Method for Determination of Ethanol in Alcoholic Beverages Using Capillary Gas Chromatography. Journal of Food and Drug Analysis 2003, 11, 133–140.
- Tang, J. Dielectric Properties of Foods. In The Microwave Processing of Foods; Schubert, H.; Regier, M.; Eds.; Woodhead Publishing Limited: Cambridge, 2005; 22–38 pp.
- Von Hippel, A.R. Dielectric Properties and Waves. John Wiley: New York, NY, 1954.
- Smith Jr., R.L.; Lee, S.B.; Komori, H.; Arai, K. Relative Permittivity and Dielectric Relaxation in Aqueous Alcohol Solutions. Fluid Phase Equilibra 1998, 144, 315–322.
- Zhang, L.; Lyng, J.G.; Brunton, N.P. The Effect of Fat, Water, and Salt on the Thermal and Dielectric Properties of Meat Batter and Its Temperature Following Microwave Or Radio Frequency Heating. Journal of Food Engineering 2007, 80, 142–151.
- Kent, M.; Anderson, D. Dielectric Studies of Added Water in Poultry Meat and Scallops. Journal of Food Engineering 1996, 28, 239–259.
- Agilent Technologies. Agilent Basics of Measuring the Dielectric Properties of Materials, Application Note. United States of America: Agilent Technologies Inc. 2006, 5989–2589EN.
- Castro-Giraldez, M.; Fito, P.J.; Fito, P. Application of Microwaves Dielectric Spectroscopy for Controlling Pork Meat (Longissimus Dorsi) Salting Process. Journal of Food Engineering 2010, 97, 484–490.
- Tang, J.; Hao, F.; Lau, M. Microwave Heating in Food Processing. Chapter 1—Advances in Bioprocessing Engineering Volume 1. Rarred Road, Singapore: World Scientific Publishing Co. Pte. Ltd., 2002.
- Foster, K.R.; Schwan, H.P. Dielectric Properties of Tissues and Biological Materials, a Critical Review in Critical Reviews in Biomedical Engineering; CRC Press: Boca Raton, FL, 1989, 25–104 pp.
- Dakin, T.W. Conduction and Polarization Mechanisms and Trends in Dielectrics. IEEE Electrical Insulation Magazine 2006, 22(5), 11–28.
- Kremer, F.; Schonhals, A.; Luck, W. Broadband Dielectric Spectroscopy; Springer-Verlag: Berlin, Germany, 2002.
- Pethig, R. Dielectric and Electronic Properties of Biological Materials; Wiley: New York, NY, 1979.
- Frohlich, H. Theory of Dielectrics; Dielectric Constant and Dielectric Loss Factor, 2nd Ed.; Clarendon Press: Oxford, United Kingdom, 1958.
- Lyng, J.G.; Zhang, L.; Brunton, N.P. A Survey of the Dielectric Properties of Meats and Ingredients Used in Meat Product Manufacture. Meat Science 2005, 69, 589–602.
- Davidek, J.; Velisek, J.; Pokorny, J. Chemical Changes During Food Processing, Vol. 21.; Elsevier Science Publishing Company, Inc.: New York, NY, 1999.
- Lawrie, R.A. Meat Science, 2nd Ed.; Braunschweig Oxford: New York, NY, 1974.
- Mudgett, R.E.; Goldblith, S.A.; Wang, D.I.C.; Westphal, W.B. Prediction of Dielectric Properties in Solid Foods of High Moisture Content at Ultrahigh and Microwave Frequencies. Journal of Food Process Preservation 1977, 1, 119–151.
- Ahmed, J.; Ramaswamy, H.S.; Raghavan, V.S.G. Dielectric properties of Indian Basmati Rice Flour Slurry. Journal of Food Engineering 2007, 80, 1125–1133.
- Bircan, C.; Barringer, S.A. Use of Dielectric Properties to Detect Egg Protein Denaturation. Journal of Microwave Power and Electromagnetic Energy 2002b, 37, 89–96.
- Wettasinghe, M.; Vasanthan, T.; Temelli, F.; Swallow, K. Volatile Flavour Composition of Cooked by-Product Blends of Chicken, Beef, and Pork: A Quantitative GC-MS Investigation. Food Research International 2001, 34(2), 149–158.
- Schlundt, J. New Directions in Foodborne Disease Prevention. International Journal of Food Microbiology 2002, 78, 3–17.
- Min, B.R.; Nam, K.C.; Cordray, J.C.; Ahn, D.U. Factors Affecting Oxidative Stability of Pork, Beef, and Chicken Meat. Animal Industry Report 2008, AS 654, ASL R2257.
- Hashim, D.M.; Che Man, Y.B.; Norakasha, R.; Shuhaimi, M.; Salmah, Y.; Syahariza, Z.A. Potential Use of Fourier Transform Infrared Spectroscopy for Differentiation of Bovine and Porcine Gelatins. Food Chemistry 2010, 118, 856–860.