Abstract
In-package pasteurization treatments based on radio frequency or microwave energy can greatly reduce the danger of microbial spoilage for Chinese steamed bread. The mixture equations methods were used to obtain dielectric properties at the frequency from 1 to 2450 MHz for the temperature range of 25–100ºC and the moisture content range of 40.1–48.5%. The effects of frequency, moisture content, and temperature on Chinese steamed bread dielectric properties were analyzed. The Bottcher Equation was adapted for calculating dielectric constant, the Landau and Lifshitz, Looyenga Equation for dielectric loss factor. Both dielectric constants and dielectric loss factors increased with increasing moisture content and temperature. Radio frequency energies had several times deeper in Chinese steamed bread than microwave energies.
Introduction
Chinese steamed bread (CSB; sometimes written as Chinese steamed bun or mantou) is a type of fermented and steamed wheat food with distinctive cultural features. It has been consumed in some parts of China as a staple food over two millennia, and the popularity is increasing around the world. It is considered as healthy food due to the absence of toxic Maillard reaction products such as acryl amide and furan,[Citation1] and also possible low oil and sodium contents. The relatively low steaming temperature (100ºC) during production may render better retention of diverse endogenous and added nutrients as compared to the baked bread.[Citation2] However, intermediate and high moisture steamed products are susceptible to rapid microbial spoilage, especially the growth of mold, which greatly limits their shelf life. Therefore, methods of controlling microbial corruption play a vital role in the industry.[Citation3–Citation5] In the past few decades, radio frequency (RF) and microwave (MW) heating have been studied to control mold growth and extend the shelf life of food products.[Citation6,Citation7] However, in order to properly design an effective dielectric heating system, it is desirable to determine the factors that affect the rate of heating throughout the product. The dielectric properties of foods are the principal parameters that determine the coupling and distribution of electromagnetic (EM) energy during dielectric heating.
The dielectric properties of food described its ability to store and dissipate electrical energy in respond to an alternating EM field.[Citation8] Dielectric properties are normally described by complex permittivity,
where ;
, the real component, referred to as dielectric constant, describes the ability of a material to store electric energy;
, the imaginary component, referred to as loss factor, determines energy dissipation in the material or the conversion from electrical energy into heat.
When subjected to fields of RF or MW and for the design and development of continuous systems, knowledge of dielectric properties is indispensable for a better understanding of the heating behavior of the treated samples, and for evaluating the potential of RF or MW differential heating. Dielectric properties data for several foods (parchment coffee, legume flours, safflower seed) have been reported.[Citation9–Citation11] Dielectric properties of a given food materials should be influenced by various factors, including frequency, temperature, moisture content, and other food compositions, such as salt content.[Citation12–Citation15] The relationship between the frequency and dielectric properties is helpful to determine the optimum frequency range in which the material has the desired dielectric characteristics.[Citation16] The relationship between moisture and dielectric properties in specific frequency ranges is used to develop online moisture meters, which applied not only during the drying processes but also in other operating units in the food industry.[Citation17] Most of the literature measurements on dielectric properties of food products were carried out in the MW frequency range, while limited studies can be found in the RF range (10–100 MHz). Among the commonly used methods, the transmission line technology was proved to be the most suitable for measuring porous materials.[Citation18] The open-ended coaxial cable method has a large bandwidth and was widely used in the food industry, moreover, it was easy to use.[Citation19–Citation22] But according to several studies,[Citation23,Citation24] when using a coaxial method of measuring the dielectric properties of porous materials, the repetition rate was very poor. This was due to the uncontrolled contact pressure during measurement and non-homogenous structure of porous materials. In order to use the coaxial probe method to obtain reliable dielectric properties of CSB, homogeneous structure for CSB was particularly necessary.
The dielectric properties of porous material (air-particle mixture) characteristic can be estimated by mixture equations.[Citation25–Citation27] As long as using the experimental method to obtain dielectric data for homogenous and truly dense CSB materials, it was possible to use those mixture equations in calculating dielectric properties of original porous CSB. There is negligible data in the literature for CSB dielectric properties over a broad frequency range and the lack of information about the relationship between dielectric properties and influencing factors. The goals of this study were to determine dielectric properties and the penetration depth of CSB using the open-ended coaxial probe and mixture equations method at the frequency range between 1 and 2450 MHz and the temperature range of 25–100ºC, and research on the relationships between dielectric properties of CSB and influencing factors, including frequency, moisture content, and temperature.
Materials and methods
Sample Preparation
Sliced CSB were purchased from a local student cafeteria in Jiangnan University, Wuxi and refrigerated 5ºC for less than 1 week before testing. The ingredients of CSB are shown in , among which moisture content,[Citation28] ash content,[Citation29] and porosity were measured in the laboratory and the others were based on the label. CSB slices were either laid in air to let go of some water to obtain lower moisture contents or covered with a wetted paper towel to get higher moisture contents, which was used to adjust moisture content of samples. Slightly dried and moistened CSB slices were packaged in Ziploc bags. Then, samples were placed at room temperature for 12 h to equilibrate the moisture. Sliced CSB were compressed into a stainless steel small cylinder (diameter: 30 mm, height: 30 mm) to remove air voids before measurements, the weight of each CSB sample was measured using an precision electronic balance (Ohaus Corp., Pine Brook, NJ, USA).
TABLE 1 Compositions analysis of steamed bun samples (mean of three replicates)
Density and Porosity
The apparent density of CSB crumb was determined by measuring the mass and volume. The particle density of CSB crumb was measured with a pycnometer Accupyc 1330 (Micromeritics, USA) using toluene as the displacement fluid. The porosity (e) of samples was given by Rahman:[Citation30]
Dielectric Properties Measurement
Five frequencies for industrial, scientific, and medical applications were selected and used, namely 13.56, 27.12, and 40.68 MHz in RF range and 915 and 2450 MHz in MW range. Dielectric properties of CSB were measured by the open-ended coaxial cable technique between 1 MHz and 2450 MHz. The test system consisted of an Agilent E5061B network analyzer with a calibration kit (Agilent Technologies, 85033E), an open-ended coaxial-cable, and 85070E dielectric probe kit. In the current measuring techniques in broad band of dielectric property measurement, the open-ended coaxial-cable method is most commonly used. CSB slices were compressed into small cylinder (30 mm inner diameter, 30 mm height) until they reached the required density. The surface of compressed was leveled, and slightly compressed by the coaxial probe before measurements.
Mixture Equations for Dielectric Properties
The global dielectric properties of a multiphase material can also be estimated from the proportions and properties of their individual constituent phases by using mixing equations. The best fit should be chosen depending on the material type, shape, and complexity. Sheen et al.[Citation31] compared exponential and logarithmic models, estimated their theoretical error, and correlated them with experimental data. They suggested that the complex refractive model and the random model were the most suitable for a polymer-ceramic mixture at a high volume fraction of the dispersed phase. These models are also widely mentioned in case of agricultural and food products.[Citation15] Therefore, the complex refractive index mixing model (CRIME, Eq. [Citation4]), the random model (Landau–Lifshitz–Looyenga [LLLE] Eq. [Citation5]) and a logarithmic model (Lichtenecker–Rother, LRE, Eq. [Citation6]), and Bottcher equation (BE; Eq. [Citation7]) were evaluated in Alfaifafi et al.[Citation32] and used in this study to estimate the material dielectric properties of an air-particle mixture as a function of the sample porosity.
In Eqs. (4–7), refers to the dielectric property of the mixture, and
correspond to the air and CSB phases, respectively, and v1, v2 to their volume fractions of the respective components, and v1 + v2 = 1. CSB were considered as a compound of air voids and solid CSB materials. In the above given equations, the dielectric property of air,
, is 1- j0, the corresponding v1 is the value of CSB porosity
; and v2 is the volume of CSB after being compressed, the corresponding
is the dielectric property of solid CSB materials. It was difficult to obtain the real complex dielectric property of the dense CSB materials (without air voids) directly, therefore,
was calculated by extrapolation from directly measured dielectric properties of CSB slices that had been compressed to three different densities.
Penetration Depth
The penetration depth of RF and MW energy is defined as the depth of the incident power reduced to 1/ (
2.718) at the surface of the material. The penetration depth
in meters for RF and MW power was calculated according to:[Citation33]
where c is the speed of light in free space () and f is the frequency of the EM wave in Hz.
Results and discussion
Selection of Mixture Equation
According to the preliminary study, relationships between dielectric constant (), dielectric loss factor (
), and frequency (f, MHz) can be described by:
where A, B, C, and D are constants. The above relationship were used in Liu et al.[Citation4] The corresponding constants of Eqs. (9) and (10) are listed in . The constants in are linearly associated with density of steamed buns (), as follows:
Dielectric properties for compressed CSB samples with 46.8% moisture content at three densities and room temperature (21ºC) are shown in and . The density of absolutely compressed CSB () was measured to be 1.2 g/cm3 using toluene as the displacement fluid with a pycnometer. The dielectric constants and dielectric loss factors of the dense CSB materials (CSB without air voids) at 46.8% moisture content and room temperature 21ºC can be calculated from Eqs. (9) and (10) by setting
in Eqs. (10–13) as:
FIGURE 1 Dielectric constants A: and dielectric loss factors
B: of compressed steamed bun samples with 46.8% moisture content at three densities and 21ºC.
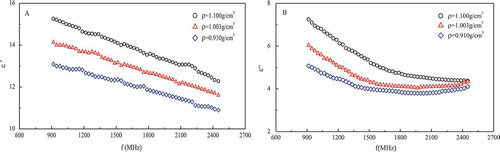
With the estimated dielectric properties and
for the solid CSB material (without air voids), the mixture equations were used to calculate
and
of CSB samples at different densities. The measured and calculated dielectric properties for CSB samples at 46.8% moisture content and room temperature 21ºC are shown in . As seen from and , the dielectric constant data of CSB calculated from BE equation fits better the measured values than the others, while the dielectric loss factor data calculated from LLLE were closest with the measured values.
TABLE 3 Measured and calculated dielectric properties for Chinese steamed bread samples with 46.8% moisture content at 21ºC
FIGURE 2 Measured and calculated dielectric properties for Chinese steamed bread samples with 46.8% moisture content at 21ºC ().
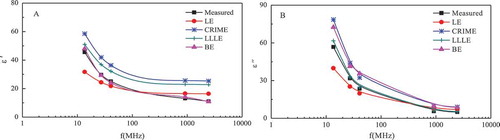
The dielectric properties of compressed CSB samples at six different temperatures (25, 40, 55, 70, 85, and 100ºC) for four moisture contents (48.5, 46.8, 43.6, and 40.1%) were obtained at five frequencies (13.56, 27.12, 40.68, 915, and 2450 MHz). At each moisture content level, six different temperatures were tested. According to the relationship between the dielectric properties and CSB density, the dielectric properties of CSB materials (without air voids) were calculated. From the foregoing, BE was used to calculate the dielectric constant () and LLLE was used to calculate the dielectric loss factor (
) of compressed CSB. Errors between the measured and calculated dielectric properties were counted according to the following equation:
By comparing all the errors between the measured and calculated values, 25% of the errors in the range of 10%, 75% fall into the range of 30%, 100% fall into the range of 40%. Considering the porosity of the CSB is 0.75, that is, CSB materials only account for 25% of the original CSB, the total error of dielectric properties of original CSB samples were most 10%. Therefore, BE was applied to calculate the dielectric constants and LLLE was used to calculate of dielectric loss factors of CSB materials.
Dielectric Properties of CSB
The values of and
of CSB (porosity = 0.75) were estimated from the measured values of compressed CSB samples using the mixture equations for five frequencies (13.56, 27.12, 40.68, 915, and 2450 MHz), four moisture contents (48.5, 46.8, 43.6, and 40.1%), and six different temperatures (25, 40, 55, 70, 85, and 100ºC). The CSB values of each group measured repeatedly three times. These values are shown in .
TABLE 4 Dielectric properties (mean ± standard deviation) of Chinese steamed bread samples with different moisture levels at 25–100ºC
Frequency-Dependent Dielectric Properties
As shown in , at any given temperature, dielectric constants of CSB (
) decreased with the increase of frequency at the moisture content 46.8%. showed that dielectric loss factors
of CSB decreased sharply with the increase in frequency up to 915 MHz, and then increased gently above 915 MHz any given temperature. The variation tendency of dielectric constant described above was consistent with findings reported by Nelson[Citation34] that dielectric constant either decreased or remained constant with the frequency increased under the condition of other influence conditions remain unchanged. Ionic conduction and dipole rotation are the dominant loss mechanisms at RF and MW frequencies for industrial dielectric heating.[Citation35,Citation36] It can be mathematically expressed as:
FIGURE 3 Values of Chinese steamed bread dielectric constants A: and loss factors
B: at 46.8% moisture content and six temperatures (
).
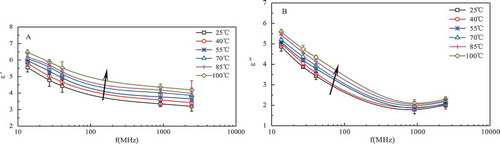
where and
are dielectric loss factors due to ionic conduction and dipole rotation, respectively.
is further expressed as:
Equation (18) was used in Liu et al.,[Citation4] where is ionic conductivity of a sample in S/m; and
is the permittivity of free space,
. Eq. (18) showed a negative linear relationship between loss factor contributed by ionic conductivity and frequency in a log-log plot. This can be seen the frequency range of 1- 915 MHz in . The regression analysis results and the coefficients of determination R2 of frequency dependent about dielectric constants and loss factors for CSB samples at 46.8% moisture content and six temperatures are listed in .
TABLE 5 Regression analysis results and R2 about frequency, moisture content, and temperature dependent of dielectric properties for steamed buns
Moisture Content-Dependent Dielectric Properties
As illustrated in and , dielectric constants and loss factors of CSB gently increased linearly with the moisture content at the temperature 25ºC and at any given frequency. This may be due to the increase in the amount of water molecules in CSB samples at high moisture content.[Citation37] In any given moisture content, dielectric constants decreased with the increasing frequency, while dielectric loss factors decreased with the increase of frequency except 2450 MHz. In summary, the effect of moisture content on RF frequencies of dielectric constants and loss factors was higher than MW frequencies. The and
of CSB materials were linear correlation with the moisture content (M, %).The moisture content dependent regression analysis results and the R2 data of dielectric properties for steamed buns at 25ºC and five frequencies are listed in .
Temperature-Dependent Dielectric Properties
As illustrated in and and shown in , dielectric constants and loss factors gradually increased with the increase of temperature at 27.12 MHz and at any given moisture contents. The observed variation tendency of dielectric constant with changes of temperature is consistent with findings reported by Nelson[Citation34] and Feng et al.[Citation38] The CSB samples had about 4.6% ash content (). The ash content played a significant role in the dielectric loss factor of CSB samples. According to the preliminary study, dielectric properties ( and
) and temperature (T, ºC) can be linear correlation. The temperature dependent regression analysis results and the R2 data of dielectric properties for CSB at 27.12 MHz and four moisture contents are listed in . Compared with dielectric constant, dielectric loss factors increased more sharply.
Frequency, Moisture Content, and Temperature-Dependent Penetration Depth
The penetration depth of CSB samples for five frequencies (13.56, 27.12, 40.68, 915, and 2450 MHz), four moisture contents(48.5, 46.8, 43.6, and 40.1%), and six different temperatures (25, 40, 55, 70, 85, and 100ºC) were calculated from average values of dielectric constants and loss factors. As shown in , the penetration depth for CSB decreased with increasing frequency and indicated temperatures at 46.8% moisture content. That is, the shorter the wavelength, the shallower the penetration depth. Furthermore, the depth decreased more rapidly in MW range than in RF range. The depth decreased linearly with increasing moisture content, and the depth decreased more sharply in RF range than in MW range (). Overall, the penetration depth of CSB samples decreased with the increase of influencing factors. The calculated penetration depth data of CSB were shown in . In order to achieve uniform pasteurization, the thickness of the food material shall not exceed two to three times the penetration depth.[Citation38] If the smallest penetration depth at 25–100ºC were used, the thickness of CSB for dielectric heating would be about 61 cm at 13.56 MHz, 31 cm at 27.12 MHz, 29 cm at 40.68 MHz, 10 cm at 915 MHz, and 3 cm at 2450 MHz.
TABLE 6 The penetration depth (cm) of RF and MW energy in steamed bun materials
Conclusions
Network analyzer with an open coaxial cable and mixture equations method was used to estimate the dielectric properties of porous CSB. The permittivities of CSB over influencing factors revealed that frequency, moisture content, and temperature affected the dielectric behavior of CSB significantly. Linear relationship between dielectric properties of CSB and moisture content and temperature were observed. Both dielectric constants and dielectric loss factors increased with increasing moisture content and temperature. Logarithmic relationship between dielectric properties and frequency were observed. Dielectric constants decreased flatly with the increase of frequency, meanwhile, dielectric loss factors decreased sharply to a minimum and then increased gently as frequency increased. The penetration depth of EM waves in CSB samples decreased with the increase of all influencing factors. Radio frequencies had deeper penetration in CSB than MW frequencies. These measurements provided valuable information about the frequency, moisture content, and temperature-dependent behavior of the dielectric properties of CSB for development of a whole package baked goods with radio-frequency and MW dielectric heating.
FUNDING
The authors acknowledge financial support from the National Natural Science Fund of China (21206051), and partial support from Jiangsu Key Laboratory of Advanced Food Manufacturing Equipment and Technology (Jiangnan University, China; FM-201503) and Fundamental Research Funds for the Central Universities (JUSRP51511).
Additional information
Funding
REFERENCES
- Su, D.M.; Ding, C.H.; Li, L.; Su, D.H.; Zheng, X.Y. Effect of Endoxylanases on Dough Properties and Making Performance of Chinese Steamed Bread. European Food Research and Technology 2005, 220, 540–545.
- Zhu, F. Influence of Ingredients and Chemical Components on the Quality of Chinese Steamed Bread School of Chemical. Food Chemistry 2014, 163, 154–162.
- Smith, J.P.; Daifas, D.P.; El-Khoury, W.; Koukoutsis, J.; El-Khoury, A. Shelf Life and Safety Concerns of Bakery Products—A Review. Critical Reviews in Food Science and Nutrition 2004, 44, 19–55.
- Liu, Y.; Tang, J.; Mao, Z. Analysis of Bread Dielectric Properties Using Mixture Equations. Journal of Food Engineering 2009, 93(1), 72–79.
- Liu, Y.; Tang, J.; Mao, Z. Analysis of Bread Loss Factor Using Modified Debye Equations. Journal of Food Engineering 2009, 93, 453–459.
- Tang, J. Dielectric Properties of Foods. The Microwave Processing of Foods (Chapter 2); Wood Head Publishing Limited: Cambridge, MA, 2005; 22–40.
- Lakins, D.G.; Echeverry, A.; Alvarado, C.Z.; Brooks, J.C.; Brashears, M.T.; Brashears, M.M. Quality of and Mold Growth on White Enriched Bread for Military Rations Following Directional Microwave Treatment. Journal of Food Science 2008, 73, M99–M103.
- Wang, J.; Tang, J.; Wang, Y.F.; Swanson, B. Dielectric Properties of Egg Whites and Whole Egg As Influenced by Thermal Treatments. LWT–Food Science and Technology 2009, 42, 1204–1212.
- Berbert, P.A.; Queiroz, D.M.; Sousa, E.F.; Molina, M.B.; Melo, E.C.; Faroni, L.R.D. Dielectric Properties of Parchment Coffee. Journal of Agricultural Engineering Research 2001, 80(1), 65–80.
- Guo, W.; Wang, S.; Tiwari, G.; Johnson, J.A.; Tang, J. Temperature and Moisture Dependent Dielectric Properties of Legume Flours Associated with Dielectric Heating. LWT–Food Science and Technology 2010, 43(2), 193–201.
- Sacilik, K.; Tarimci, C.; Colak, A. Moisture Content and Bulk Density Dependence of Dielectric Properties of Safflower Seed in the Radio Frequency Range. Journal of Food Engineering 2006, 78(4), 1111–1116.
- Calay, R.K.; Newborough, M.; Probert, D.; Calay, P.S. Predictive Equations for the Dielectric Properties of Foods. International Journal of Food Science and Technology 1995, 29, 699–713.
- Sun, E.; Datta, A.; Lobo, S. Composition-Based Prediction of Dielectric Properties of Foods. Journal of Microwave Power and Electromagnetic Energy 1995, 30(4), 205–212.
- Tang, J.; Wang, Y.; Chan, T.V.C.T. Radio-Frequency Heating in Food Processing. In Novel Food Processing Technologies; Barbosa-Canovas, G.V.; Tapia, M.S; Cano, M.P.; Eds.; CRC Press: New York, NY, 2005; 501–524.
- Venkatesh, M.S.; Raghava, G.S.V. An Overview of Microwave Processing and Dielectric Properties of Agri-Food Materials. Biosystems Engineering 2004, 88(1), 1–18.
- Wang, S.; Birla, S.L.; Tang, J.; Hansen, J.D. Postharvest Treatment to Control Codling Moth in Fresh Apples Using Water Assisted Radio Frequency Heating. Postharvest Biology and Technology 2006, 40, 89–96.
- Berbert, P.A.; Queriroz, D.M.; Melo, E.C. Dielectric Properties of Common Bean. Biosystems Engineering 2002, 83, 449–462.
- Goedeken, D.L.; Tong, C.H.; Virtanen, A.J. Dielectric Properties of a Pregelatinized Bread System at 2450 Mhz As a Function of Temperature, Moisture, Salt, and Specific Volume. Journal of Food Science 1997, 62(1), 145–149.
- Nelson, S.O.; Kraszewski, A.W. Dielectric Properties of Materials and Measurement Techniques. Drying Technology 1990, 8(5), 1123–1142.
- Engelder, D.S.; Buffler, C.R. Measuring Dielectric Properties of Food Products at Microwave Frequencies. Microwave World 1991, 12(2), 6–15.
- Nelson, S.O. Dielectric Properties Measurement Techniques and Applications. Transactions of the ASAE 1999, 42(2), 523–529.
- Venkatesh, M.S.; Raghavan, G.S.V. An Overview of Dielectric Properties Measuring Techniques. Canadian Biosystems Engineering 2005, 47, 7.15–7.30.
- Sumnu, G.; Datta, A.K.; Sahin, S.; Keskin, S.O.; Rakesh, V. Transport and Related Properties of Breads Baked Using Various Heating Modes. Journal of Food Engineering 2006, 78(4), 1382–1387.
- Sakiyan, O.; Sumnu, G.; Sahin, S.; Meda, V. Investigation of Dielectric Properties of Different Cake Formulations During Microwave and Infrared-Microwave Combination Baking. Journal of Food Science 2007, 72(4), E205–E213.
- Lal, K.; Parshad, R. The Permittivity of Heterogeneous Mixtures. Journal of Physics D: Applied Physics 1973, 6, 1363–1368.
- Lal, K.; Parshad, R. Permittivity of Conductor–Dielectric Heterogeneous Mixtures. Journal of Physics D: Applied Physics 1973, 6, 1788–1792.
- Sihvola, A.H.; Kong, J.A. Effective Permittivity of Dielectric Mixtures. IEEE Transactions on Geoscience and Remote Sensing, 1988, 26(4), 420–429.
- AOAC. AOAC Official Method, 2000, 925.09.
- AOAC. AOAC Official Method, 2000, 923.03.
- Rahman, M.S. Mass-Volume-Area-Related Properties of Foods. In Engineering Properties of Foods; Rao, M.A.; Rizvi, S.S.H.; Datta, A.K.; Eds.; CRC Press: Boca Raton, FL, 2005; 1–39.
- Sheen, J.; Hong, Z.W.; Su, C.W.; Chen, H.C. Microwave Measurements of Dielectric Constants by Exponential and Logarithmic Mixture Equations. Progress in Electromagnetic Research 2010, 100, 13–26.
- Alfafi, B.; Tang, J.; Jiao, Y.; Wang, S.; Rasco, B.; Jiao, S.; Sablani, S. Radio Frequency Disinfestation Treatments for Dried Fruit: Model Development and Validation. Journal of Food Engineering 2014, 120, 268–276.
- Metaxas, A.C.; Meredith, R.J. Industrial Microwave Heating; Peter Peregrinus Ltd.: London, 1983.
- Nelson, S.O. Electrical Properties of Grain and Other Food Materials. Journal of Food Processing and Preservation 1978, 2, 137–154.
- Ryynänen, S. The Electromagnetic Properties of Food Materials: A Review of the Basic Principles. Journal of Food Engineering 1995, 26(4), 409–429.
- Zhu, X.; Guo, W.; Wu, X. Frequency- and Temperature-Dependent Dielectric Properties of Fruit Juices Associated with Pasteurization by Dielectric Heating. Journal of Food Engineering 2012, 109(2), 258–266.
- Datta, A.K.; Sumnu, G.; Raghavan, G.S.V. Dielectric Properties of Foods. In Engineering Properties of Foods; Rao, M.A.; Rizvi, S.S.H.; Datta, A.K.; Eds.; CRC Press: Boca Raton, FL, 2005; 501–565.
- Feng, H.; Tang, J.; Cavalieri, R.P. Dielectric Properties of Dehydrated Apples As Affected by Moisture and Temperature. Transactions of the ASAE 2002, 45(1), 129–135.