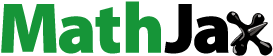
ABSTRACT
The objective of this study was to determine the effects of rice residue on the physicochemical properties of silver carp surimi gels. The whiteness of gels was slightly decreased when rice residue was added. Breaking force and deformation of gels were significantly decreased with more than 1% rice residue addition (p < 0.05). The trends of storage modulus, tan δ, and interactions demonstrated a negative effect of rice residue (more than 1%) on gel network. The addition of rice residue reduced the interactions in surimi gel network, such as hydrophobic interaction. The sodium dodecyl sulfate–polyacrylamide gel electrophoresis indicated that there was little interaction between rice proteins and myofibrillar proteins. Rice residue deteriorated the freeze-thaw stability of surimi gels. Therefore, rice residue could be an inactive filler in the gel network unless it was less than 1%.
Introduction
Surimi, obtained from fish through heading, gutting, mincing, washing, dewatering, refining, and blending with cryoprotectants, is a concentrate of high-quality myofibrillar proteins as an intermediate product.[1] The washing process, which could concentrate the water-insoluble myofibrillar proteins such as myosin, actin, and the actomyosin complex,[Citation2] can remove the impurities and water-soluble sarcoplasmic proteins responsible for organoleptic properties. Generally, heat-induced gelation of protein is considered as an important route to obtain the desired properties of surimi-based products. It is a complex physicochemical process involving functional and structural changes of myofibrillar proteins. During gelation, fish proteins unfold and the functional groups expose to form a three-dimensional network via intra- and intermolecular bonds between myofibrillar proteins.[Citation3] Ionic linkage, hydrogen bond, hydrophobic interaction, and covalent bond are formed in the process of protein gelation.[Citation4] Intermolecular hydrophobic interaction and disulfide bond are a primary mechanism of the formation of surimi gel.[Citation4,Citation5]
Recently, nonmeat protein additives, such as whey protein concentrates,[Citation6] egg white,[Citation7] and leguminous extracts protein[Citation8,Citation9] were added into surimi-based products to improve their qualities. The addition of protein additives usually has three major functional advantages in surimi-based seafoods: (1) enzyme inhibition, (2) improvement of gel elasticity and firmness, and (3) anti-retrogradation during frozen storage.[Citation10] Rice residue (RR) has high-nutritive qualities among cereal proteins for the high content of lysine[Citation11] and also has specially unique hypoallergenic properties.[Citation12] It is a by-product from rice starch syrup production and contains abundant rice proteins (50–70%). However, it is usually used as fertilizer or animal feed, leading to the waste of high-quality proteins. Moreover, in China, the resource of RR is so abundant that its price is inexpensive. Besides, RR could be potentially served as food ingredients in food industry.[Citation13]
Silver carp (Hypophthalmichthys molitrix) is the second large production of freshwater fish with 3.85 million tons in China,[Citation14] but its market value is very low. Therefore, silver carp is used to produce surimi as value-added processing in China. Therefore, the objective of this study was to investigate the effects of RR on the physicochemical properties of silver carp surimi gels.
Materials and methods
Materials
Silver carp surimi (AAA grade) was purchased from the Jinli Fishery Food Co., Ltd. (Honghu, Hubei, China) and stored at −21°C until use. The protein content of surimi was determined as 15.37%, the moisture content was 76.45%, and the remaining contents included cryprotectant (sucrose), sodium pyrophosphate, and disodium hydrogen phosphate. RR (Jindege Co. Ltd., Wuhan, Hubei, China) was freeze-dried at about 0.35 mbar and −34°C for 24 h (Christ Beta 2-8 LD plus; Marin Christ, Co., Ltd, Osterode, Germany) and then stored at −21°C until use. Additionally, RR had 8.94% fat, 4.69% ash, 28.05% fiber, and 58.32% protein, which included albumins (1.29%), prolamin (1.77%), globulins (3.26%), glutelin (25.94%), and other proteins (26.06%). β-Mercaptoethanol (β-ME) and protein markers were purchased from Sigma Chemical Co. (St. Louis, MO, USA). N,N,Nʹ,Nʹ-Tetramethyl ethylene diamine, sodium dodecyl sulfate (SDS), coomassie blue R-250, and other chemicals for electrophoresis were obtained from Bio-Rad Laboratories (Hercules, CA, USA).
Preparation of surimi gels
Frozen silver carp surimi was partially thawed at 4°C for 5 h, cut into pieces with proper thickness of 3 cm, and then chopped in a food processor (HR7625, Philips, Hong Kong, China) for 2 min, followed by chopping for another 1 min with the addition of NaCl (2.5%). RR at different levels (0, 0.5, 1.0, 1.5, and 2.0%, w/w) was added to the surimi paste. Ice water was added to adjust the final moisture content of the surimi paste to 80% and maintain the temperature in the range of 4–10°C during the process. The mixture was homogenized for 3 min. Then, the surimi paste was stuffed into Krehalon casings (the diameter 2.0 cm and the length 20 cm) and both ends were sealed tightly. The surimi gels were prepared by incubating at 40°C for 30 min and followed by heating at 90°C for 20 min in a water bath (HH-4; Changzhou Guohua Electric Co., Ltd, Jintan, Jiangsu, China). Subsequently, all gels were cooled in ice water for 20 min and stored at 4°C overnight for further analysis.
Freeze-thaw processing
Freeze-thaw processing was applied on surimi gels without and with RR (1%, w/w) prepared as mentioned earlier. The gels were subjected to five freeze-thaw cycles. Each freeze-thaw cycle was carried out by storing the gels at −21°C for 7 days and thawing at 4°C for 12 h. The freeze-thaw stability of gels was determined by measuring textural changes (puncture test).
Color evaluation
The color values of surimi gel samples were detected by a HunterLab Ultra Scan XE colorimeter (Hunter Lab Co., Ltd, Reston, VA, USA). Lightness (L*), redness (a*) and yellowness (b*) values were recorded. The whiteness (W) of samples was calculated according to the following equation[Citation15]:
Water-holding capacity
Water-holding capacity (WHC) of surimi gels was determined by Avanti J-E Centrifuge (Beckman Coulter Inc., Fullerton, CA, USA). Surimi sample (2 ± 0.2 g, W1) was placed between two layers of filter paper (No. 102φ = 11 cm; Hangzhou Xinhua, Filter Paper Co., Ltd, Hangzhou, Zhejiang, China), and then transferred into the bottom of 50 mL centrifuge tube and centrifuged at 3000 × g for 15 min at 4°C. After centrifugation, filter papers were removed and gels were weighed again (W2). WHC was calculated from the following equation:
Puncture test
Puncture test of surimi gel was carried out using a texture analyzer (TA-XT2i; Stable Micro Systems, Surrey, UK). After equilibrating at room temperature for 2 h, surimi samples were cut into cylinders (20 mm in height) before the puncture test. Surimi samples were placed in the texture analyzer equipped with a spherical probe (P/0.25 s) to penetrate 15 mm into the samples at a speed of 1 mm/s. Breaking force (g) and deformation (mm) were obtained.
Dynamic rheological properties
Dynamic rheological properties of surimi sols with or without RR were measured by an AR2000ex Rheometer (TA Instruments, New Castle, DE, USA) equipped with a 40 mm parallel steel plate in oscillation mode. Gap was set at 1.0 mm and silicone oil was used to prevent water evaporation during the experiment. Stress and frequency sweep tests were conducted before measurement in order to obtain the linear viscoelastical region for the dynamic analysis. To program the temperature sweep, the surimi samples were heated from 20°C to 90°C at a rate of 1°C/min with 1% strain and 1 Hz frequency, which were in the linear range. Storage modulus (G′) and loss tangent (tan δ) values were directly recorded.
Sodium dodecyl sulfate–polyacrylamide gel electrophoresis (SDS–PAGE)
Protein patterns of surimi gels with or without RR were analyzed by SDS–PAGE according to the method of Laemmli.[Citation16] To prepare the protein sample, the gel sample (3 ± 0.05 g) was added with 27 mL of 50 g/L SDS (85°C) solution and homogenized at speed of 10,000 × g for 2 min. The mixed solutions were incubated at 85°C in a water bath for 1 h to dissolve proteins. Then, the sample was centrifuged at 10,000 × g for 15 min to remove undissolved debris. The bovine serum albumin was used as standard to measure the protein concentration within the supernatant according to the method of Lowry.[Citation17] The sample was diluted with water to equilibrate the protein concentration to 1.0–1.5 mg/mL. The samples (15 μg) were loaded onto the polyacrylamide gel made of 5% stacking gel and 10% running gel and subjected to electrophoresis at a constant current of 15 mA per gel, using a Mini Protein II unit (Bio-Rad Laboratories, Inc., Hercules, CA, USA). After separation, the proteins were stained with 0.2 g/L coomassie brilliant blue R-250 in 75 mL/L acetic acid and 500 mL/L methanol and destained with 75 mL/L acetic acid and 500 mL/L methanol, followed by 75 mL/L acetic acid and 50 mL/L methanol.
Determination of gel solubility
Solubility of surimi gels with different concentrations of RR in a number of selected solutions was conducted to determine ionic bond, hydrogen bond, hydrophobic interaction, and disulfide bond according to the method of Gómez-Guillén and others.[Citation18] The selected solutions were as follows: 0.05 mol/L NaCl (SA), 0.6 mol/L NaCl (SB), 0.6 mol/L NaCl + 1.5 mol/L urea (SC), 0.6 mol/L NaCl + 8 mol/L urea (SD) and 0.6 mol/L NaCl + 8 mol/L urea + 0.5 mol/L β-ME solutions. Ionic bond was the difference between protein solubilized in SB and protein solubilized in SA; hydrogen bond was the difference between protein solubilized in SC and protein solubilized in SB; hydrophobic interaction was the difference between protein solubilized in SD and protein solubilized in SC, and disulfide bond was the difference between protein solubilized in SE and protein solubilized in SD. The protein concentration was determined by the method of Lowry.[Citation17]
Scanning electron microscopy (SEM)
Microstructure of surimi gels was determined using the SEM. Surimi gels without or with 1.0% and 2.0% RR (thickness of 2–3 mm) were fixed with 25 mL/L glutaraldehyde in 0.2 mol/L phosphate buffer (pH = 7.2). Then, the samples were rinsed for 1 h in distilled water before being dehydrated in ethanol with a serial concentration of 300, 500, 700, 800, 900, and 1000 mL/L for 15 min. Dried samples were mounted on a bronze stub and sputter-coated with gold (IB-3, EIKO Co., Ltd, Japan). The specimens were observed with SEM (JEOL JSM-6300 PLV, Tokyo, Japan) at an acceleration voltage of 20 kV.
Statistical analysis
ANOVA was performed using SPSS Package (SPSS 17.0 for Windows; SPSS Inc., Chicago, IL, USA). Differences among the mean values of various treatments were measured by Duncan’s multiple range test (p < 0.05). All experiments were replicated at least three times.
Results and discussion
Color evaluation
illustrates the effects of RR on the color attributes of silver carp surimi gels. The addition of RR slightly changed the color of surimi gels (p < 0.05). Compared with the control (without RR), the L* value of surimi gels with RR was slightly decreased (p < 0.05), while the b* and a* values significantly increased. The results suggested that there was a change from slightly greenish in the control to slightly reddish associated with an increase in the yellowish with the increasing content of RR. In general, the variety and amount of additives have the primary effects on the color properties of surimi gels.[Citation19,Citation20] Therefore, the slight reduction in whiteness of surimi gels was mainly due to the pale straw color of RR.
Table 1. Effects of rice residue (RR) contents on the color properties of surimi gels.
Water-holding capacity
WHC can reflect the strength of gel network. presents the effects of RR at different contents on WHC of silver carp surimi gels. There was a slight loss in WHC of the gels with the increasing contents of RR in this study (p < 0.05). Generally, surimi gel forms a continuous three-dimensional network during thermal gelation and holds water in its network.[Citation21] The decrease in WHC in surimi gels containing RR could be associated with a lower WHC of RR, comparing to myofibrillar proteins. RR might probably cause a disruptive effect on the gel structure. In addition, a relatively lower proportion of protein in the gels with RR might also cause the decrease in WHC. The result was also in accordance with insoluble solids including proteins from surimi wash water lowering WHC of pacific whiting surimi.[Citation22]
Gel strength
presents the effects of RR at different contents on breaking force and deformation of silver carp surimi gels. When 0.5% content of RR was added into surimi gels, there was no difference in breaking force and deformation of gels, comparing to the control. However, when more RR was added, surimi gels displayed significantly lower breaking force than the control (p < 0.05), while there was a similar trend in deformation. As RR content increased, the proportional reduction of myofibrillar protein content in the gel samples was an important factor in the decrease of breaking force and deformation. For example, the content of fish protein in the control was 13.07%, while the gel with 2.0% RR had only 12.05% fish protein. Besides, it might also be related with the disruption of the surimi gel matrix due to the higher insolubility of RR in the surimi system. There were over 40% nonprotein compounds in RR, such as fat and fiber, which might not enhance but weaken the gel structure of fish protein. The result was consistent with other studies. For example, the loss of gel strength of surimi gels with nonmuscle protein additives could be caused by “dilution” of myofibrillar proteins.[Citation23] In addition, the insoluble wheat dietary fiber[Citation24] and insoluble solid from surimi wash water[Citation22] also lower the functional and mechanical properties of giant squid (Dosidicus gigas) and pacific whiting surimi gels, respectively.
Rheological properties
demonstrates the effects of RR content on the storage modulus (Gʹ) and loss tangent (tan δ) of silver carp surimi gels during heating from 20°C to 90°C. The Gʹ generally relates to the elasticity, which reflects the heat-induced gel-forming ability of food proteins.[Citation25,Citation26] In the control, Gʹ increased from 20°C and reached the first peak at approximately 31°C, followed by rapidly decreasing to the lowest value at about 53°C. Subsequently, Gʹ moderately increased again up to 90°C. Broadly, the first peak indicated the cross-linking among protein molecules and the formation of preliminary protein network structure through hydrogen bond at low temperature.[Citation27,Citation28] The downtrend of Gʹ during the temperature ranging from 31°C to 53°C might be associated to the structural disintegration of fish protein, due to the helix-to-coil transformation of myosin. It might disrupt the formation of protein network to some extent and leaded to a large increase in the fluidity of the semi-gel.[Citation29] As the temperature rises further from 53°C to 90°C, the second increase was observed in Gʹ, which might symbolize the formation of a highly elastic stable protein gels due to continuous protein aggregation. These results were similar to the rheological properties of Alaska Pollock paste[Citation30] and actin–myosin (AM) solution from silver carp.[Citation31]
Figure 3. The effects of rice residue at different contents on the storage modulus (Gʹ) and loss tan (tan δ) of surimi pastes during thermal gelation.
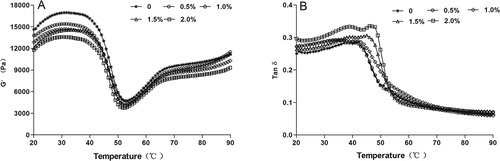
The G′ of paste samples with RR displayed the similar trend to the control, but a decrease of Gʹ values was observed, especially at temperatures ranging 20–31°C. It implied that RR, especially at the high content, weakened the hydrogen bond among myofibrillar proteins during the initial thermal gelation. The Gʹ generally reflects the heat-induced gel-forming ability of fish proteins, so the result might be attributed to the damaging and weakening effect of RR on fish protein gel-forming ability. However, comparing with the control, there was a delay in the second increase of Gʹ, especially in higher RR content. It might probably be caused by RR that mildly alleviated the dissociation of AM through serving as functional filler infiltrating in surimi gel network. Soy protein isolates could postpone the development of Modori phenomena of bighead carp surimi via retarding the disulfide bridge and hydrophobic interaction formation among the myofibrillar protein.[Citation32]
The tan δ (Gʺ/G′) of all surimi gels was less than 1 (), which indicated that surimi gels were more elastic than viscous. The tan δ pattern of surimi sol with/without RR showed an increase from 30°C to 45°C and a sharp drop from 45°C to 90°C. The initial increase was due to the fact that an increased structural “bulkiness” of myosin during the initial heating produces a viscous sol.[Citation33] During the heating from 45°C to 90°C, more molecular interactions and cross-linking were formed to increase the elasticity,[Citation34] leading to a decrease in tan δ. There was a gradual increase in the tan δ of surimi sols with RR before 45°C. It indicated that RR decreased the elasticity of surimi gel and increased the viscosity. However, comparing with the control, there was also a delay for the sharp drop in tan δ, especially in the higher RR content, similar to the patterns of G′. The result further verified that RR had an intervening effect on the gel-forming ability during the gelation of surimi proteins. Besides, these results were consistent with WHC () and gel strength ().
Effect of RR on protein pattern of surimi gels
Surimi gels were analyzed using the SDS–PAGE (), in order to clarify the effect of RR on the cross-linking of myosin heavy chains (MHC) during gel formation. The result displayed that MHC was the major protein band, followed by actin in all surimi samples. As more RR was added in the surimi gels, the band intensities were reduced, especially in the higher RR content. It indicated the dilution effect of RR on surimi gels. It also implied that there was few interaction or association formed between rice proteins and myofibrillar proteins. Generally, denaturation temperatures of nonmuscle protein and muscle protein are dramatically different; so, the two types of proteins form mixing gels via inter-infiltration rather than protein–protein aggregation.[Citation35] For example, wheat gluten was usually used as functional filler in surimi-based products since the insolubility of gluten protein made it difficult to form a possible interaction with myofibrillar proteins.[Citation26,Citation36] RR contains a large number of glutelin (25.94%) and other insoluble compounds (i.e., fiber). Therefore, RR might act as a functional filler to inter-infiltrate with surimi proteins to change the properties of surimi gels rather than inducing the cross-linking or interacting with myofibrillar proteins. Therefore, surimi gels with a higher RR content had lower gel strength than the control ().
Protein solubility: disruption of bonds
shows the protein solubility of gels treated with RR at different concentrations selected for their capacity to disrupt certain kinds of bonds. The highest value was hydrophobic interaction in all samples. It implied that hydrophobic interaction might be the major and most important bond in surimi gels. Similar results were reported that hydrophobic interaction was higher than other bonds in surimi gels.[Citation36,Citation37] In general, hydrophobic interaction plays an important role to strengthen surimi gels for its association of the hydrophobic portions of neighboring proteins. Besides, the process of heating might induce the unfolding of native protein and the exposure of buried polar groups; as a result, protein–protein interaction strengthened by hydrophobic association.[Citation4] However, as the addition of RR, hydrophobic interaction was decreased in surimi gels, except at 0.5% content of RR. It might be due to the negative effects of the RR on cross-linking of AM, resulting in weakening the surimi gel network.
Figure 5. The effects of rice residue contents on chemical bonds of surimi gels. Different letters on the bars within the same bar indicate significant differences (p < 0.05).
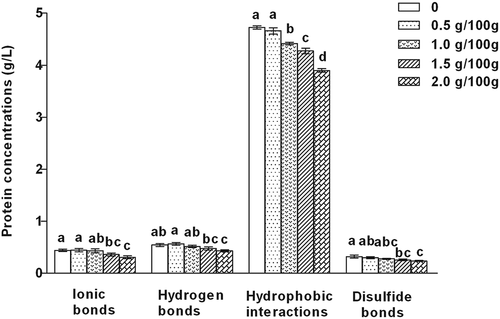
Compared with the control, ionic bond slightly decreased in surimi gel when the content of RR was over 1%. It might probably be due to higher insolubility of RR, which prevented the formation of ionic bonds in surimi gels, especially at higher content of RR. Like ionic bond, there was no significant change (p > 0.05) in hydrogen bond of surimi gels with 0.5% and 1.0% RR, except with over 1.0%. When RR was below 1.0% in surimi gels, it might be still able to imbibe a portion of water to compensate for the decrease of cross-linking between fish protein and water. However, when RR was over 1.0%, the negative effect was increased, resulting in the lower hydrogen bond content in surimi gels. The result was in accordance with the trends of WHC (), G′ and tan δ () of surimi gels with RR. Comparing with the control, disulfide bond did not change much in surimi gels with RR up to 1.0%, but followed a slight decrease with RR over 1.0%. When more RR was added, fish proteins or myofibrillar proteins in surimi gels were relatively lower than the control. Therefore, it reduced the cross-linking of protein–protein by lowering disulfide bond content in surimi gels. In general, reducing interactions in surimi gels with RR, especially hydrophobic interaction, also indicated the change patterns of breaking force and deformation ().
Microstructure
shows the microstructure of silver carp surimi gels without and with RR. A large porous matrix with some cavities was visualized in all surimi gels. However, surimi gels without RR showed a well-structured matrix with a highly interconnected network of strands and fine three-dimensional protein network, while surimi gels with RR showed more uneven and incompact gel network. It might be due to the negative effect of RR on cross-linking of myofibrillar protein, consequently, disrupting the surimi gel matrix. This result was also coincidental with decreasing breaking force and deformation of surimi gel added with RR (). However, it was different from the trends of other nonmuscle proteins added into surimi gels. Comparing with control, the protein network of kamaboko gel with 3% whey protein (WP),[Citation6] 1% bambara groundnut protein isolates (BGPI), and mungbean protein isolates (MGPI)[Citation8] seemed to be more compacted with smaller clusters of aggregated protein. Such difference might be attributed to higher insolubility of RR, but WP, BGPI, and MGPI had much higher solubility than RR.
Freeze-thaw stability
Freeze-thaw processing could cause quality deterioration of fish muscle.[Citation38] presents textural parameters of silver carp surimi gels with and without 1% RR after five freeze-thaw cycles. All surimi gels showed the decreases in both breaking force and deformation values when the freeze-thaw cycles increased (p < 0.05). During freezing, ice crystals were formed from intracellular or extracellular water, resulting in mechanical damage caused by irregular ice crystals and disrupting the three-dimensional network structure of surimi gels.[Citation38] The formation of ice crystals increased the concentration of surimi gel system, changed the ionic strength, and destroyed the protein structure. Meanwhile, it changed the combination state of protein and water molecules.[Citation39] As the freeze-thaw cycles increase, the gel network structure was further disrupted and lead to the decrease in both breaking force and deformation of gels.
Figure 7. Effects of rice residue (RR) on breaking force and deformation of surimi gels during freeze-thaw cycles. Different letters indicate significant differences (p < 0.05). Uppercase indicates the significant differences among different freeze-thaw cycles in the same surimi gel, while lowercase indicates the significant differences among surimi gels with or without RR in the same freeze-thaw cycle.
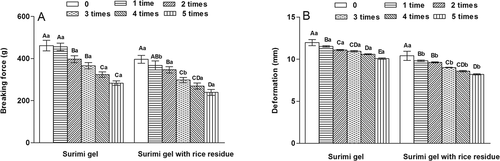
For the same cycle, deformation in gels with RR was much lower than those without RR (p < 0.05), while breaking force was lower in the gels with RR in the first two freeze-thaw cycles. It may also be related with the disruption of the surimi gel matrix for higher insolubility of RR in the surimi system; thus, resulting in the decrease of breaking force and deformation. After two freeze-thaw cycles, there was no significant difference in breaking force between the surimi gels with or without RR, probably due to the fact that further freeze-thaw processing could weaken more surimi gel structure.[Citation40,Citation41] In general, RR deteriorated the freeze-thaw stability of surimi gels.
Conclusions
In general, the addition of RR into silver carp surimi gels resulted in slight reduction in the whiteness. When RR was less than 1% in surimi gels, there was not much change in the textural parameters in surimi gels. However, when more RR was added, there were significant decreases in breaking force and deformation. The lower Gʹ and higher tan δ also confirmed such decreases in gel structure. The major interaction was hydrophobic interaction in surimi gels with or without RR. Reducing interactions in surimi gels with RR, especially hydrophobic interaction, also indicated the change pattern of both characteristics. The SDS–PAGE suggested that there was little interaction between rice proteins and surimi proteins. The addition of RR deteriorated the freeze-thaw stability of surimi gels. Therefore, RR could be an inactive filler in the gel network unless its content was less than 1%. There is a need to further isolate proteins from RR in order to enhance its filler functionality, especially for the application of surimi-based seafood.
Additional information
Funding
References
- Yin, T.; Park, J. W. Effects of Nano-Scaled Fish Bone on the Gelation Properties of Alaska Pollock Surimi. Food Chemistry 2014, 150, 463–468.
- Ramírez, J. A.; Martín-Polo, M. O.; Bandman, E. Fish Myosin Aggregation as Affected by Freezing and Initial Physical State. Journal of Food Science. 2000, 65(4), 556–560.
- Yoon, W. B.; Gunasekaran, S.; Park, J. W. Characterization of Thermorheological Behavior of Alaska Pollock and Pacific Whiting Surimi. Journal of Food Science. 2004, 69(7), 338–343.
- Lanier, T. C.; Carvajal, P.; Yongsawatdigul, J. Surimi Gelation Chemistry. In Surimi and Surimi Seafood, 2nd ed.; Park, J. W.; Editor.; Taylor and Francis Group: Boca Raton, FL, 2005; 435–477.
- Kishi, A.; Itoh, Y.; Obatake, A. The Polymerization of Protein through Disulfide during the Heating of Carp Myosin. Nippon Suisan Gakkaishi. 1995, 61(1), 75–80.
- Rawdkuen, S.; Benjakul, S. Whey Protein Concentrate: Autolysis Inhibition and Effects on the Gel Properties of Surimi Prepared from Tropical Fish. Food Chemistry. 2008, 106(3), 1077–1084.
- Campo-Deaño, L.; Tovar, C. The Effect of Egg Albumen on the Viscoelasticity of Crab Sticks Made from Alaska Pollock and Pacific Whiting Surimi. Food Hydrocolloids. 2009, 23(7), 1641–1646.
- Kudre, T.; Benjakul, S.; Kishimura, H. Effects of Protein Isolates from Black Bean and Mungbean on Proteolysis and Gel Properties of Surimi from Sardine (Sardinella albella). LWT – Food Science and Technology. 2013, 50(2), 511–518.
- Luo, Y.; Shen, H.; Pan, D.; Bu, G. Gel Properties of Surimi from Silver Carp (Hypophthalmichthys Molitrix) as Affected by Heat Treatment and Soy Protein Isolate. Food Hydrocolloids 2008, 22(8), 1513–1519.
- Park, J. W.;. Ingredient Technology for Surimi and Surimi Seafood. In Surimi and Surimi Seafood, 2nd ed.; Park, J. W.; Editor.; Taylor and Francis Group: Boca Raton, FL, 2005; 649–702.
- Shih, F.F.; Champagne, E.T.; Daigle, K.; Zarins, Z. Use of Enzymes in the Processing of Protein Products from Rice Bran and Rice Flour. Nahrung 1999, 43(1), 14–18.
- Helm, R. M.; Burks, A. W. Hypoallergenicity of Rice Protein. Cereal Foods World. 1996, 41, 839–843.
- Anderson, A.; Hettiarachchy, N.; Ju, Z. Y. Physicochemical Properties of Pronase-Treated Rice Glutelin. JAOCS. 2001, 78(1), 1–6.
- Chinese Fishery Statistics Yearbook; China Agriculture Press: Beijing, 2014.
- Park, J. W.;. Functional Protein Additives in Surimi Gels. Journal of Food Science. 1994, 59(3), 525–527.
- Laemmli, U. K.;. Cleavage of Structural Proteins during the Assembly of the Head of Bacteriophage. Nature. 1970, 227(5259), 680–685.
- Lowry, O.H.; Rosebrough, N.J.; Farr, L.A.; Randall, R.J. Protein Measurement with the Folin Phenol Reagent. Journal of Biological Chemistry 1951, 193(1), 265–275.
- Gómez-Guillén, M. C.; Borderı́as, A.; Montero, P. Chemical Interactions of Nonmuscle Proteins in the Network of Sardine (Sardina pilchardus) Muscle Gels. LWT – Food Sciences Technological. 1997, 30(6), 602–608.
- Benjakul, S.; Visessanguan, W.; Chantarasuwan, C. Effect of Porcine Plasma Protein and Setting on Gel Properties of Surimi Produced from Fish Caught in Thailand. LWT – Food Science and Technology. 2004, 37(2), 177–185.
- Rawdkuen, S.; Benjakul, S.; Visessanguan, W.; Lanier, T.C. Effect of Chicken Plasma Protein and Some Protein Additives on Proteolysis and Gel-Forming Ability of Sardine (Sardinella gibbosa) Surimi. Journal of Food Processing and Preservation 2007, 31(4), 492–516.
- Liu, R.; Zhao, S.M.; Xiong, S.B.; Xie, B.J.; Qin, L.H. Role of Secondary Structures in the Gelation of Porcine Myosin at Different pH Values. Meat Science 2008, 80(3), 632–639.
- Ramirez, J.A.; Velazquez, G.; Echevarria, G.L.; Torres, J.A. Effect of Adding Insoluble Solids from Surimi Wash Water on the Functional and Mechanical Properties of Pacific Whiting Grade A Surimi. Bioresource Technology 2007, 98(11), 2148–2153.
- Burgarella, J.C.; Lanier, T.C.; Hamann, D.D.; Wu, M.C. Gel Strength Development during Heating of Surimi in Combination with Egg White or Whey Protein Concentrate. Journal of Food Science 1985, 50(6), 1595–1597.
- Sánchez-Alonso, I.; Solas, M. T.; Borderías, A. J. Technological Implications of Addition of Wheat Dietary Fibre to Giant Squid (Dosidicus gigas) Surimi Gels. Journal of Food Engineering. 2007, 81(2), 404–411.
- Karthikeyan, M.; Dileep, A. O.; Shamasundar, B. A. Effect of Water Washing on the Functional and Rheological Properties of Proteins from Thread Fin Bream (Nemipterus japonicas) Meat. International Journal of Food Science and Technology. 2006, 41(9), 1002–1010.
- Kim, B. Y.; Park, J. W.; Yoon, W. B. Rheology and Texture Properties of Surimi Gels. In Surimi and Surimi Seafood, 2nd ed.; Park, J. W.; Editor.; Taylor and Francis Group: Boca Raton, FL, 2005; 491–576.
- Cao, M.J.; Wu, L.L.; Hara, K.J.; Weng, L.; Su, W.J. Purification and Characterization of a Myofibril-Bound Serine Proteinase from the Skeletal Muscle of Silver Carp. Journal of Food Biochemistry 2005, 29(5), 533–546.
- Egelandsdal, B.; Fretheim, K.; Samejima, K. Dynamic Rheological Measurements on Heat-Induced Myosin Gels: Effect of Ionic Strength, Protein Concentration and Addition of Adenosine Triphosphate or Pyrophosphate. Journal of the Science of Food and Agriculture. 1986, 37(9), 915–926.
- Nakagawa, T.; Watabe, S.; Hashimoto, K. Electrophoretic Analysis of Sarcoplasmic Protein from Fish Muscle on Polyacrylamide Gels. Nippon Suisan Gakkaishi 1988, 54, 993–998.
- Taskaya, L.; Chen, Y. C.; Jaczynski, J. Functional Properties of Proteins Recovered from Whole Gutted Silver Carb (Hypophthalmichthys molitrix) by Isoelectric Solubilization/Precipitation. Journal of the Science of Food and Agriculture. 2009, 89(2), 349–358.
- Liu, R.; Zhao, S.; Xiong, S.B.; Qiu, C.; Xie, B.J. Rheological Properties of Fish Actomyosin and Pork Actomyosin Solutions. Journal of Food Engineering 2008, 85(2), 173–179.
- Luo, Y. K.; Pan, D. D.; Ji, B. P. Gel Properties of Surimi from Bighead Carp (Aristichthys nobilis): Influence of Setting and Soy Protein Isolate. Journal of Food Science. 2004, 69(8), 374–378.
- Wu, M.; Xiong, Y.L.; Chen, J.; Tang, X.; Zhou, G. Rheological and Microstructural Properties of Porcine Myofibrillar Protein-Lipid Emulsion Composite Gels. Journal of Food Science 2009, 74(4), E207–E217.
- Fukushima, H.; Okazaki, E.; Fukuda, Y.; Watabe, S. Rheological Properties of Selected Fish Paste at Selected Temperature Pertaining to Shaping of Surimi-Based Products. Journal of Food Engineering 2007, 81(2), 492–499.
- Lanier, T. C.; Functional Properties of Surimi. Food Technology. 1986, 40, 107–112.
- Gómez-Guillén, M. C.; Borderías, A. J.; Montero, P. Chemical Interactions of Nonmuscle Proteins in the Network of Sardine (Sardina pilchardus) Muscle Gels. LWT – Food Science and Technology. 1997, 30(6), 602–608.
- Liu, R.; Zhao, S.M.; Xie, B.J.; Xiong, S.B. Contribution of Protein Conformation and Intermolecular Bonds to Fish and Pork Gelation Properties. Food Hydrocolloids 2011, 25(5), 898–906.
- Benjakul, S.; Bauer, F. Biochemical and Physicochemical Changes in Catfish (Silurus Glanis Linne) Muscle as Influenced by Different Freeze-Thaw Cycles. Food Chemistry 2001, 72, 207–217.
- Ramiarez, J. A.; Martian‐Polo, M. O.; Bandman., E. Fish Myosin Aggregation as Affected by Freezing and Initial Physical State. Journal of Food Science. 2000, 65(4), 556–560.
- Kingwascharapong, P.; Benjakul, S. Effect of Phosphate and Bicarbonate Replacers on Quality Changes of Raw and Cooked Pacific White Shrimp as Influenced by the Repeated Freeze–Thawing. International Journal of Refrigeration 2016, 67, 345–354.
- Xia, X.; Kong, B.; Liu, Q.; Liu, J. Physicochemical Change and Protein Oxidation in Porcine Longissimus dorsi as Influenced by Different Freeze-Thaw Cycles. Meat Science 2009, 83(2), 239–245.