ABSTRACT
This article presents a study on fruiting bodies of Boletus badius, Boletus edulis, Cantharellus cibarius, Lactarius deliciosus, Leccinum scabrum, Suillus bovinus, Suillus luteus, Pleurotus ostreatus, Tricholoma equestre, Armillaria mellea, Agaricus bisporus, and Auricularia polytricha. The amounts of their health-promoting bioelements and physiologically significant indole and phenolic compounds were determined. The significance of edible mushrooms in the human diet and in disease prevention were also established. The high-performance liquid chromatography was used to assess the contents of indole compounds and phenolic acids. The atomic absorption spectroscopy was applied to determine bioelements. In all samples, the content of 5-hydroxy-L-tryptophan was studied. The highest extraction amount of serotonin was found for P. ostreatus species. Phenolic compounds were determined in the conditions described in the present study for the first time. Additionally, protocatechuic, p-hydroxybenzoic, syringic, and gallic acids were determined.
Introduction
The fruiting bodies of 12 edible mushrooms were studied, including wild and commercially cultivated species. These species were selected based on: (1) dietary value, (2) therapeutic properties, (3) consumption frequency, and (4) authorization for sale in Poland. For example, Boletus edulis is a rich source of selenium and tocopherol with strong antioxidant properties.[Citation1–Citation3] This species contains essential amino acids similar to animal foods. In addition, B. edulis contains very high levels of fatty acids such as oleic acid, linoleic acid, and palmitic acid, and sterols such as ergosterol, ergosta-7,22-dienol, ergosta-5,7-dienol, and ergosta-7-enol. The highest amounts of phenolic compounds such as protocatechuic acid, p-hydroxybenzoic acid, p-coumaric acid, and cinnamic acid with strong antioxidant and anticancer properties[Citation5] were found in B. badius species.[Citation3,Citation4] In turn, Cantharellus cibarius has the highest content of vitamins B, A, E, and C––comparable to baking yeasts––and ergocalciferol (vitamin D2) and β-carotene.[Citation3,Citation4,Citation6,Citation7]
Tricholoma equestre is an edible species that is available commercially and is characterized by its high content of sodium and dry weight. Currently, this species is under study for its toxic effect on muscle tissue, as several people have been hospitalized after consuming it.[Citation8] Leccinum scabrum is a good source of minerals. Dry extracts of birch bolete fruiting bodies demonstrate antiulcer and anticancer properties. Pleurotus ostreatus was first cultivated for culinary purposes during the First World War in Germany and has been classified as a medicinal mushroom, as it contains lovastatin, which is a hypolipidemic drug used in the treatment of the circulatory system, heart diseases, and stroke.[Citation9,Citation10] It also contains pleuran––a polysaccharide with anticancer properties.[Citation11,Citation12] The first information about Agaricus bisporus cultivation appeared at France in 1707. Agaricus bisporus has antioxidant properties due to the presence of phenolic compounds in the fruiting bodies, and ergothioneine––a derivative of histidine. Ergothioneine has antioxidant, antimutagenic, chemo-, and radio-protective roles in cells. Agaricus bisporus is also a valuable source of vitamins, especially of riboflavin and ergocalciferol (successful experiments have been conducted by exposing the fruiting bodies of vitamin D2-enriched mushrooms to ultraviolet [UV]-B), and minerals such as selenium, magnesium, copper, potassium, iron, calcium, silver, and zinc.[Citation5,Citation13]
Lactarius deliciosus is rich in oligosaccharides with anticancer activity. This activity probably results from the stimulation of the immune mechanisms of an organism.[Citation14,Citation15] Lactarius deliciosus is also a good source of indole compounds, including serotonin, possessing an antidepressant effect and regulating intestinal function.[Citation16] Suillus bovinus exhibits antioxidant properties, mainly due to the presence of phenolic compounds.[Citation17] Also, S. luteus is a rich source of antioxidant compounds, and extracts derived from this species possess antimicrobial activity and are effective against both gram-negative and gram-positive bacteria.[Citation2,Citation18] Auricularia polytricha, known as “black fungus,” is a tree fungus observed in tropical areas and it is well known for its enhancing of properties of the immune system. An amyloid precursor protein that stimulates the immune system has been isolated from this species.[Citation19] Armillaria mellea contains significant amounts of indole compounds (e.g., serotonin, l-tryptophan, and 5-hydroxy-l-tryptophan) used in the treatment of mood disorders and depression.[Citation16]
The special feature that distinguishes the fruiting bodies of mushrooms from other organisms is their ability to collect and accumulate organic and inorganic compounds (especially metals) from the environment. Metallothioneins present in the fruiting bodies are responsible for the accumulation of chemical elements. A large number of publications are available regarding the content of organic compounds and metals in the fruiting bodies of mushrooms, but there are no data indicating on their release from such material.[Citation3,Citation4,Citation20] Therefore, the aim of this study was to transfer and adapt the methodology for mushroom material extraction in artificial digestive juices, namely in conditions similar to those that prevail in the human digestive tract at a temperature of 37°C. Also, we determined the number of selected health-promoting elements and physiologically important indole and phenolic compounds released from edible mushrooms to the digestive juices. Examination of the release and bioavailability, as well as the content of constituents of the mushroom fruiting bodies may be important in human disease prevention. This is the first comprehensive study on determination of the significance of mushrooms in human diet supplementation with physiologically important components.
Materials and methods
Mushroom material
The fruiting bodies of the following wild edible mushrooms were selected for the study: Boletus badius (Fr.) Fr. (Bay bolete), Boletus edulis Bull. (King bolete), Cantharellus cibarius Fr. (Chanterelle), Lactarius deliciosus (L. ex Fr.) Gray (Saffron milk cap), Leccinum scabrum (Bull.) Gray (Birch bolete), Suillus bovinus (L.: Fr.) O. Kuntze (Jersey cow mushroom), Suillus luteus (L.) Roussel (Slippery jack), Pleurotus ostreatus (Jacq.) P. Kumm. (Oyster mushroom), Tricholoma equestre (L.) P. Kumm. (Man on horseback), and Armillaria mellea (Vahl) P. Kumm. Honey mushrooms were harvested from their natural state. The study also included the fruiting bodies of edible mushrooms that were commercially cultivated and bought in a market: Agaricus bisporus (J.E. Lange) Imbach (white mushroom) and Auricularia polytricha (Mont.) Sacc. (Jelly fungus). Fruiting bodies of 12 species were bought in a hypermarket or collected from the natural environment in mixed forests of South Poland (close to Nowy Sącz, Piwniczna Zdrój, and Alwernia) between 2013 and 2015. Taxonomic identification of the young sporocarps was made according to online keys (http://www.mycokey.com) and Knudsen and Vesterholt.[Citation21] Representative voucher specimens were deposited at the Department of Pharmaceutical Botany, Jagiellonian University Medical College, Kraków, Poland.
Reagents
MgCl2 was obtained from Chempur (Krakow, Poland); NaCl and NaHCO3 were from PPH Golpharm (Krakow, Poland); pepsin and bile salts were from BTL (Łódź, Poland); CaCl2 was from Pharma Zentrale GmbH (Germany); pancreatic extract, HCl, KCl, HNO3 concentrated, Suprapur®, and KNO3, Suprapur® were obtained from Merck (Darmstad, Germany); C6H8O7, ZnSO4, KHCO3, Na2HPO4, K2HPO4, and NaOH were from Polish Company of Chemistry (Gliwice, Poland); standards of seven indole compounds––5-hydroxy-l-tryptophan, 6-methyl-d,l-tryptophan, l-tryptophan, melatonin, tryptamine, serotonin, and 5-methyl-tryptamine––were from Sigma-Aldrich (St. Louis, MO, USA); all these compounds were of high-performance liquid chromatography (HPLC) grade. Standards of four phenolic acids––syringic acid, gallic acid, protocatechuic acid, and p-hydroxybenzoic acid––were from Sigma-Aldrich (St. Louis, MO, USA). Acetic acid, petroleum, ether, methanol, and dichloromethane were from Merck (Darmstad, Germany). Water (quadruple-distilled) with a conductivity of less than 1 µS/cm was obtained using an S2-97A2 distillation apparatus (ChemLand, Stargard Szczecin, Poland).
Preparation of artificial digestive juice solutions
Artificial saliva (pH = 7.0) was prepared according to Arvidson’s model. This liquid was prepared by mixing the following compounds with fourfold distilled water: 100 mL of 150 mM KHCO3, 100 mL of 25 mM KH2PO4, 100 mL of 24 mM Na2HPO4, 100 mL of 150 mM KHCO3, 100 mL of 100 mM NaCl, 6 mL of 25 mM C6H8O7, 100 mL of 1.5 mM MgCl2, and 100 mL of 15 mM CaCl2. In this model of artificial saliva, no digestive enzymes present in saliva were included.[Citation22] Artificial gastric juice (pH = 2.0) solution was prepared according to Polish Pharmacopeia X. It was prepared by dissolving 3.2 g pepsin and 2.0 g NaCl in quadruple-distilled water. Then, 80 mL of 1 M HCL was added to adjust the pH to 2.0. Quadruple-distilled water was then added to make up the solution to 1000 mL in a measuring flask.[Citation23] Artificial intestinal juice (pH = 8.0) was prepared by mixing bile salts (25 g/L) and 5 mL of pancreatic extract (4 g/L) in 0.1 M NaHCO3 water solution. Quadruple-distilled water was then added to make up to a final volume of 1000 mL.[Citation24]
Material preparation for analysis
The fruiting bodies were cleaned, frozen, and then dried using the lyophilization method (Freezone 4.5. Labconco; –40°C). One gram of processed fruiting bodies was weighed, ground in an agate mortar, and placed in flasks containing 25 mL of artificial saliva. The samples were then incubated in a Gastroel-2014 apparatus at normal human body temperature for 1 min (this is assumed to be the time the food stays in the mouth). The solution was then decanted, and partially digested material was treated with 25 mL of artificial gastric juice. The material was incubated in the gastric juice for 15, 60, and 120 min, in order to simulate the normal residence time of food in the stomach, depending on its type. Incubation of the material in the gastric juice was also conducted at a temperature of 37°C in the Gastroel-2014. After the specified time, the solution was again decanted and 25 mL of artificial intestinal juice was added to the remaining biomass, and the material was incubated in the Gastroel-2014 under the same temperature conditions. The period of digestion in intestinal juice was 150 min and this was the same for all samples. Decanted solutions were each time filtered using membrane filters (Millex, Millipore Corporation, USA) and divided into two equal parts. The contents of elements were examined in one part. The remaining part was subject to evaporation in order to obtain a solid residue. The solid residue was dissolved in 1.5 mL of methanol and transferred using sterile syringes and syringe filters (Millex, Millipore Corporation, USA) to Eppendorf tubes. The content of indole compounds and phenolic acids was determined in the obtained methanol extracts.
The extracts of artificial digestive juices (10 mL) obtained via the digestion of 12 mushroom species were analyzed for the content of selected macro- and microelements. The amounts of these elements were measured directly from the obtained extract using the flame technique for Mg, Fe, Cu, Ca, and Zn and the flame photometry method for K and Na. A Perkin Elmer 3110 atomic absorption spectrometer (USA) was used for all analyses.
Determination of indole compounds
The HPLC method was performed according to the procedure described by Muszyńska.[Citation2,Citation25] HPLC analyses were conducted using a Hitachi apparatus equipped with an L-7100 pump, an L-7400 UV Detector (λ = 280 nm), and a Purospher RP-18e column (250 mm × 4 mm, 5 µm) thermostated at 25°C. The mobile phase was composed of ethanol/water/concentrated acetic acid in a ratio of 1:3.96:0.04 (v/v/v).
Determination of phenolic compounds
The HPLC method was performed according to Ellnain-Wojtaszek and Zgórka.[Citation26] HPLC analyses were conducted using an HPLC VWR Hitachi-Merck apparatus: L-2200 autosampler, L-2130 pump, RP-18e LiChrospher column (250 mm × 4 mm, 5 µm) thermostated at 25°C, L-2350 column oven, and L-2455 diode array detector at UV range 200–400 nm. The mobile phase consisted of solvent A (methanol/0.5% acetic acid 1:4 [v/v]), and solvent B (methanol). The gradient was as follows: 100:0 for 0–25 min, 70:30 for 35 min, 50:50 for 45 min, 0:100 for 50–55 min, and 100:0 for 57–67 min.
Statistical analysis
For each of the samples, three repetitions were used for the determination of every compound and all analyses were conducted in triplicate. The results were expressed as mean values and standard deviations (±SD). Statistical analyses were conducted using Microsoft Excel 2010 and GraphPad InStat Software. Values were considered significantly different at p ˂ 0.05.
Results
The methodology to extract mushroom materials from artificial gastric juices was elaborated. Selected health-promoting bioelement contents (Mg, K, Na, Ca, Cu, Zn, Fe; ) released from 12 species of edible mushrooms into artificial digestive juices were determined, concurrently taking into account their different incubation times in artificial gastric juice (–).The atomic absorption spectrometry method was used for all analyses. All the results (represented as the average of four measurements) were in each case supplemented by ±SD values. The results allowed us to state the relationships between mushroom species, length of incubation time in the gastric juice, and the degree of element release into artificial gastric juices. In addition, it was possible to isolate clusters or groups of objects characterized by a high level of similarity.
Table 1. The content of bioelements in the lyophilized biomass of 12 species of edible mushrooms extracted into artificial digestive juices over different time periods.
Figure 1. A radar chart of metals released into artificial gastric juice for an incubation time of 15 min. (Po, P. ostreatus; Be, B. edulis; Te, T. equestre; Ap, A. polytricha; Ls, L. scabrum; Sb, S. bovinus; Sl, S. luteus; Ld, L. deliciosus; Am, A. mellea; Ab, A. bisporus; Cc, C. cibarius; Bb, B. badius).
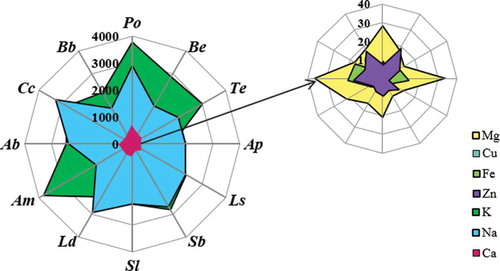
Figure 2. A radar chart of metals released into artificial gastric juice for an incubation time of 60 min. (Po, P. ostreatus; Be, B. edulis; Te, T. equestre; Ap, A. polytricha; Ls, L. scabrum; Sb, S. bovinus; Sl, S. luteus; Ld, L. deliciosus; Am, A. mellea; Ab, A. bisporus; Cc, C. cibarius; Bb, B. badius).
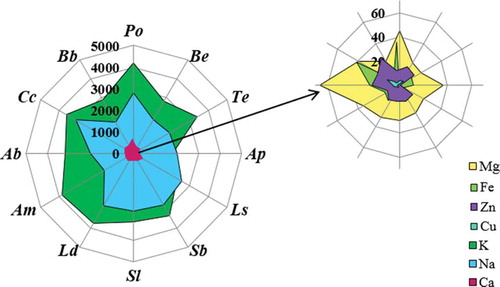
Figure 3. A radar chart of metals released into artificial gastric juice for an incubation time of 120 min. (Po, P. ostreatus; Be, B. edulis; Te, T. equestre; Ap, A. polytricha; Ls, L. scabrum; Sb, S. bovinus; Sl, S. luteus; Ld, L. deliciosus; Am, A. mellea; Ab, A. bisporus; Cc, C. cibarius; Bb, B. badius).
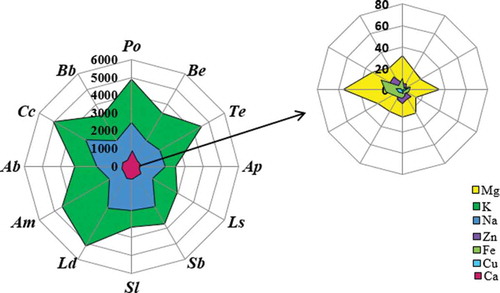
The highest amount of Mg in artificial saliva was released from P. ostreatus (55 mg/100 g dry weight; dw) and A. bisporus (47 mg/100 g dw; ). However, the lowest amount of Mg (18 mg/100 g dw) in artificial saliva was released from S. luteus. Therefore, based on the content of Mg released into the artificial gastric juice, it was found that the amount of Mg depends on the incubation time in gastric juice. It was usually observed that Mg was best released into artificial gastric juice when the fruiting bodies were incubated for 60 min – P. ostreatus released 45.1 mg/100 g dw of Mg (). The concentration of Mg in intestinal juice was 4.94 mg/100 g dw (L. scabrum) and 14.4 mg/100 g dw (A. bisporus). The results recorded for each species in artificial intestinal juice were characterized by a satisfactory level of reproducibility. Considering the results obtained for the release of K into artificial saliva, it was found that the best result was obtained, as in the previous case, for P. ostreatus (8590 mg/100 g dw; ) as opposed to L. scabrum, for which the lowest result was noted (4332 mg/100 g dw). Optimum results for the release of K into artificial gastric juice corresponded to an incubation time of 120 min – 4885 mg/100 g dw (P. ostreatus; ). The concentrations of K in the artificial intestinal juice were observed to be in a range from 814 mg/100 g dw for L. deliciosus to 3458 mg/100 g dw for B. edulis (). Artificial saliva also demonstrated Na content. The highest sodium concentration in the artificial saliva was obtained for A. mellea (5834 mg/100 g dw), while the lowest was for B. badius (2929 mg/100 g dw; ). In this case, the amount of Na released into the artificial gastric juice depended on the incubation time and mushroom species. Two groups were separated on this basis: the first group included L. deliciosus, P. ostreatus, T. equestre, A. bisporus, and C. cibarius, for which the most effective incubation time was 15 min (3285 mg/100 g dw of Na released from C. cibarius; ). The second group, for which the best incubation time was 60 min, included A. mellea, A. polytricha, B. badius, B. edulis, L. scabrum, S. bovinus, and S. luteus (2742 mg/100 g dw of Na released from S. bovines; ). The number of elements released into the artificial intestinal juice ranged from 2760 mg/100 g dw (A. polytricha) to 4181 mg/100 g dw (T. equestre). It was also found that the most efficient release of Na occurred in artificial intestinal juice (). Interesting results were obtained when analyzing the amount of Ca released into the artificial saliva. These contents were in a narrow range from 273 mg/100 g dw (L. deliciosus) to 368 mg/100 g dw (P. ostreatus; ), which suggests the absence of a relationship between mushroom species and the degree of Ca release into the artificial saliva. In such cases, as with Mg, the best results were found for an incubation time in gastric juice of 60 min (178 mg/100 g dw for P. ostreatus). The amount of Ca released into the artificial intestinal juice did not exceed 60 mg/100 g dw in any species. Moreover, the results obtained within each species were reproducible. The highest result—58.4 mg/100 g dw—was obtained for T. equestre, while the lowest was noted for B. edulis: 33 mg/100 g dw. Only trace amounts of microelements such as Cu and Zn were released into the artificial gastric juices; for example, the concentration of Cu in artificial saliva was 0.040 mg/100 g dw for A. polytricha and 0.43 mg/100 g dw for C. cibarius and the concentration of Zn was in a range from 0.249 mg/100 g dw for A. polytricha to 2.2 mg/100 g dw for C. cibarius. The optimum incubation time for Cu and Zn in artificial gastric juice was 60 min, similar to that of Mg and Ca (). The content of Cu released from C. cibarius into artificial gastric juice was found the highest—1.60 mg/100 g dw, in contrast to A. polytricha, for which the measured content was only 0.073 mg/100 g dw. For Zn, these values were 4.42 mg/100 g dw for B. badius and 0.537 mg/100 g dw for A. polytricha. Furthermore, at an optimum time of 60 min (), for both Cu and Zn, the least effective results for release into gastric juice were obtained for tree fungi (A. polytricha, A. mellea, P. ostreatus). Analysis of artificial intestinal juices showed that microelements such as Cu were ranged from 0.021 mg/100 g dw for A. polytricha to 0.537 mg/100 g dw for B. edulis and Zn was found in a range of 0.58 mg/100 g dw for P. ostreatus to 2.90 mg/100 g dw for A. mellea. Artificial saliva was also analyzed for Fe content within this study. The analysis demonstrated that this macroelement is present in an amount of 0.30 mg/100 g dw for S. luteus and 2.5 mg/100 g dw for P. ostreatus. It is interesting that this element was not released from L. scabrum. It was also observed that the macroelement Fe was best released at an incubation time of 60 min—6.7 mg/100 g dw for C. cibarius (). The release of Fe into artificial intestinal juice was low and in a range of 0.150 mg/100 g dw for S. luteus to 2.6 mg/100 g dw for A. bisporus.
Organic compounds
The presence and the content of indole compounds and phenolic acids were analyzed using HPLC. The analysis involved methanol extracts of lyophilized fruiting bodies of 12 species of edible mushrooms after digestion in solutions of artificial digestive juices (saliva, gastric, and intestinal juice). A total of 108 samples were determined, all in triplicates. The results are presented as mean values with SD ( and ). The standards of previously prepared indole compounds and phenolic acids were used for qualitative and quantitative analyses. For qualitative analyses, the retention times of compounds contained in extracts were compared with retention times of reference substances. Qualitative analyses involved previously prepared standard curves for the examined compounds, which were used to calculate the coefficients of the calibration curve equation
Table 2. Sized factor loads for the three main components (PC1, PC2, PC3).
Table 3. The content of indole compounds in the lyophilized biomass of 12 species of edible mushrooms extracted into artificial digestive juices over different time periods.
Table 4. The content of phenolic acids in the lyophilized biomass of twelve species of edible mushrooms extracted into artificial digestive juices over different time periods.
Seven indole compounds were detected and quantified: serotonin, 5-hydroxy-l-tryptophan, l-tryptophan, tryptamine, 5-methyltryptamine, 6-methyl-d,l-tryptophan, and melatonin. 5-hydroxy-l-tryptophan was determined in all samples and its levels in most analyzed extracts were the highest (ranging from 588 mg/100 g dw; ). Serotonin was also determined in a significant number of samples. The amounts of serotonin were of a similar order to those obtained for 5-hydroxy-l-tryptophan and even exceeded those in some samples. In the present experiment, the highest amount of serotonin (712 mg/100 g dw) was released from P. ostreatus into artificial digestive juices. The optimal times which allowed the highest content to be obtained after the extraction of mushroom fruiting bodies into artificial gastric juices for both the above compounds were 60 and 120 min of incubation in artificial gastric juice. Lower amounts of indole compounds were released after 15 min of incubation in artificial gastric juice, indicating that mushroom material is not sufficiently digested. Higher amounts of indole compounds were released after material digestion in artificial gastric juice compared to extracts obtained after material digestion in artificial intestinal juice. However, in one of the three time variants of digestion, B. edulis and A. bisporus proved to be exceptions (after 15 min of incubation in artificial gastric juice), and more 5-hydroxy-l-tryptophan and serotonin were released after sequential incubation in artificial intestinal juice compared to the artificial gastric juice. The lowest amounts of both indole compounds were released after 1 min of incubation of the material in artificial saliva solution, and were in a range from 7.16 mg/100 g dw for S. luteus to 22.1 mg/100 g dw for A. mellea. The highest amount of 5-hydroxy-l-tryptophan was released from A. bisporus and B. edulis (about 588 mg/100 g dw) and also C. cibarius, A. mellea, and L. scabrum (more than 300 mg/100 g dw). The lowest amounts were, in turn, determined for A. polytricha (from 10.5 mg/100 g dw in artificial saliva solution to 171 mg/100 g dw in artificial gastric juice after 120 min of digestion). The highest amount of serotonin was determined for P. ostreatus, B. edulis, S. luteus, L. deliciosus, and A. bisporus (a maximum of 712 mg/100 g dw). For all 12 species, the compound with the lowest release was l-tryptophan; in most samples less than 0.001 mg/100 g dw Tryptamine was determined in all species except A. polytricha; however, its levels were much lower than for 5-hydroxy-l-tryptophan and serotonin (from 11.06 mg/100 g dw to 14.2 mg/100 g dw). In turn, 6-methyl-d,l-tryptophan was not observed in C. cibarius, A. mellea, P. ostreatus, T. equestre, or A. polytricha. 5-Methyltryptamine is an indole compound which is also found in mushrooms. This compound was determined in all 12 species, and its levels ranged from 2.99 mg/100 g dw for B. edulis to 10.1 mg/100 g dw for A. bisporus. It is also interesting that melatonin is observed in edible mushrooms, but compared to serotonin or 5-hydroxy-l-tryptophan not in high doses, e.g., from 0.98 mg/100 g dw for P. ostreatus, A. polytricha and L. scabrum to 22.9 mg/100 g dw for A. mellea. The applied therapeutic dose of melatonin is most frequently from 1 mg to 5 mg, which with full bioavailability would indicate the need for the consumption of about 100 g of fruiting bodies, which release lower amounts of melatonin. For A. mellea, it has already been emphasized that a sufficient amount would be about 10 g of this species. Among the examined 12 species of edible mushrooms, the species that globally releases the highest amounts of indole compounds is one of the world’s most popular edible mushrooms, A. bisporus, in which the total number of seven released indole compounds is the highest when incubated for 120 min in artificial gastric juice. A slightly lower total content is observed in P. ostreatus and B. edulis, also after prior 120 min incubation in artificial gastric juice. Among the analyzed 12 mushrooms species, the lowest amount (both qualitatively and quantitatively) of indole compounds was determined in A. polytricha––this phenomenon can be explained by a tree fungus originating from a different climatic zone. In A. polytricha, 5-hydroxy-l-typtophan was determined in all replicates, serotonin and l-tryptophan in half of the analyzed material, and 5-methyltryptamine and melatonin only in one analyzed sample (after 15 min of material digestion in artificial gastric juice). In turn, 6-methyl-d,l-tryptophan and tryptamine were not determined in any of the nine extracts derived from the incubation of this mushroom species. The total amounts of indole compounds determined in A. polytricha ranged from 152.77 to 379.7 mg/100 g dw
Protocatechuic acid, p-hydroxybenzoic acid, syringic acid, and gallic acid were determined in the edible mushrooms analyzed. Protocatechuic acid was determined in as many as seven species. P-hydroxybenzoic acid was also determined in seven species, but in fewer samples. The extracts of P. ostreatus, L. scabrum, A. mellea, and B. badius obtained after incubation did not contain any of these compounds. This may indicate that these compounds are present next to each other in a given species, and are released to the digestive juices in the same conditions, and that they are present in most analyzed edible mushrooms species. A characteristic feature of these species is the fact that although p-hydroxybenzoic and protocatechuic acids were absent, a considerable amount of syringic acid (maximum up to 19 mg/100 g dw) was observed (). Gallic acid was determined in a limited number of extracts (B. edulis, A. polytricha, L. scabrum, and C. cibarius). The amounts of protocatechuic acid ranged from 0.6 to 8.6 mg/100 g dw, p-hydroxybenzoic acid from 0.03 to 1.3 mg/100 g dw, syringic acid from 0.28 to 19 mg/100 g dw, and gallic acid from 0.02 to 15 mg/100 g dw Syringic and gallic acids were determined in a limited number of samples and in higher quantities. Four phenolic acids were determined in C. cibarius. B. badius, and P. ostreatus were proved to be species in which only syringic acid was determined. In contrast, none of these phenolic acids were determined in the fruiting bodies of A. mellea. Boletus badius released the highest total amount of phenolic acids––23.5 mg/100 g dw––at an incubation time of 120 min in artificial gastric juice. Leccinum scabrum released the lowest total amount of phenolic acids into artificial gastric juices at an incubation time of 60 min prior species digestion in artificial gastric juice (0.03 mg/100 g dw). The least preferred solution for phenolic acid release was artificial saliva, probably due to the short incubation time. It is difficult to determine unequivocally which digestion time is optimal for phenolic acid release to digestive juices, since this varied depending on the mushroom species incubated.
Discussion
The results of the study (see ) constitute a large and important source of information concerning the release of bioelements and organic compounds into artificial digestive juices. Due to the number of results, as well as the dispersion of their values, interpretation is difficult. Therefore, in order to obtain relevant information to identify the relationships between the objects (mushroom species) and measured variables (bioelements, organic compounds), chemometric tools were used (Statgraphics Centurion XVI)––cluster analysis (CA) and principal component analysis (PCA).
CA
The first method used in the study, CA, allows identification of groups—clusters characterized by a clear similarity, or a lack thereof (). The basis of the analysis is the distance between the objects. Objects are classified as similar if their position in multi-dimensional space is not distinct. The results are presented in graphical form—dendrograms ( and ). For this purpose, Ward’s algorithm was used as a distance measure, and the distance between the clusters was calculated based on the City-Block method. The dendrograms, known as trees ( and ), present the clusters, in which the x-axis corresponds to be the analyzed element (), while the y-axis is identified as the distance between the examined objects.[Citation12,Citation27–Citation30] In , the x-axis shows the division due to the time of incubation in artificial gastric juice (I: 15 min; II: 60 min; III: 120 min) and mushroom classification according to hymenophore (b: lamellar; r: tubular; n: no classification).
Figure 4. Dendrogram presenting similarity observed between the analyzed micro- and macroelements (The City-Block distance, Ward’s algorithm).
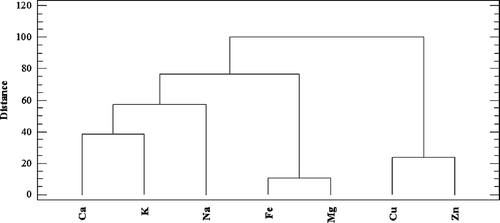
Figure 5. Dendrogram presenting similarity within 12 mushroom species resulting from incubation time in artificial gastric juice and their classification (incubation time: I: 15 min; II: 60 min; III: 120 min. Classification: b: lamellar; r: tubular; n: no classification; m: fungi forming mycorrhiza; p: parasitic fungi).
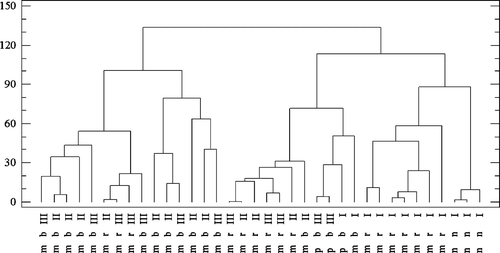
Three clusters (subgroups) of elements characterized by a similar variability were distinguished based on the analysis of similarity (). The first cluster is formed by Cu, K, and Na. The second group includes Fe and Mg and the third group is represented by Cu and Zn. Elements belonging to the group may demonstrate similar tendencies within the cluster for bioelement release into artificial gastric juice. Moreover, the highest correlation was observed within the second (green line) and third group (pink line), as evidenced by the shortest length of dendrogram arms. On considering the second dendrogram (), an arrangement in several areas (different incubation times in artificial gastric juice) was observed. It can be noted in this case that the releasing tendencies of macro- and microelements in a time period of 60 and 120 min are similar. In turn, the elements that are released in a time period of 15 min belong to a completely separate group that does not have any relationships with the first one. Moreover, mushrooms are further classified into subgroups based on the structure of their hymenophore (e.g. lamellar and tubular). There is a high level of similarity within the specified group which suggests that a correlation may exist between the release of bioelements into artificial gastric juice and mushroom structure.
The second method applied in the study, PCA, enables a reduction in the number of input variables needed to examine the interactions occurring in a relevant set of data.[Citation27–Citation32] Based on the main components analysis, it was observed that 70.8% of the changes in the output data set may be described using the first three so-called principal components (PC1, PC2, and PC3). Thus, the obtained variables correspond to the linear combination of primary variables multiplied by the corresponding load value. The size of the load corresponds to variable saturation and represents the correlation coefficient for the basic variables. It should be emphasized that the most significant effect is noted for the element, for which the assigned load value is the highest. Load values are presented in . Based on this, it can be concluded that the size of PC1 is significantly affected by the concentration of Fe and Ca. Other variables can be combined in the same way. The use of this procedure allows the presentation of the results in three-dimensional space, based on the principal components PC1, PC2, and PC3. One such graphical presentation in three-dimensional space is a biplot ().
Figure 6. Biplot-graph created in three-dimensional space, presenting the correlation between the incubation time of mushroom material in artificial gastric juice and amounts of elements released (created on the basis of three main components PC1, PC2, PC3. I: incubation time 15 min; II: incubation time 60 min; III: incubation time 120 min).
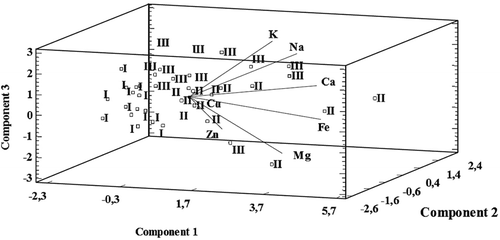
It was observed that the increase in the content of analyzed macro- and microelements occurs along the straight arms marked on the graph, and is directed to where the release time from the mushrooms to artificial gastric juice was 60 and 120 min (II and I, respectively). However, in the area with elements marked I—corresponding to 15 min—there was no such tendency. These results confirm the thesis that the amounts of elements released into artificial gastric juice are related to the incubation time of the mushroom materials. In addition, an incubation time of 15 min is sufficient to digest the mushroom material ().
Organic compounds
Indole compounds had previously been determined in extracts to simulated gastric juice at 25°C.[Citation33] The composition of gastric juice was the same, and the samples were incubated for four time variants – 15, 30, 60, and 90 min. No reference was made in the previous study to the 120 min of material digestion in a solution of artificial gastric juice, which was carried out in the present experiment. Muszyńska et al. identified the following: 5-hydroxy-l-tryptophan (0.6–100 mg/100 g dw), serotonin (0.01 mg/100 g dw), l-tryptophan (0.9–8.3 mg/100 g dw), and 5-methyltryptamine (33 mg/100 g dw).[Citation33] The species in which L-tryptophan was noted in the present study are consistent with the species previously examined for the content of this compound. The amounts of other indole compounds were slightly different from those obtained in the present experiment. This indicates that conditions possibly similar to those prevailing in the human body (a temperature of 37°C and imitation of peristaltic movements in Gastroel-2014 by sample rotation) affect the release of indole compounds from the fruiting bodies of edible mushrooms in most of the materials analyzed. Partial digestion of the fruiting bodies in artificial saliva solution may also be a factor that enhances the subsequent release of indole compounds into the solution of artificial gastric juices. Considerably higher amounts of indole compounds were released from particular mushroom species, because artificial intestinal juice is considered to be the optimum environment for the release of these compounds, a factor which was not considered in the previous experiment. Also, seven indole compounds, in contrast to four as in the previous experiment, were determined in this experiment.[Citation33] Phenolic compounds were determined in the conditions described in the present study for the first time. Similar results have been achieved by other studies, but in a methanolic extract of freeze-dried mushrooms.[Citation34]
Conclusion
This study demonstrated in an innovative way that fruiting bodies of edible mushrooms are a valuable source of indole compounds, phenolic acids, and also elements that are necessary for the proper functioning of the organism. There are still no comparative studies into the release of physiologically active compounds from edible mushroom fruiting bodies, and this emphasizes the innovative nature of the present study. The study also demonstrated that mushrooms, contrary to popular opinion, are valuable components of the diet. Simulation of the conditions existing in the human digestive tract (Gastroel-2014, Jagiellonian University Medical College) indicates an environment suitable to study the release of these health-promoting components. It was proved that mushrooms not only accumulate and synthesize particular compounds and bioelements, but also release them into the human gastrointestinal tract. Future studies should focus on the extent of the absorption of these compounds released from the fruiting bodies of mushrooms and into the tissues and organs of the human body.
References
- Falandysz, J. Selenium in Edible Mushrooms. Journal of Environmental Science and Health 2008, 26, 259–299.
- Muszyńska, B.; Sułkowska-Ziaja, K.; Ekiert, H. Indole Compounds in Edible Basidiomycota Species: Boletus Edulis (Bull. ex Fr.), Suillus Luteus (L. ex Fr.) and Pleurotus Ostreatus (Jacq.ex Fr.) Kumm. International Journal of Medicinal Mushrooms 2011, 13, 449–454.
- Muszyńska, B.; Sułkowska-Ziaja, K.; Ekiert, H. Phenolic Acids in Selected Edible Basidiomycota Species: Armillaria Mellea, Boletus Ba1dius, Boletus Edulis, Cantharellus Cibarius, Lactarius Deliciosus and Pleurotus Ostreatus. Acta Scientiarum Polonorum Hortorum Cultus 2013, 12, 107–116.
- Muszyńska, B.; Sułkowska-Ziaja, K.; Ekiert, H. Analysis of Indole Compounds in Methanolic Extracts from the Fruiting Bodies of Cantharellus Cibarius (the Chanterelle) and from the Mycelium of This Species Cultured in Vitro. Journal of Food Science and Technology 2013, 50, 1233–1237.
- Elmastas, M.; Isildak, O.; Turkekul, I.; Temur, N. Determination of Antioxidant Activity and Antioxidant Compounds in Wild Edible Mushrooms. Journal of Food Composition and Analysis 2007, 20, 337–345.
- Ng, T.B.; Wang, H.X. A Homodimeric Laccase with Unique Characteristics from the Yellow Mushroom Cantharellus Cibarius. Biochemical and Biophysical Research Communications 2004, 313, 37–41.
- Rangel-Castro, J.I.; Staffas, A.; Danell, E. The Ergocalciferol Content of Dried Pigmented and Albino Cantharellus Cibarius Fruit Bodies. Mycological Research 2002, 106, 70–73.
- Bedry, R.; Baudrimont, I.; Deffieux, G.; Creppy, E.E.; Pomies, J.P.; Ragnaud, J.M.; Dupon, M.; Neau, D.; Gabinski, C.; De Witte, S.; Chapalain, J.C.; Godeau, P.; Beylot, J. Wild-Mushroom Intoxication As a Cause of Rhabdomyolysis. The New England Journal of Medicine 2001, 345, 798–802.
- Gunde-Cimerman, N.; Cimerman, A. Pleurotus Fruiting Bodies Contain the Inhibitor of 3-Hydroxy-3-Methylglutaryl-Coenzyme a Reductase-Lovastatin. Experinental Mycology 1995, 9, 1–6.
- Hossain, S.; Hashimoto, M.; Choudhury, E.K. Dietary Mushroom (Pleurotus Ostreatus) Ameliorates Atherogenic Lipid in Hypercholesterolaemic Rats. Clinical and Experimental Pharmacology and Physiology 2003, 30, 470–475.
- Nosálová, V.; Bobek, P.; Černá, S.; Galbavý, S.; Štvrtina, S. Effects of Pleuran (Beta-Glucan Isolated from Pleurotus Ostreatus) on Experimental Colitis in Rats. Physiologycal Research 2001, 50, 575–581.
- Smiderle, F.R.; Olsen, L.M.; Carbonero, E.R.; Baggio, C.H.; Freitas, C.S.; Marcon, R.; Santos, A.R.; Gorin, P.A.; Iacomini, M. Anti-Inflammatory and Analgesic Properties in a Rodent Model of a (1–>3),(1–>6)-Linked Beta-Glucan Isolated from Pleurotus Pulmonarius. European Journal of Pharmacology 2008, 597, 86–91.
- Koyalamudi, S.R.; Jeong, S.C.; Song, C.H.; Cho, K.Y.; Pang, G. Vitamin D2 Formation and Bioavailability from Agaricus Bisporus Button Mushrooms Treated with Ultraviolet Irradiation. Journal of Agricultural and Food Chemistry 2009, 57, 3351–3355.
- Ding, X.; Hou, Y.; Hou, W.; Zhu, Y.; Fu, L.; Zhu, H. Structure Elucidation and Anti-Tumor Activities of Water-Soluble Oligosaccharides from Lactarius Deliciosus (L. ex Fr.) Gray. Pharmacognosy Magazine 2015, 11, 716–723.
- Hou, Y.; Ding, X.; Hou, W.; Song, B.; Wang, T.; Wang, F.; Zhong, J. Immunostimulant Activity of a Novel Polysaccharide Isolated from Lactarius Deliciosus (L. ex Fr.) Gray. Indian Journal of Pharmaceutical Sciences 2013, 75, 393–399.
- Muszyńska, B.; Łojewski, M.; Rojowski, J.; Opoka, W.; Sułkowska-Ziaja, K. Natural Products of Relevance in the Prevention and Supportive Treatment of Depression. Psychiatria Polska 2015, 49, 435–453.
- Guo, Y.J.; Deng, G.F.; Xu, X.R.; Wu, S.; Li, S.; Xia, E.Q.; Li, F.; Chen, F.; Ling, W.H.; Li, H.B. Antioxidant Capacities, Phenolic Compounds and Polysaccharide Contents of 49 Edible Macro-Fungi. Food & Function 2012, 3, 1195–1205.
- Alves, M.J.; Ferreira, I.C.F.R.; Froufe, H.J.C.; Abreu, R.M.V.; Martins, A.; Pintado, M. Antimicrobial Activity of Phenolic Compounds Identified in Wild Mushrooms, SAR Analysis and Docking Studies. Journal of Applied Microbiology 2013, 115, 346–357.
- Sheu, F.; Chien, P.J.; Chien, A.L.; Chen, Y.F.; Chin, K.L. Isolation and Characterization of An Immunomodulatory Protein (APP) from the Jew’s Ear Mushroom Auricularia Polytricha. Food Chemistry 2004, 87, 593–600.
- Kalać, P. Chemical Composition and Nutritional Value of European Species of Wild Growing Mushrooms: A Review. Food Chemistry 2009, 113, 9–16.
- Knudsen, H.; Vesterholt, J. Funga Nordica: Agaricoid, Boletoid and Cyphelloid Genera. Nordsvamp Press: Copenhagen, Denmark, 2008.
- Arvidson, K.; Johasson, E.G. Galvanic Current Between Dental Alloys in Vitro. Scandinavian Journal of Dental Research 1985, 93, 467–473.
- Polish Pharmacopeia. PTFarm Press: Warsaw, Poland, 2014.
- Neumann, M.; Goderska, K.; Grajek, K.; Grajek, W. The in Vitro Models of Gastrointestinal Tract to Study Bioavailability of Nutriments. Food Science Technology Quality 2006, 1, 30–45. (in Polish)
- Muszyńska, B.; Sułkowska-Ziaja, K.; Ekiert, H. Indole Compounds in Fruiting Bodies of Some Edible Basidiomycota Species. Food Chemistry 2011, 125, 1306–1308.
- Ellnain-Wojtaszek, M.; Zgórka, G. High-Performance Liquid Chromatography and Thin-Layer Chromatography of Phenolic Acids from Ginkgo Biloba L. Leaves Collected Within Vegetative Period. Journal of Liquid Chromatography & Related Technologies 1999, 22, 1459–1471.
- Gemperline, P. Practical Guide to Chemometrics. Taylor & Francis Group Press: Boca Raton, FL, 2006.
- Massart, M.B.G.; Vandeginste, L.M.C.; Buydens, S.; De Jong, P.J.; Lewi, J.; Smeyers-Verbeke, J. Handbook of Chemometrics and Qualimetrics: Part A. Elsevier Press: Amsterdam, The Netherlands, 1997.
- Mazerski, J. Podstawy Chemometrii [Fundamentals of Chemometrics]. Wyd. Politechniki Gdańskiej Press: Gdańsk, Polska, 2000. (in Polish)
- Otto, M. Chemometrics: Statistics Computer Application in Analytical Chemistry. Wiley-VCH Press: Weinheim, Germany, 1999.
- Brereton, R.G. Chemometrics: Data Analysis for the Laboratory and Chemical Plant. John Wiley & Sons Press: Chichester, England, 2003.
- Kramer, R. Chemometric Techniques for Quantitative Analysis. Marcel Dekker Press: New York, NY, 1998.
- Muszyńska, B.; Kała, K.; Sułkowska-Ziaja, K.; Gaweł, K.; Zając, M.; Opoka, W. Determination of Indole Compounds Released from Selected Edible Mushrooms and Their Biomass to Artificial Stomach Juice. LWT–Food Science and Technology 2015, 62, 27–31.
- Muszyńska, B.; Sułkowska-Ziaja, K.; Ekiert H. Analysis of the Contents of Phenolic Compounds in Edible Basidiomycota Species: Armillaria Mellea, Boletus Edulis, Boletus Badius, Cantharellus Cibarius, Lactarius Deliciosus and Pleurotus Ostreatus. Acta Scientiarum Polonorum Hortorum Cultus 2013, 12, 107–116.