ABSTRACT
Ekşikara (Vitis vinifera L.) is a poorly known native Turkish grape cultivar, which is being cultivated for thousands of years for table consumption, raisin, and grape molasses production. The aim of this study was to investigate the phenolic profile of whole berry, skin, and seeds of the cultivar and to determine the effect of harvesting year and altitude of the vineyard location. The grape samples were collected from two vineyards, located at 1000 and 1500 m of altitude in year 2014 while it was collected from 1500 m of altitude in year 2015. In addition to phenolic profile, antioxidant activity, total phenolic, anthocyanin, and tannin contents, and fatty acid profile of seed oil were also analyzed. In this study, anthocyanins, resveratrol, rutin, and isorhamnetin-3-glucoside were mainly found in skin while monomers and dimers of flavan-3-ols were present mainly in the seed. Altitude had a drastic effect on phenolic compounds as well as on the antioxidant activity of whole berry, skin, and seeds.
Introduction
European grape vine (Vitis vinifera) is a well-known fruit crop native to Mediterranean region and central Asia. Ekşikara is a cultivar and a local variety of Vitis vinifera and originating from south of Central Anatolia, especially Konya-Karaman region. It is grown around the towns of Hadim and Taşkent under the name of Keçimen and Karaoğlan. Its berries are sweet, distinctive intensely aromatic, and dark-skinned. It is generally cultivated for table consumption, raisin, and grape molasses production. Despite of having special taste and aroma, Ekşikara still remains a poorly known and uncommon cultivar.
The chemical composition of seeds, skin, and fleshy tissues of grape berry differs considerably from each other and is affected by several factors such as variety, climate, water availability, soil, sun light, and altitude.[Citation1] Phenolic compounds in edible plants are the main secondary metabolites, which are abundantly presented, and originated from tyrosine and phenylalanine.[Citation2] Importance of polyphenols in plants arise from positive effects to sensory properties of products, and human health.[Citation3] Health beneficial effects of phenolics are usually associated with antioxidant activities, oxygen spices scavenging abilities, free metal chelation abilities, and nitrosation inhibition abilities.[Citation4]
Grape berries contain phenolic acids (hydroxybenzoic andhydroxycinnamic acid, and their derivatives), stilbenes, flavon-3-ols, flavonols, anthocyanins, and condensed tannins.[Citation5] Amount and profile of phenolics in grape show considerable variations in different parts of fruits like seed, skin, and pulp.[Citation6] Accumulation of flavonoid in plants is induced by biotic and abiotic stresses.[Citation7] Exogenous factors such as light, temperature, and pathogenesis affect synthesis of flavonols. Especially, flavonol synthesis is a light dependent process, and UV-B radiation induces it.[Citation8,Citation9] The anthocyanin profile of grape is intrinsic to the varieties and it is thought to be controlled by some genetic factors.[Citation10] Whereas, differences in the amount of individual anthocyanin are the result of the enviromental factors. Some environmental factors, such as sun light exposure, plant growth regulators, and water deficits promote the anthocyanin synthesis.[Citation11] Latitude and altitude of vineyard also affect the grapes polyphenol synthesis by making differences in sunlight hours, temperature, and UV-B exposure to vines.[Citation12] Many of Vitis species can synthesize stilbene, which is influenced by factors such as soil type, heat stress, altitude of cultivation site, fertilizer application. In many cases, stilbene accumulation is induced by water deficits, fungal infection, and ultraviolet (UV) radiation.[Citation9] The aim of this study was to determine phenolic profiles and antioxidant activity of Ekşikara grapes berry, skin, and seeds harvested from vineyards located at different altitudes, and in different years. We intend to introduce this cultivar to researchers.
Material and methods
Samples
Ekşikara grape variety is native to South of the Central Anatolia Region. Therefore, two vineyards, located at Hadim, Konya, Turkey (latitude 36° 59’ N; longitude 32° 27’ E) were chosen as sampling area (). The first vineyard was located at an altitude of 1500 m and the other was at 1000 m. The grapes were harvested from these two vineyards in September 2014. The samples were also collected in the following year (September 2015) from the vineyard located at 1500 m to investigate the changes in phenolic composition depending on harvesting year.
Figure 1. A map showing sampling area: A: Map of sampling site in Turkey; B: Location of vineyards in Hadim, Konya. In Figure B, the vineyard labeled with circle is located at 1000 m and rectangle at 1500 m.
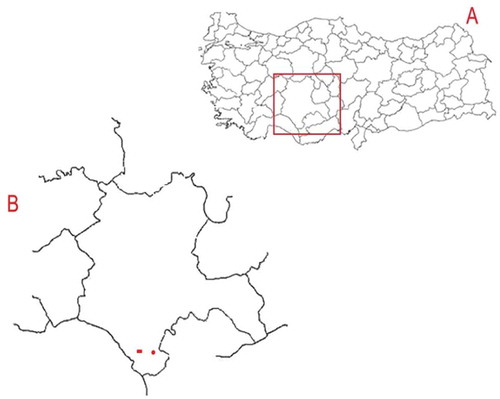
The vines were grown on own roots. Sixty clusters from different vines were used in sampling. Ten berries were removed from each cluster and 600 berries were obtained. They were divided equally into three groups to obtain whole berry, seeds, and skin. Grapes, harvested at commercial maturity (22 °Brix), were transferrred to the laboratory, and were manually separated into seeds and skin. Seeds, skins, and whole grapes were lyophilizied in a freeze dryer (Scanvac, Coolsafe, Lynge, Denmark) seperately and stored at –20°C.
Chemicals
Gallic acid, procyanidin B1, (+)-catechin, procyanidin B2, (-)-epicatechin, epigallocatechin, epicatechin gallate, gentisic acid, chlorogenic acid, caffeic acid, rutin, kaempferol-3-glucoside, isorhamnetin-3-glucoside, and trans-resveratrol were purchased from Extrasynthese (Genay, France). High-performance liquid chromatography (HPLC) grade acetonitrile, ethyl acetate, methanol, acetic acid, Folin Ciocalteu’s reagent, sodium carbonate, sodium hydroxide, sodium acetate trihydrate, hydrochloric acid, potassium peroxodisulfate were acquired from Merck (Darmstadt, Germany). 2,2-Diphenyl-1-picrylhydrazyl (DPPH), 2,2’-Azino-bis(3-ethylbenzothiazoline-6-sulphonic acid; ABTS), bovine serum albumin, and ethanol were obtained from Sigma Chemical Co. (St. Louis, MO). 6-Hydroxy-2,5,7,8-tetramethylchroman-2-carboxylic acid (Trolox) was from Fluka.
Analyses
Extraction procedure for total phenolic, tannin, and total monomeric anthocyanin analyses
Lyophilized whole berries, skins, and seeds were finely ground. One gram of each fraction was extracted with 50 mL acidified methanol:water (80:20) by using a homogenizer (WiseMixTM HG-150; Daihan Scientific, Korea). Then, extracts were centrifuged (NF 800R, Nuve, Turkey) at 4100 × g for 10 min. Supernatant was removed and collected into a glass jar. The residual pellate was re-extracted with methanol:water mixture. The supernatants from two extractions were pooled and stored at –18°C until further analyses.
Total phenolic content
The total phenolic contents of samples were determined using Folin–Ciocalteu procedure, described by Akbulut and Coklar.[Citation13] The methanolic extract (0.5 mL) was mixed with 2.5 mL Folin–Ciocaleu’s reagent (0.2 N) and 2.0 mL sodium carbonate (75 g/L). The samples were read against the blank at 765 nm after 120 min using a spectrophotometer (U-1800, Hitachi, Japan). Gallic acid standard solutions at concenrations 12.5 to 200 ppm were used to construct the calibration curve and the results were expressed as milligram gallic acid equivalents (GAE)/g dry weight (DW).
Total monomeric anthocyanin content
Total monomeric anthocyanin content in the samples was determined by pH differential method as described by Akbulut andCoklar.[Citation13] Briefly, 1 mL of extract was taken into two flasks and diluted with 4 mL of a buffer pH 1.0 (potassium chloride, 0.025M) and buffer at pH 4.5 (sodium acetate, 0.4M), separately. After 30 min, absorbance at wavelenghts 515 and 700 nm was recorded and the difference between the values was calculated according to Eq. (1). The total monomeric anthocyanin content of samples was calculated by Eq. (2) and results were reported as milligram of malvidin-3-glucoside equivalent (ME)/g DW.
where A is the absorbance differences, DF is dilution factor, MW is molecular weight of malvidin-3-glucoside (493.43g/mol), ε is molar absorptivity of malvidin-3-glucoside (28000 L /mol cm), and l is pathlength in centimeters.
Tannin content
Tannin content of the extracts was determined by protein precipitation method as decribed by Hagerman and Butler.[Citation14] Briefly, 1 mL methanolic extract was added to 2 mL of bovine serum albumine solution at a concentration of 1.0 mg/mL (in 0.20 M acetate buffer, pH 5.0, containing 0.17 M sodium chloride), kept at 4°C overnight to precipitate protein and tannin complex, and then centrifugated at 9000 × g for 15 min. Pellet was dissolved in 4 mL of SDS-triethanolamine, 1 mL 0.01 M ferric chloride in 0.01 N hydrochloric acid was added and immediately vortexed. The absorbance was recorded at 510 nm after 15 min. The results were calculated according to the standard curve that was prepared using 0.1–1 mg/mL tannic acid solutions and given as mg/g of DW.
Antioxidant activity
Ferric reducing antioxidant power (FRAP) assay
The FRAP of the samples were determined as per the method described by Benzie and Strain.[Citation15] Briefly, 50 µL extract and 150 µL deionized water were added to the 1.5 mL fresly prepeared FRAP reagent (300 M acetate buffer, pH 3.6:10 M TPTZ:20 M FeCl3.6H2O [10:1:1]). The reaction mixture was incubated at 37.0°C for 4 min. Absorbance was read at 593 nm, and the results were calculated according to the calibration curve of FeSO4. 7H2O prepared in the range of 100–1000 µmol/L. Results were given as µmol Fe+2/g DW.
ABTS assay
The protocol, described by Re et al.,[Citation16] was followed to determine the ABTS antioxidant activity of extracts. To generate the ABTS• radical, 2.5 mL of potassium persulfate solution (2.45 Mm) was added to 5 mL ABTS solution (7 mM). The mixture was incubated at room temperature nearly 16 h. The stock solution was diluted with ethanol, to an absorbance of 0.700 ± 0.02 at 734 nm. The extract (10 µL) was added to 990 µl ABTS• solution. The absorbance at 734 nm was measured after 6 min and the reduction in the absorbance was noted. Results were reported as mmol Trolox equivalent (TE)/kg DW.
DPPH assay
DPPH• antioxidant activity was determined according to the Brand-Williams et al.[Citation17] Briefly, an aliquot (0.1 mL) of extract was added to 3.9 mL of a DPPH (6×10−5 M) methanolic solution. After 30 min of incubation at room temperature in the dark, the absorbance was measured at 515 nm. The results were expressed as mmol TE/kg DW.
Anthocyanin and phenolic profile
One gram of lyophilized and milled seed, skin and whole berry was seperately weighted and extracted with 50 mL methanol:water:formic acid (50:48.5:1.5) mixture by homogenizer for 2 min and centrifuged at 3000 × g for 10 min at 4°C. The supernatant was collected and the residue was re-extracted with 20 mL solvent mixture.
For phenolic and anthocyanin profile analysis, 2 mL of extract was purified by using C18 SPE cartidges (Agilent, USA). Non-anthocyanin phenolics and anthocyanins were eluted with ethyl acetate and methanol, respectively. Ethyl acetate and methanol eluates were evaporated at 35°C, resuspended in 1 mL methanol and then filtred through 0.45 µm pore size syringe filter (Sartorius AG, Goettingen, Germany). To determine trans-resveratrol content of grape, liquid-liquid extraction procedure was carried out by using ethyl acetate.
The analysis of phenolic compounds in the extracts was carried out by Agilent 1260 Infinity Series HPLC system equipped with diode array detector. Seperation was achieved by a reverse phase C18 column (5 μm, 250 × 4.6 mm i.d). Mobil phase consisted of acetic acid:water and water:acetonitril:acetic acid, and the flow rate was 0.75 mL/min. The detector was set at 280, 306, 320, and 360 nm for non-anthocyanin phenolics and 520 nm for anthocyanins. The identification of phenolics was confirmed by comparing their retention times and UV spectra. The data were analyzed by ChemStation software.[Citation18]
Fatty acid profile of grape seeds oil
The ground seeds (4 g) were extracted with petrolium ether in an soxhlet extractor for 6 h as described by Gecgel et al.[Citation19] To prepare the methyle esters of fatty acids, 0.1 g oil was weighed in an screw cap tube, 0.2 mL potassium hydroxide in methanol (2N) and 2 drops of methyl orange were added. The flask was shaked for 30 s, after phase separation, the upper layer was transferred into a vial for gas chromatography (GC) analysis.[Citation20] Fatty acid profile anlysis was performed on an agilent 7890 A-FID gas chromatography system, equipped with 100 m × 0.25 mm × 0.2 µm HP-88 capillary column. Split injector and flame ionization detector (FID) were operated at 260°C. Oven temperature was set at 140°C, held for 5 min, and ramped at 4°C/min to 240 for 15 min. The sample (1 µL) was injected to system with a split ratio 30:1. Hydrogen gas was used as carier gas at a flow rate of 30 mL/min, and air flow rate was set at 300 mL/min.[Citation21]
Statistical analysis
The results are being presented as means ± standard deviations (SD) and were subjected to one-way analysis of variance (ANOVA), at a confidence level of 95% to determine whether there was any significant difference between the means of altitude and harvested year. Statistical analysis was performed by using MINITAB (Released 14, Minitab Inc. USA).
Results and discussion
Total phenolics, total monomeric anthocyanin, and tannin content of the fractions of Ekşikara grape
shows the data of the total phenolic content, total monomeric anthocyanin content, and tannin content of seeds, skin, and whole grape berry as a function of harvested year and location. Total phenolic content of whole berry, seed, and skin of Ekşikara grape harvested in 2014 were found to be 20.91, 115.74, and 49.58 mg GAE/g DW, respectively. In the samples of 2015, total phenolic content was nearly same as quantitative in seeds and whole grapes.
Table 1. Total phenolic, total monomeric anthocyanin, and tannin contents of whole berry, skin, and seed in Ekşikara grape.
However, the total phenolic content in skins showed a decrease according to 2014 year.
Several studies have determined total phenolic content of berries, seeds, and skins of distinct grape cultivars.[Citation6] Most of them reported that total phenolic content in Vitis vinifera seeds is higher than that in skin.[Citation6,Citation22] Xu et al.[Citation23] reported the phenolic content of 18 grape cultivars, and found a variation from 15.79 to 99.28 mg GAE/g DM for seed and from 12.11 to 41.21 mg GAE/g DM for skin.
An increase in total phenolic content of all fractions was noted with increasing altitude. However, there were some insignificant changes in total phenolic of different fractions according to the year, except the skin. The total phenolic content of skin was decreased from 49.58 to 39.66 mg GAE/g DW in following year.
Phenolic compound accumulation is highly dependent on the enviromental factors.[Citation8,Citation24–Citation28] Vineyard soil quality, temperature, humidity, solar, and UV radiation are influenced by altitude.[Citation12] Ristic et al.[Citation29] noted a significant impact of shading on total phenolic content of grape berries. According to their results, the grapes grown in shaded had a lower total phenolic content than the grape with a normal sunlight exposure.
Total monomeric anthocyanin content of Ekşikara berries and skins was determined as 3.24 and 27.24 mg/g DW, respectively. Significant differences in total monomeric anthocyanin content were also detected among the berries and skins of Ekşikara located at different altitudes. This finding is consistent with previous studies that investigated the effect of altitude on anthocyanin accumulation.[Citation24]
An increase in total anthocyanin content of grape berries under the influence of sunlight exposure and cold temperature was noted by Cohen et al.[Citation30] Lower anthocyanin content in berry skin at higher temperature, such as 35°C, is thought to depend upon the anthocyanin degradation and inhibition of anthocyanin accumulation.[Citation31] Regarding to tannin content of fractions, the highest amount of tannin was found in seed with 46.72 mg/g DW in 2014 at high altitude. The lowest value was in whole berry with 7.52 mg/g harvested in 2015. The tannin content in seed was decreased from 46.72 to 39.76 mg/g DW in the following year. With increasing altitude, a statistically significant decrease was observed in both seed and skin tannin levels.
Antioxidant activities of grape berries, seeds, and skins
To evaluate the antioxidant activity of berry, skin, and seed extracts of Ekşikara, DPPH, ABTS, and FRAP methods were used. The results of DPPH antioxidant activity are shown in . Among all three fraction, seed exract of grapes harvested from high altitude in 2015 showed the maximum antioxidant activity with 362.34 mmol TE/kg DW, whereas whole berry cultivated at low altitude showed the lowest antioxidant activity (23.34 mmol TE/kg DW). There was no statistically significant difference in DPPH antioxidant activity of seed extract from the standpoint of harvesting year and location altitude. Similar result was found in FRAP antioxidant activity of the seed extract (). The ABTS antioxidant activity of the seed extract showed significant decrease for the sample collected at the low altitude (). The DPPH value of whole berry extract showed an increase from 49.97 to 53.86 mmol TE/kg DW in the following year. Statistically, significant (p < 0.01) decrease in DPPH value for whole grape extract was seen with the decrease in altitude. In the contrast to the whole berry and seed extracts, the DPPH, and FRAP antioxdant activities of skin extract was different for both the years. With the decreasing altitude, all methods reported significant decreases in the antioxidant activities of Vitis vinifera berry, seed, and skin.
Figure 2. DPPH antioxidant activities of whole grape, seeds, and skin of Ekşikara cultivated at different altitude and year.

Figure 3. ABTS antioxidant activities of whole grape, seeds and skin of Ekşikara cultivated at different altitude and year.
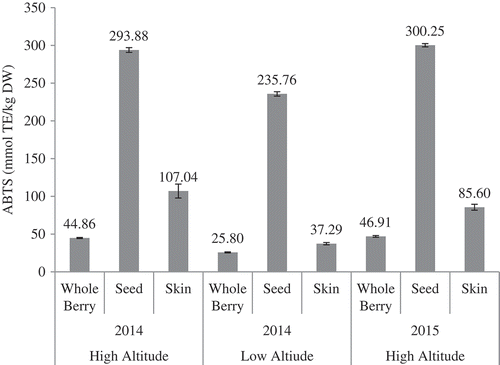
Figure 4. FRAP values of whole berry, seeds, and skin of Ekşikara grape cultivated at different altitude and year.
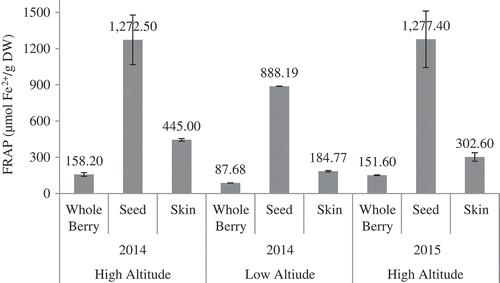
Xu et al.[Citation23] evaluated the antioxidant activity of seeds and skin extracts for 18 grape cultivars and found DPPH antioxidant activity of 94.05–275.96 µmol TE/g DM for skin and 52.42–422.18 2 µmol TE/g DM for seeds. Ky andTeissedre[Citation32] reported the FRAP values for seed extract of Grenache, Syrah, Carignan cultivars as 1.28, 1.45, and 1.20 mmol Fe2+/g DW, respectively.
Phenolic compunds, such as vitamin C and E, tocopherols, tocotrienols and carotenoid, are one of the key antioxidants. Hydroxycinnamates exhibit equal or two times greater antioxidant activity than vitamin C or E, while flavan-3-ols, anthocyanidins, and flavon-3-ols possess aproximately two- to five-fold higher antioxidant activity than that of vitamin C and E.[Citation33] As noted by Spranger et al.,[Citation34] antioxidant activity of flavan-3-ols is positively related to their degree of polymerization and catechin possesses lower antioxidant activity than its polymers and oligomers. When antioxidant activity was eveluated by taking into consideration of total phenolic, it was seen that there was a strong correlation between them. As phenolic content increased, the antioxidant activities measured by DPPH, ABTS, and FRAP also increased for seeds, whole berry, and skin extracts.
Our findings are in agreement with the study of Yilmaz andToledo[Citation35] on Vitis vinifera cv. Muscadine, Merlot, Chardonnay. They found antioxidant activity ranging between 245.91 and 450.51 µmol of TE/g of DM for seed and 69.81 and 102.81 µmol of TE/g of DM for the skin. Different antioxidant activity values for grape berry, seeds, and skin have been reported by previous studies.[Citation6,Citation23,Citation34,Citation36,Citation37] As noted by Spigno et al.,[Citation36] it might be caused by differences in grape cultivar, extraction method, and antioxidant activity assay.
Fatty acid profile of grape seed oils
The fatty acid composition of Ekşikara grape seed oil is presented in . The most abundant fatty acid in Ekşikara grape seed oil was linoleic acid (C18:2). Oleic acid (C18:1), palmitic acid (C16:0), stearic acid (C18:0), arachidic acid (C20:0), and linolenic acid (C18:3) were also determined in this oil. Proportion of fatty acids of Ekşikara was similar to that reported by Crews et al.[Citation38] In agreement with Baydar andAkkurt,[Citation39] the highest fatty acid proportion was found for linoleic acid with 73.72% and the lowest for linolenic acid with 0.01%. The ranges of fatty acids in Ruby red, Muscadine, Concord, and Chardonnay grape seed oils were 66.0–75.3% for linoleic acid, 13.9–21.9% for oleic acid, 7.05–7.75% for palmitic acid, 2.52–4.72% for stearic acid, 0.25–1.14% for linolenic and trace amount—0.15% for arachidic acid.[Citation40]
Table 2. Relative amounts (percentage) of fatty acids of Ekşikara grape seed oil.
There was no significant difference in the percentage of linoleic, linolenic, and arachidic acid of the grape seed oilin terms of years and altitudes. However, palmitic acid, oleic acid, and stearic acid contents of oils showed variations as a result of the year and altitude. With increasing altitude, statistically significant decreases were recorded for stearic and oleic acid in Ekşikara seed oil.
Enviromental factors, such as altitude, air temperature, average annual relative humidity, and light intensity affect the accumulation of saturated, monounsaturated, and polyunsaturated fatty acid in plants.[Citation41] The effect of temperature on the changes in fatty acid profile of oils can be attributed to certain modifications in enzymatic activities.[Citation42]
According to Carvalho and Velásquez,[Citation43] cultivation of avocado at lower altitude resulted in lower percentage oleic acid in its oils. A previous study also indicated that oleic acid concentration in some oils of hazelnut varieties (Sivri, Yomra, and Karayaglı) increases with altitude.[Citation44] Palmitoleic, linoleic, stearic, and palmitic acids are the other fatty acids of avocado and hazelnut oils that are highly affected by altitude changes.[Citation43,Citation44]
Anthocyanin profile
Relative amounts of individual anthocyanin in Ekşikara grape berries and skins cultivated at different altitude and year are presented in . As a predominated anthocyanin in Ekşikara grape, malvidin-3-O-glucoside constituted 39.74 and 32.17% of total anthocyanin in the skin and grape berry, respectively. Peonidin-3-O-glucoside, delphinidin-3-O-glucoside, petunidin-3-O-glucoside, cyanidin-3-O-glucoside were also detected in descending order of their relative amount (). This result is in agreement with that reported by Kallithraka et al.[Citation45] The authors identified five anthocyanin monoglucosides and one coumaroylated anthocyanin in 17 cultivars of Vitis vinifera in Greece; and they also found malvidin-3-O-monoglucoside as dominant anthocyanin in all the cultivars.
Table 3. Relative amounts (percent) for anthocyanins found in whole berry and skin of Ekşikara grape.
Figure 5. HPLC chromatogram of Anthocyanins in Ekşikara (Vitis vinifera L.) berry harvested at A: 1500 m in year 2014; B: 1500 m in year 2015; and C: 1000 m in year 2014, (1: Delphinidin-3-O-glucoside, 2: Cyanidin-3-O-glucoside, 3: Petunidin-3-O-glucoside, 4: Peonidin-3-O-glucoside, 5: Malvidin 3-O-glucoside, 6–10: Acylated anthocyanins).

As shown in , statistically significant changes were observed in the relative amounts of cyanidin-3-O-glucoside, petunidin-3-O glucoside, malvidin-3-O-glucoside, and acylated anthocyanins (p < 0.05) in whole berries that were in the same vineyard in 2014 and 2015. In the whole grape, an increase from 31.17 to 42.11% occured in malvidin-3-O-glucoside in the following year. On the contrary, relative amounts of cyanidin-3-O-glucoside, petunidin-3-O glucoside and acylated anthocyanins were decreased. In the skin, except peonidin-3-O-glucoside, there was no significant difference in the proportion of anthocyanins by years. The increase of peonidin-3-O-glucoside in skin from 17.89 to 27.47% was found significant. As expected, same anthocyanins were determined in whole berry and skin extracts of the grapes cultivated at low and high altitude vineyards. On the other hand, the relative proportions of each anthocyanin were significantly changed compared to the higher altitude. The relative amount of malvidin-3-O-glucoside in both skin and whole berry increased with decreasing altitude. Conversely, petunidin-3-O-glucoside ratio was decreased from 11.91 to 10.25% in the whole berry and from 12.40 to 8.71% in the skin.
As previously mentioned, anthyocyanin synthesis in grapes are highly affected by enviromental factors and potential effects of altitude on anthocyanin synthesis are caused by the variations in temperature, sunlight exposure, and water deficits. UV radiation, moderate sunlight exposure, low temperature (close by 25°C), and water deficit enhance the anthocyanin accumulation by stimulating the expression of the structural and regulatory genes.[Citation26,Citation31] Xing et al.[Citation24] reported that anthocyanin accumulation in grapes at higher altitudes occurs more than lower altitudes.
The lower proportions of cyanidin-3-O-glucoside, peonidin-3-O-glucoside and petunidin 3-O-glucoside were found in whole berry cultivated at the lower altitude; and the acylated anthocyanin proportion increased significantly at higher altitude. This result is consistent with the findings of Fernandes de Oliveira et al.[Citation11] where they reported an increase in acylated anthocyanin proportion under light treatment.
Phenolic profile
shows the HPLC chromatogram of phenolic compounds in Ekşikara berry recorded at 280, 320, and 360 nm. Gallic acid, chlorogenic acid, caffeic acid, and gentisic acid were identified in Ekşikara berry as shown in . Chlorogenic acid was the most abundant non-flavanoid phenolic in the Ekşikara berries (180.9 mg/kg DW). It was followed by gallic acid, gentisic acid, and caffeic acid in a decreasing order. Gallic acid was detected only in the whole berries and seeds. This is in agreement with the results of Gómez-Alonso et al.[Citation46] Similar results were reported by Breksa et al.[Citation47] who determined the phenolic profiles of 16 cultivars and selections of Vitis vinifera. They did not find gallic acid in the grapes, except for two selections.
Table 4. Phenolic compounds of whole berry, seeds, and skin of Ekşikara cultivar (mg/kg DW).
Figure 6. HPLC chromatogram of phenolic compounds in Ekşikara (Vitis vinifera L.) berry cultivated at 1500 m in year 2014 (1:Gallic acid; 2: Procyanidin B1; 3: (+)-Catechin; 4: Procyanidin B2; 5: (-)-Epicatechin; 6: Epigallocatechin; 7: Epicatechin gallate; 8: Gentisic acid; 9: Chlorogenic acid; 10: Caffeic acid; 11: Rutin; 12: Kaempferol-3-glucoside; and 13: Isorhamnetin-3-glucoside).
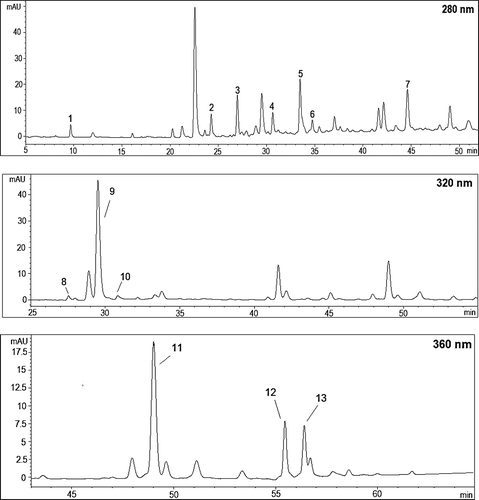
While no difference in the amount of gallic acid in both berries and seeds was observed in the lower altitude, chlorogenic acid amounts in berry and seed decreased with increasing altitude. As a result of increasing altitude, a significant decrease in gentisic acid amount of berries (p < 0.01) and caffeic acid amount of seeds (p < 0.05) observed. Except the caffeic acid content in seeds, negligible changes were observed in gentisic and caffeic acid amounts in all fractions according to the years.
Three flavonols, namely rutin (quercetin-rutinoside), kaempferol-3-glucoside, and isorhamnetin-3-glucoside, were detected in the berry. Rutin contents were found to be 183.50 mg/kg DW in the berry, 34.86 mg/kg DW in the seed, and 172.70 mg/kg DW in the skin. As the altitude increased, drastic decreases occurred in the amounts of rutin (p < 0.01) and isorhamnetin-3-glucoside (p < 0.05) in the whole berry. However, no significant changes were found in rutin and isorhamhetin-3-glucoside contents of skin and seeds as a result of higher altitude and year.
As can be seen in , flavanols were the main phenolics of Ekşikara grape. (+)-catechin, (-)-epicatechin, epigallocatechin, and epicatechin gallate were found in whole berries, skins, and seeds. Among the fractions, seeds had the highest epicatechin concentration. It was followed by the berry (374.70 mg/kg DW) and skin (96.21 mg/kg DW). Epicatechin, the most abundant monomeric flavanol in Ekşikara, was presented at highest concentration in seed (4784.00 mg/kg DW). The contents in whole berry and skin were 374.70 and 96.21 mg/kg DW, respectively.
Epicatechin and catechin are the most abundant flavanol-3-ols monomers present in grape varieties, and they are found at about equal concentrations. However, epicatechin is found slightly higher amounts in some grapes, especially in red grape cultivars.[Citation48] Flavanol monomer and dimer concentrations in the seeds of eleven grapes cultivated in Turkey ranged from 4.71 to 25.80 mg/g for catechin, 2.49 to 16.88 mg/g for epicatechin, 0.32 to 11.50 mg/g for epicatechin gallate, 0.79 to 2.55 mg/g for epigallocatechingallate, 0.79 to 5.96 mg/g for epigallocatechin, 0.56 to 1.94 mg/g for procyanidin B1 and 0.52 to 1.60 mg/g for procyanidin B2.[Citation37]
There were significant differences in the catechin concentrations of berries, harvested at different years and vineyards at different altitudes. Epigallocatechin gallate amount in the whole berry increased between the cultivation years. Epicatechin content of the skin was highly affected by the altitude (p < 0.05) and year (p < 0.01).
Procyanidin B2 was found in all fractions, while procyanidin B1 was detected only in the berry and seed of Ekşikara grape. The highest concentrations of procyanidin B1 and procyanidin B2 were observed in the seed as 4719.00 and 5987.00 mg/kg DW, respectively. Both cultivation year and altitude had no significant effect on procyanidin B1 and procyanidin B2 contents of all fractions.
These results have a number of similarities with findings of Xing et al.[Citation24] who noted a favorable role of high altitude on flavonoid synthesis in the grape berry. They reported lower values for most of the flavonols, flavan-3-ols and anthocyanins, identified in Cabernet Sauvignon grapes, at higher altitude cultivation. Generally, high altitude is associated with lower temperature and more intense sunlight exposure which acts as main stimulants in flavonoid synthesis.[Citation24,Citation49]
HPLC chromatogram of trans-resveratrol in Ekşikara grape skin is shown in . Skin, berry and seed of Ekşikara grape had 8.17, 2.27 and 1.97 mg/kg DW of trans-resveratrol, respectively. At lower altitude, a decrease was found in trans-resveratrol contents of skin and seed. Moreover, in skin, resveratol content increased from 8.17 to 18.00 mg/kg DW in following year. The trans-resveratrol content of grape is highly changeable according to the variety. Vincenzi et al.[Citation50] reported resveratrol contents in the skin of 21 Vitis vinifera cultivars ranging from 19 to 508 μg/g.
Conclusions
Ekşikara is a polyphenol-rich grape cultivar with high antioxidant activity, as in the case of many Vitis vinifera grapes that are subject to researchs. High antioxidant activity is bestowed by phenolic content and indivial phenolic compounds. Flavan-3-ols and tannins are found mainly in seed, while flavonols are mainly present in skin. Because of the containing seeds and skin, whole berry also exhibits high antioxidant activities. Some differences were observed in individual phenolics of grape by years associated with the climatic changes, whereas altitude had a drastic effect on phenolics in the grape. As altitude arised from 1000 to 1500 m, phenolic accumulation was up regulated and consequently antioxidant activity was increased. Altitude influence the fatty acid profile of grape seed oil as well as the phenolic profile of the grape seed, skin, and whole berry.
References
- Kennedy, J. Understanding Grape Berry Development. Practical Winery & Vineyard 2002, 4, 1–5.
- Naczk, M.; Shahidi, F. Phenolics in Cereals, Fruits and Vegetables: Occurrence, Extraction and Analysis. Journal of Pharmaceutical and Biomedical Analysis 2006, 41, 1523–1542.
- Montealegre, R.R.; Peces, R.R.; Vozmediano, J.C.; Gascueña, J.M.; Romero, E.G. Phenolic Compounds in Skins and Seeds of Ten Grape Vitis Vinifera Varieties Grown in a Warm Climate. Journal of Food Composition and Analysis 2006, 19, 687–693.
- Yilmaz, Y.; Toledo, R.T. Health Aspects of Functional Grape Seed Constituents. Trends in Food Science & Technology 2004, 15, 422–433.
- Adams, D.O. Phenolics and Ripening in Grape Berries. American Journal of Enology and Viticulture 2006, 57, 249–256.
- Poudel, P.R.; Tamura, H.; Kataoka, I.; Mochioka, R. Phenolic Compounds and Antioxidant Activities of Skins and Seeds of Five Wild Grapes and Two Hybrids Native to Japan. Journal of Food Composition and Analysis 2008, 21, 622–625.
- Nakabayashi, R.; Yonekura‐Sakakibara, K.; Urano, K.; Suzuki, M.; Yamada, Y.; Nishizawa, T.; Matsuda, F.; Kojima, M.; Sakakibara, H.; Shinozaki, K. Enhancement of Oxidative and Drought Tolerance in Arabidopsis by Overaccumulation of Antioxidant Flavonoids. The Plant Journal 2014, 77, 367–379.
- Downey, M.O.; Harvey, J.S.; Robinson, S.P. The Effect of Bunch Shading on Berry Development and Flavonoid Accumulation in Shiraz Grapes. Australian Journal of Grape and Wine Research 2004, 10, 55–73.
- Teixeira, A.; Eiras-Dias, J.; Castellarin, S.D.; Gerós, H. Berry Phenolics of Grapevine Under Challenging Environments. International Journal of Molecular Sciences 2013, 14, 18711–18739.
- Kumsta, M.; Pavlousek, P.; Kárník, P. Use of Anthocyanin Profiles When Differentiating Individual Varietal Wines and Terroirs. Food Technology and Biotechnology 2014, 52, 383.
- Fernandes de Oliveira, A.; Mercenaro, L.; Del Caro, A.; Pretti, L.; Nieddu, G. Distinctive Anthocyanin Accumulation Responses to Temperature and Natural UV Radiation of Two Field-Grown Vitis Vinifera L. Cultivars. Molecules 2015, 20, 2061–2080.
- Gladstones, J. Wine, Terroir and Climate Change. Wakefield Press: Kent Town, South Australia, 2011.
- Akbulut, M.; Coklar, H. Effect of Adsorbent and Ion Exchange Resin Applications on Total Phenolic Content and Antioxidant Activity of White and Red Grape Juices. Selcuk Journal of Agriculture and Food Sciences 2015, 29, 31–33.
- Hagerman, A.E.; Butler, L.G. Protein Precipitation Method for the Quantitative Determination of Tannins. Journal of Agricultural and Food Chemistry 1978, 26, 809–812.
- Benzie, I.; Strain, J. Ferric Reducing/Antioxidant Power Assay: Direct Measure of Total Antioxidant Activity of Biological Fluids and Modified Version for Simultaneous Measurement of Total Antioxidant Power and Ascorbic Acid Concentration. Methods in Enzymology 1998, 299, 15–27.
- Re, R.; Pellegrini, N.; Proteggente, A.; Pannala, A.; Yang, M.; Rice-Evans, C. Antioxidant Activity Applying An Improved ABTS Radical Cation Decolorization Assay. Free Radical Biology and Medicine 1999, 26, 1231–1237.
- Brand-Williams, W.; Cuvelier, M.-E.; Berset, C. Use of a Free Radical Method to Evaluate Antioxidant Activity. LWT–Food Science and Technology 1995, 28, 25–30.
- Demir, N.; Yildiz, O.; Alpaslan, M.; Hayaloglu, A. Evaluation of Volatiles, Phenolic Compounds and Antioxidant Activities of Rose Hip (Rosa L.) Fruits in Turkey. LWT–Food Science and Technology 2014, 57, 126–133.
- Gecgel, U.; Velioglu, S.D.; Velioglu, H.M. Investigating Some Physicochemical Properties and Fatty Acid Composition of Native Black Mulberry (Morus Nigra L.) Seed Oil. Journal of the American Oil Chemists’ Society 2011, 88, 1179–1187.
- Williams, S. Official Methods of Analysis of the Association of Official Analytical Chemists. Arlington, Virginia, USA, 1984.
- Destaillats, F.D.R.; Cruz-Hernandez, C.; Giuffrida, F.; Dionisi, F. Identification of the Botanical Origin of Pine Nuts Found in Food Products By Gas-Liquid Chromatography Analysis of Fatty Acid Profile. Journal of Agricultural and Food Chemistry 2010, 58, 2082–2087.
- Kyraleou, M.; Kotseridis, Y.; Koundouras, S.; Chira, K.; Teissedre, P.-L.; Kallithraka, S. Effect of irrigation Regime on Perceived Astringency and Proanthocyanidin Composition of Skins and Seeds of Vitis Vinifera L. cv. Syrah Grapes Under Semiarid Conditions. Food Chemistry 2016, 203, 292–300.
- Xu, C.; Zhang, Y.; Cao, L.; Lu, J. Phenolic Compounds and Antioxidant Properties of Different Grape Cultivars Grown in China. Food Chemistry 2010, 119, 1557–1565.
- Xing, R.R.; He, F.; Xiao, H.L.; Duan, C.Q.; Pan, Q.H. Accumulation Pattern of Flavonoids in Cabernet Sauvignon Grapes Grown in a Low-Latitude and High-Altitude Region. South African Journal of Enology and Viticulture 2015, 36, 32–43.
- De Orduna, R.M. Climate Change Associated Effects on Grape and Wine Quality and Production. Food Research International 2010, 43, 1844–1855.
- Castellarin, S.D.; Pfeiffer, A.; Sivilotti, P.; Degan, M.; Peterlunger, E.; Di Gaspero, G. Transcriptional Regulation of Anthocyanin Biosynthesis in Ripening Fruits of Grapevine Under Seasonal Water Deficit. Plant, Cell & Environment 2007, 30, 1381–1399.
- Dokoozlian, N.; Kliewer, W. Influence of Light on Grape Berry Growth and Composition Varies During Fruit Development. Journal of the American Society for Horticultural Science 1996, 121, 869–874.
- Schultz, H. Climate Change and Viticulture: A European Perspective on Climatology, Carbon Dioxide and UV‐B Effects. Australian Journal of Grape and Wine Research 2000, 6, 2–12.
- Ristic, R.; Downey, M.O.; Iland, P.G.; Bindon, K.; Francis, I.L.; Herderich, M.; Robinson, S.P. Exclusion of Sunlight from Shiraz Grapes Alters Wine Colour, Tannin and Sensory Properties. Australian Journal of Grape and Wine Research 2007, 13, 53–65.
- Cohen, S.D.; Tarara, J.M.; Kennedy, J.A. Assessing the Impact of Temperature on Grape Phenolic Metabolism. Analytica Chimica Acta 2008, 621, 57–67.
- He, F.; Mu, L.; Yan, G.-L.; Liang, N.-N.; Pan, Q.-H.; Wang, J.; Reeves, M.J.; Duan, C.-Q. Biosynthesis of Anthocyanins and Their Regulation in Colored Grapes. Molecules 2010, 15, 9057–9091.
- Ky, I.; Teissedre, P.-L. Characterisation of Mediterranean Grape Pomace Seed and Skin Extracts: Polyphenolic Content and Antioxidant Activity. Molecules 2015, 20, 2190–2207.
- Rice-Evans, C.; Miller, N.; Paganga, G. Antioxidant Properties of Phenolic Compounds. Trends in Plant Science 1997, 2, 152–159.
- Spranger, I.; Sun, B.; Mateus, A.M.; de Freitas, V.; Ricardo-da-Silva, J.M. Chemical Characterization and Antioxidant Activities of Oligomeric and Polymeric Procyanidin Fractions from Grape Seeds. Food Chemistry 2008, 108, 519–532.
- Yilmaz, Y.; Toledo, R.T. Major Flavonoids in Grape Seeds and Skins: Antioxidant Capacity of Catechin, Epicatechin, and Gallic Acid. Journal of Agricultural and Food Chemistry 2004, 52, 255–260.
- Spigno, G.; Tramelli, L.; De Faveri, D.M. Effects of Extraction Time, Temperature and Solvent on Concentration and Antioxidant Activity of Grape Marc Phenolics. Journal of Food Engineering 2007, 81, 200–208.
- Bozan, B.; Tosun, G.; Özcan, D. Study of Polyphenol Content in the Seeds of Red Grape (Vitis Vinifera L.) Varieties Cultivated in Turkey and Their Antiradical Activity. Food Chemistry 2008, 109, 426–430.
- Crews, C.; Hough, P.; Godward, J.; Brereton, P.; Lees, M.; Guiet, S.; Winkelmann, W. Quantitation of the Main Constituents of Some Authentic Grape-Seed Oils of Different Origin. Journal of Agricultural and Food Chemistry 2006, 54, 6261–6265.
- Baydar, N.G.; Akkurt, M. Oil Content and Oil Quality Properties of Some Grape Seeds. Turkish Journal of Agriculture and Forestry 2001, 25, 163–168.
- Lutterodt, H.; Slavin, M.; Whent, M.; Turner, E.; Yu, L.L. Fatty Acid Composition, Oxidative Stability, Antioxidant and Antiproliferative Properties of Selected Cold-Pressed Grape Seed Oils and Flours. Food Chemistry 2011, 128, 391–399.
- Yuemei, C.; Junmin, D.; Caihong, Z. The Analysis on Fat Characteristics of Walnut Varieties in Different Production Areas of Shanxi Province. Journal of Plant Studies 2014, 3, 28.
- Izquierdo, N.; Aguirrezábal, L.; Martínez-Force, E.; Garcés, R.; Paccapelo, V.; Andrade, F.; Reid, R.; Zambelli, A. Effect of Growth Temperature on the High Stearic and High Stearic-High Oleic Sunflower Traits. Crop and Pasture Science 2013, 64, 18–25.
- Carvalho, C.P.; Velásquez, M.A. Fatty Acid Content of Avocados (Persea Americana Mill. Cv. Hass) in Relation to Orchard Altitude and Fruit Maturity Stage. Agronomía Colombiana 2015, 33, 220.
- Beyhan, O.; Elmastas, M.; Genc, N.; Aksit, H. Effect of Altitude on Fatty Acid Composition in Turkish Hazelnut (Coryllus Avellana L.) Varieties. African Journal of Biotechnology 2013, 10, 16064–16068.
- Kallithraka, S.; Mohdaly, A.A.-A.; Makris, D.P.; Kefalas, P. Determination of Major Anthocyanin Pigments in Hellenic Native Grape Varieties (Vitis Vinifera sp.): Association with Antiradical Activity. Journal of Food Composition and Analysis 2005, 18, 375–386.
- Gómez-Alonso, S.; García-Romero, E.; Hermosín-Gutiérrez, I. HPLC Analysis of Diverse Grape and Wine Phenolics Using Direct Injection and Multidetection by DAD and Fluorescence. Journal of Food Composition and Analysis 2007, 20, 618–626.
- Breksa, A.P.; Takeoka, G.R.; Hidalgo, M.B.; Vilches, A.; Vasse, J.; Ramming, D.W. Antioxidant Activity and Phenolic Content of 16 Raisin Grape (Vitis Vinifera L.) Cultivars and Selections. Food Chemistry 2010, 121, 740–745.
- Hatzidimitriou, E.; Nenadis, N.; Tsimidou, M.Z. Changes in the Catechin and Epicatechin Content of Grape Seeds on Storage Under Different Water Activity (A W) Conditions. Food Chemistry 2007, 105, 1504–1511.
- Alonso-Amelot, M.E.; Oliveros-Bastidas, A.; Calcagno-Pisarelli, M.P. Phenolics and Condensed Tannins of High Altitude Pteridium Arachnoideum in Relation to Sunlight Exposure, Elevation, and Rain Regime. Biochemical Systematics and Ecology 2007, 35, 1–10.
- Vincenzi, S.; Tomasi, D.; Gaiotti, F.; Lovat, L.; Giacosa, S.; Torchio, F.; Segade, S.R.; Rolle, L. Comparative Study of the Resveratrol Content of Twenty-One Italian Red Grape Varieties. South African Journal of Enology and Viticulture 2013, 34, 30–33.