ABSTRACT
The present study describes the screening of eight herbs namely Cosmos caudatus, Pluchea indica, Lawsonia inermis, Carica papaya, Piper betle, Andrographis paniculata, Pereskia bleo, and Melicope lunu based on their anti-obesity and antioxidant activities. Out of all tested herbs, Cosmos caudatus demonstrated excellent anti-obesity and antioxidant potential with pancreatic lipase inhibitory effect (21.7 ± 1.3%) and DPPH radical scavenging activity (IC50 value of 31.98 ± 1.22 µg/mL). Cosmos caudatus was selected for further studies and extracting solvent composition with best anti-obesity and antioxidant potential was identified. Hundred percent Cosmos caudatus ethanolic extract was found to be the most effective and showed highest anti-obesity and antioxidant activities. Moreover, metabolite profiling of Cosmos caudatus extract was also carried out using UHPLC-MS/MS. The analysis depicted the presence of quercetin-3-rhamnoside, catechin, kaempherol, kaempherol glucoside, quercetin, quercetin-3-glucoside, quercetin-O-pentoside, quercetin-rhamnosyl galactoside, quinic acid, 1-caffeyolquinic acid, monogalloyl glucose, and procyanidin B1. Results revealed Cosmos caudatus as promising medicinal plant for the development of new functional food with prodigious applications in obesity.
Introduction
Obesity is a common disorder usually caused by the interaction of genetic, nutritional, and environmental factors. It has now become one of the most important health issues of the modern society around the world.[Citation1] It is often associated with other diseases such as arteriosclerosis, hypertension, cancer, diabetes, and osteoarthritis.[Citation2,Citation3] The incidence of obesity is increasing exponentially, and it has been revealed that about 500 million adults are obese worldwide.[Citation4]
Pancreatic lipase (PL), secreted by the pancreas, is a key enzyme, responsible for the digestion of 50–70% of fat into monogylceride and free fatty acids for absorption by the entherocytes. Inhibition in the digestion and absorption of fat usually reduces its accumulation in the adipose tissue.[Citation5] Therefore, one of the key targets for anti-obesity agent is inhibition of PL.[Citation6] On the other hand, lipoprotein lipase (LPL) is a rate-limiting enzyme that hydrolyzes the triglycerides (TG)-rich lipoproteins, chylomicrons, and very low-density lipoproteins (VLDL), resulting into the release of non-esterified fatty acids (NEFA) and monoacylglycerol. These fatty acids and monoacylglycerol are either used by the muscles for metabolic energy or re-esterified into TG and stored as neutral lipids in adipose tissue. Any imbalance in LPL activity affects the distribution of TG between muscle and adipose tissue and thus influences obesity.[Citation1,Citation7] Consequently, compounds, which can inhibit activity of these lipases, are supposed to function as anti-obesity agents.[Citation8]
There have been extensive claims on the beneficial effects of various plant extracts especially based on their antioxidant properties.[Citation9–Citation12] It is well established that polyphenols found ubiquitously in the plant kingdom are effective antioxidant. They exhibit ability to scavenge free radicals through inhibition of some enzymes or by chelation of trace metals involved in the production of free radicals and are also able to protect the antioxidant defense system.[Citation13] Escalation in oxidative damage and oxidative stress markers are associated with several diseases such as obesity, diabetis, Alzheimer’s diseases, other neurodegenerative diseases, cancer, and atherosclerosis.[Citation14–Citation16] It is important to note that any actions that can reduce oxidative stress would be therapeutically beneficial.[Citation17–Citation19] It is also important to relate the anti-obesity and antioxidant properties of these commonly consumed herbs.
Obesity is associated with a state of excessive oxidative stress, which plays an important role in the pathogenesis of many diseases.[Citation20,Citation21] The side effects of some anti-obesity drugs and synthetic antioxidants have prompted scientists to search for safe and effective natural bioactive compounds that can target both anomalies. The present study was therefore aimed to evaluate the antioxidant and anti-obesity effects of Cosmos caudatus, Pluchea indica, Lawsonia inermis, Carica papaya, Piper betle, Andrographis paniculata, Pereskia bleo, and Melicope lunu in vitro and to determine the bioactive compounds (in the most active extract) that may be responsible for the bioactivities measured. The obtained information may help in seeking potential herb with excellent anti-obesity and antioxidant attributes.
Materials and methods
Plant materials and reparation of extracts
Fresh leaves of Melicope lunu, Carica papaya, Pluchea indica, Lawsonia inermis, Pereskia bleo, Andrographis paniculata, Cosmos caudatus, and Piper betle were obtained from University Agricultural Farm, identified by botanist from Faculty of Forestry, Universiti Putra Malaysia (UPM), Malaysia, and the voucher specimens were submitted in herbarium with vouture numbers H016, H017, H018, H019, H020, H021, H022, and H023, respectively. The leaf extracts of the plants were prepared using the modified method of Chang et al.[Citation22] Fresh leaves were cut and washed under running tap water, followed by freeze drying for two days. The dried plant material was ground to powder and sieved for homogeneity. Absolute ethanol (100 mL) or aqueous ethanol with different ratios of ethanol and water (100:0, 80:20, 60:40, 50:50, and 40:60) was used as extracting solvent to extract 10 grams of dried material for 24 hours at 40°C. The extracts were filtered, and solvent was evaporated off using rotary evaporator at 40°C. The resulting viscous extract was freeze-dried to ensure complete removal of water. Finally, the dried crude extract was diluted to required concentration for further experimental work.
In vitro pancreatic lipase (PL) inhibitory assay
Plant extracts were prepared at different concentrations in 0.01 M Tris-HCL buffer. Porcine pancreatic lipase was dissolved in 0.01 M Tris-HCL buffer (25 units/mL). The substrate was prepared using the modified method of Fox and Stepaniak.[Citation23] Briefly, olive oil (10% v/v) was mixed with Arabic gum mixture (10% w/v in 0.1 M Tris-HCL buffer, pH 8, 0.5 M NaCl, and 20 mM CaCl2) using a homogenizer. Inhibition of PL by plant extracts was determined using the method reported by Fukumoto et al. with some modifications.[Citation24] Lipase solution (0.2 mL) was allowed to react with 0.5 mL of plant extract for 30 minutes at 4°C. Substrate emulsion (2 mL) was then added and incubated for 30 minutes at 37°C. One-mL acetone and ethanol (1:1) mixture was used to stop the reaction and titrated with 0.02 M NaOH until reached pH 9.4. Auto titrator was used to perform the titrations (Metrohom, 785 DMP Titrino). The experiment was repeated thrice for each sample extract. The amount of free fatty acid (FFA) liberated was reflected by the amount of base required by the incubation mixture which is equivalent to PL activity. Control sample was equivalent to 100% enzyme activity. Percent inhibition was calculated based on the following equation:
where, Vsample is the amount of base added to sample, and Vcontrol is the amount of base added to control.
In vitro lipoprotein lipase (LPL) inhibitory assay
A modified method by Schotz et al.[Citation25] was used for the preparation of substrate. An activator consisted of Apo C-II from human plasma and diluted to 1 μg/mL with 0.002 M Tris HCl (pH 8.0) was prepared. In the preparation of substrate, 0.6 mL triolein, 24 mL apo C-II, 3.6 mL of 1% BSA solution, 3.6 mL of 1% triton X-100, and 28.8 mL of 0.2 Tris HCl buffer (pH 8.0) were mixed. The mixture was then sonicated in ice for 3 minutes. Enzyme LPL from bovine milk was prepared by diluting with 0.02 M Tris HCl (pH 8.0) to a concentration of 25 units/mL. The LPL activity was then determined using a method reported by Chung and Scanu.[Citation26] Briefly, 0.5 mL of previously diluted LPL was added to 0.5 mL of extracts and epicatechin (100 ppm) in test tubes followed by pre-incubation at 4°C for 30 minutes. Then, 1 mL of substrate emulsion was added to the mixture of enzyme and extracts, followed by incubation in waterbath at 37°C, to initiate hydrolysis. The reaction was stopped with the addition of 1 mL of 1 M NaCl. Control samples were consisted of mixture of enzyme and substrate only. The liberated free fatty acids (FFA) were titrated with 0.01 M NaOH until pH 9.4 using autotitrator (Metrohom, 785 DMP Titrino). The amount of liberated free fatty acid (FFA) was reflected by the amount of base required by the incubation mixture which is equivalent to LPL activity. Control sample was equivalent to 100% enzyme activity. The experiment was repeated thrice for each sample extract, and percent inhibition was calculated.
Total phenolic content (TPC)
Total phenolic content of plant extracts was determined according to the method of Verza et al.[Citation27] Folin Ciocalteu reagent (0.5 mL), plant extracts (0.5 mL), and 7% sodium carbonate (10 mL) were allowed to react at room temperature for 1 hour. Absorbance of the resulting blue color complex was noted at 725 nm (Shimadzu UV Visible Spectrophotometer, UV-1650 PC, Japan). The concentration levels used were in the range from 0.02 to 0.1 mg/mL. A standard curve was plotted with gallic acid standard, and the phenolic content was expressed as mg gallic acid equivalent (GAE)/g extract.
Total flavonoid content (TFC)
Total flavonoid content of plant extracts was determined spectrophotometrically according to the method adapted from Quettier-Deleu et al.[Citation28] The method was based on the formation of a flavonoid–aluminum complex having the absorbance at 430 nm. Rutin was used as standard for calibration curve. Diluted samples (1 mL) were separately mixed with 1 mL of 2% methanolic aluminum chloride solution. After incubation at room temperature for 15 min, the absorbance of the reaction mixture was measured at 430 nm with UV–Vis spectrophotometer and total flavonoids content was expressed as mg rutin equivalent (RE)/g extract.
DPPH (2,2-diphenyl-2-picrylhydrazyl) radical scavenging assay
Scavenging activity of samples was assessed according to the method described by Brand-Williams et al.[Citation29] Antioxidant activity was expressed as IC50 (defined by the concentration of samples required to scavenge 50% of the free radicals). All the experiments were performed in triplicate using ascorbic acid, BHA, and α-tocopherol as positive controls. Plant extract (250 µL) at different concentrations was added to 1.75 mL of 25 ppm DPPH in methanol. All test samples were prepared in 24-well plates. The mixture was left to stand for 30 minutes at room temperature in the dark. Absorbance was then noted using spectrophotometer (Biotek EL800 Microplate Reader) at 517 nm. The readings were compared with those of the blanks, and the percent radical scavenging activity of samples was then calculated using the following equation followed by the measurement of IC50 values:
β-Carotene–Linoleate bleaching assay
The antioxidant activity of plant extracts was also determined based on β-carotene bleaching method developed by Velioglu et al.[Citation30] BHT was used as the standard, and all the tests were performed in triplicate. β-carotene (0.2 mg in 1 mL chloroform), linoleic acid (0.02 mL), and Tween 20 (0.2 mL) were transferred into a round-bottomed flask, and the mixture was added to 0.2 mL of plant extracts or standard or ethanol (as control). Chloroform was removed using a rotary evaporator. Following evaporation, 50 mL of distilled water was added to the mixture and shaked vigorously to form emulsion. Two-mL aliquots of the emulsion were pipetted into the test tubes and immediately placed in water bath at 50°C. The absorbance measurements were then taken at 20-minute intervals for 2 hours at 470 nm. Degradation rates (DR) were calculated according to first-order kinetics, using the following equation:
where a is the initial absorbance (470 nm) at time 0, b is the absorbance (470 nm) at 20, 40, 60, 80, 100, or 120 minutes, t is the time. Antioxidant activity (AA) was expressed as percent of inhibition relative to the control, using the following formula:
High-performance liquid chromatography (HPLC) analysis
The HPLC analysis of plant extract was carried out according to the protocol developed by Crozier et al. with some modifications.[Citation31] The samples were prepared by dissolving 10 mg of crude ethanolic plant extracts in 1 mL of methanol. The resulting solution was then filtered prior to analysis. The standards were prepared by dissolving 1 mg of catechin, epicatechin, rutin, quercetin-3-rhamnoside, quercetin, myricetin, fisetin, hesperitin, naringin, and genistein in 1 mL of HPLC grade methanol. The sample and standards were analyzed using HPLC system (Waters Delta 600 with 600 Controller) with photodiode array detector (Waters 996). A Phenomenex-Luna (5 µm) PFP-2 (4.6 mm i.d. × 250 mm) column was used, and for elution of the constituents, two solvents denoted as A and B were employed. Solvent A was 0.1% formic acid in deionized water, and solvent B was acetonitrile. Gradient elution was performed as followed for solvent A: 0 min (95%), 12–20 min (75%), 22–30 min (85%), 35 min (95%). The flow rate used was 1 mL/min, and the injection volume was 10 µL. The detector was set at the range of 210–366 nm. The retention times, peak areas, and UV spectra of the major peaks were analyzed. Rutin, catechin, and quercetin-3-rhamnoside standards (20–140 μg/mL) were further used for quantification based on standard curve.
Liquid chromatography–mass spectroscopy (LC-MS/MS) analysis
Crude ethanolic plant extracts (5 mg) were suspended in 1 mL methanol and then filtered through a PTFE filter (pore size 0.22 µm). The analysis was carried out on a Linear Ion Trap Quadrupole LC/MS/MS Mass Spectrometer (AB Sciex 3200QTrap LCMS/MS with Perkin Elmer FX 15 UHPLC system). LCMS analysis was carried out according to internal protocol developed by instruments manufacturer (AB Sciex, Canada). Scan ranges from 100 to 1200 m/z for full scan and 50–1200 m/z for MS/MS scan. The chromatographic separation was performed on a Zorbax C18 column (150 mm × 4.6 mm × 5 Um) with a gradient mobile phase-comprising water (solvent A) and acetonitrile (solvent B) (each with 0.1% formic acid and 5 mM ammonium formate). The ionization mode used was negative. The gradient program started from 10% B to 90% B for a period of 0.01 min to 8.0 min, hold for 2 min and back to 10% B in 0.1 min and re-equilibrated for 3 minutes with a flow rate of 800 μl/minute, and an injection volume of 20 µL was used. The UHPLC-MS/MS system was equipped with Analyst 1.5.2 (a mass spectrometric software) and an ACD spectral library (ACD Labs, Toronto, ON, Canada). The resolved peaks were identified based on their accurate masses, molecular ion peaks, mass fragmentation patterns, comparison with ACD mass spectral library, and literature data.
Statistical analysis
All the experimental data were expressed as mean ± standard deviation. Data were analyzed for one-way ANOVA using SPSS 20.0. Duncan’s multiple-range test was used to assess difference between means. A significant difference was considered at the level of p < 0.05.
Results
Medicinal plants with good antioxidant potential and ability to inhibit PL and LPL activities are known to exhibit excellent anti-obesity properties. Inhibition of these enzymes helped in reducing the fat digestion, absorption, and slowed down the deposition of fat into adipose tissue. The detailed results regarding the anti-obesity and antioxidant effects of understudy plant extracts are described as follows.
Screening of herbs as potential anti-obesity and antioxidant agents
Pancreatic lipase inhibitory activities of different plant extracts
Pancreatic lipase inhibitory characteristics were examined to evaluate the potential of understudy herbal extracts as anti-obesity agents. Results on the inhibitory activity of plant extracts toward pancreatic lipase are illustrated in . Orlistat and epicatechin were used as synthetic and natural positive controls, respectively. The herbal extracts were assayed at various concentrations ranging from 62.5 ppm (0.06 mg/mL) to 1000 ppm (1 mg/mL). All the plant extracts showed increase in inhibitory effect as the concentration increased, except for Pereskia bleo and Carica papaya extracts, whereas the percent inhibition was highest at 500 ppm (0.5 mg/mL). Cosmos caudatus extract demonstrated highest inhibition of 21.7 ± 1.3% at 1000 ppm, which was not significantly (p > 0.05) different with that of Lawsonia inermis with 20.6 ± 2.0% inhibition. Epicatechin showed moderate inhibition (18.6 ± 2.4%), while the lowest inhibition (10.4 ± 1.0%) was shown by Melicope lunu. At all concentrations, orlistat exhibited the highest activity ranging from 36.6 ± 1.8 to 26.0 ± 3.2%.
Table 1. Inhibitory effects (%) of different plant extracts on pancreatic lipase at different concentrations.
DPPH free radical scavenging capacity
DPPH free radical scavenging activities of the understudy herbs are described in . The IC50 of the understudy plant extracts varied from 31.98 to 1304.95 µg/mL. Results revealed that Cosmos caudatus and Pluchea indica were the potential-free radical scavengers with IC50 lesser than 50 µg/mL. Interestingly, the antioxidant capacity of both extracts was nonsignificantly different (p > 0.05) from that of ascorbic acid, BHA, and α-tocopherol. Lawsonia inermis and P. bettle showed moderate antioxidant activities with IC50 less than 100 µg/mL, while Melicope lunu, Carica papaya, Pereskia bleo, and Andrographis paniculata were depicted to be the weakest free radical scavengers with IC50 exceeding 150 µg/mL.
Table 2. DPPH, β-Carotene-linoleic scavenging activity and TPC in different plant extracts.
β-Carotene–Linoleate bleaching assay
It was found that 250 ppm Lawsonia inermis exhibited the highest antioxidant activity based on β-Carotene–linoleate bleaching assay (82.0 ± 1.5%) followed by Carica papaya, Pluchea indica, Melicope lunu, Cosmos caudatus, and Pereskia bleo, whereas the lowest activity was shown by Andrographis paniculata at 15.1 ± 2.7% (). At the same concentration, the antioxidant activities of understudy plant extracts were depicted to be significantly lower than that of BHA (93.5 ± 1.8%).
Total phenolic content (TPC)
Based on the results related to TPC (), the plant extracts can be divided into three ranges based on their TPC, that is, high, middle, and low ranges of TPC at <100, 100–500, and >500 mg GAE/g extract. Among the plant extracts, Pluchea indica and Cosmos caudatus were revealed to consist of high TPC. Piper betle, Lawsonia inermis, and Melicope lunuand Carica papaya showed moderate TPC, whereas the least TPC was depicted by Pereskia bleo and Andrographis paniculata. The high phenolic content of Pluchea indica and Cosmos caudatus extracts might be responsible for their antioxidant activities. The total phenolic content of plant extracts ranged from 715.11 ± 14.69 mg GAE/g extract (Pluchea indica) to 50.00 ± 2.35 mg GAE/g extract (Andrographis paniculata).
Study for best extracting solvent composition for cosmos caudatus with optimal anti-obesity and antioxidant potential
The first part of the study revealed that Cosmos caudatus is the most potent plant with anti-obesity effects and exhibited exceptionally high antioxidant capacity. Therefore, this plant was selected for further study. Different ratios of ethanol and water (100:0, 80:20, 60:40, 50:50, and 40:60) as extracting solvent systems were employed with the objective to identify the best extracting solvent composition for its extraction. Extracting solvent consisted of ethanol and water was chosen in this study for the extraction due to its safe use comparing to methanol, acetone, and other organic solvents.[Citation32–Citation34]
Pancreatic lipase inhibitory activity of cosmos caudatus extracts
The results obtained in this study (), revealed that 100% ethanolic extract (at 1000 ppm) showed the highest inhibition (21.8 ± 1.53%), which differs nonsignificantly (p > 0.05) with 19.9 ± 1.39% inhibition observed at 500 ppm concentration. However, all the ethanolic extracts showed significantly (p < 0.05) lower activities compared to that of Orlistat.
Table 3. Inhibitory effects (%) of different extracts of Cosmos caudatus on pancreatic lipase at different concentrations.
Lipoprotein lipase (LPL) inhibitory activity of cosmos caudatus extracts
The ethanolic extracts of Cosmos Caudatus (40, 50, 60, 80 and 100%) showed different LPL inhibition activities ranging from 11.9 to 19.9% (). At a concentration of 0.1 mg/mL, 60% Cosmos caudatus ethanolic extract showed the lowest activity (11.9 ± 1.1%), while 100% ethanol extract showed the highest inhibition of LPL (19.9 ± 1.1%). The 40, 50, and 60% Cosmos caudatus ethanolic extracts did not show any significant difference (p > 0.05) in their inhibitory effect when compared to that of pure epicatechin (12.7 ± 1.7%).
Figure 1. Inhibitory effects (%) of different extracts of Cosmos caudatus and Epicatechin on lipoprotein lipase (0.1 mg/mL). Values with different letters indicate significance difference (p < 0.05) measured by one-way ANOVA, SPSS 20. All experiments were repeated three times with triplicate readings. Error bars represent the standard deviation.
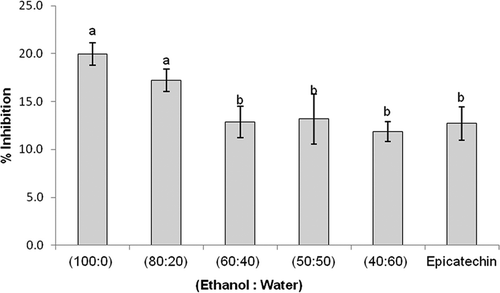
Total phenolic content of cosmos caudatus extracts
The total phenolic content of 40, 50, 60, 80, and 100% Cosmos caudatus ethanolic extracts is shown in . Almost similar trend was depicted, whereby 100% ethanolic extract showed the highest phenolic content (865.8 ± 5.0 mg GAE/g extract) and was significantly higher (p < 0.05) than that of 80, 60, 50, and 40% ethanolic extracts with total phenolic content 473.8 ± 43.4, 377.1 ± 36.4, 311.8 ± 13.3, and 266.3 ± 53.7 mg GAE/g extract, respectively.
Table 4. DPPH, TPC and TFC of different extracts of Cosmos caudatus.
Total flavonoid content of cosmos caudatus extracts
describes the total flavonoid content of different Cosmos caudatus ethanolic extracts. Similar to that of TPC and DPPH assay results, 100% ethanolic extract showed the highest flavonoid content (398.8 ± 34.8 mg RE/g extract) and was significantly (p < 0.05) higher than that of 80, 60, 50, and 40% ethanolic extracts with flavonoid content 234.0 ± 8.4, 207.2 ± 33.8, 152.6 ± 23.8, and 125.2 ± 15.5 mg RE/g extract, respectively.
DPPH free radical scavenging capacity of cosmos caudatus extracts
The results showed that the highest scavenging activity was shown by 100% ethanolic extract followed by 80, 60, 50, and 40% ethanolic extracts (). The 100% Cosmos caudatus ethanolic extract showed exceptionally high antioxidant activity with a nonsignificant difference (p > 0.05) to that of BHA and ascorbic acid.
Correlation of bioactive compounds with antioxidant and anti-obesity activity
Pearson’s correlation coefficients (r) between total phenolic content, total flavonoid content, antioxidant capacity, and lipases enzyme inhibitory activities of Cosmos caudatus extracts were determined. The results showed strong positive correlations between antioxidant activity (DPPH) and both phenolic (r = 0.708) and flavonoid (r = 0.766) content. The same trend was also seen between anti-obesity (PL and LPL) and phenolic (r = 0.935, 0.845) and flavonoid (r = 0.945, 0.835) contents.
HPLC and UHPLC-MS/MS analysis of cosmos caudatus extract
HPLC and UHPLC-MS/MS analysis of Cosmos caudatus extracts were carried out based on literature data, mass spectral library, online databases, and available reference standards.[Citation35–Citation37] Ten flavonoid standards (catechin, epicatechin, quercetin-3-rhamnoside, rutin, quercetin, myricetin, fisetin, hesperitin, naringin, and genistein) were used to determine the flavonoid content of Cosmos caudatus extract, and the selection was based on the flavonoids, which are commonly found in plants, fruits, and vegetables. Out of ten standards used, three flavonoids were detected and quantified by HPLC. Quercetin-3-rhamnoside was depicted to be the major compound based on its high quantity (52.9 ± 5.60 mg/g) when compared to that of catechin (34.1 ± 3.38 mg/g) and rutin (6.1 ± 0.53 mg/g).
Furthermore, LC-MS/MS analysis of Cosmos caudatus extract shows the presence of 1-caffeyolquinic acid, catechin, kaempherol, kaempherol glucoside, quercetin, quercetin-3-glucoside, quercetin-O-pentoside, quercetin-rhamnosyl galactoside, quinic acid, monogalloyl glucose, and procyanidin B1 (). The findings were in agreement with the previous reports on Cosmos caudatus.[Citation35–Citation50]
Table 5. Identified metabolites of Cosmos caudatus extract with their mass spectral characteristics.
Discussion
Lipid metabolism plays a pivotal role in the management of energy homeostasis. Therefore, inhibition of relevant enzymes may be considered as emphatic targets for the treatment of obesity.[Citation51,Citation52] Triglyceride (TG) is an energy dense fuel, and it is insoluble in the plasma. It has to be transported in the form of large multi-molecular lipoprotein particles. In small intestine, the large TG is hydrolyzed by PL to form small molecules of monoglycerides and free fatty acids. These PL-hydrolyzed TG molecules are then re-esterified in enterocytes and packaged into chylomicron particles, while the endogenous synthesized TG is secreted by the liver to form very low-density lipoprotein (VLDL) particles. Both classes of lipoprotein enter into circulation for the distribution of TG to tissues before the TG-depleted remnant particles are taken up by receptor-mediated processes. During circulation, these VLDL and TG-rich chylomicron particles are then hydrolyzed by the LPL.[Citation53] Overconsumption of high fat diets is mainly responsible for weight gain and obesity due to their high energy and fat content.[Citation54] Lower levels of calorie uptake are needed to prevent the event of obesity. However, positive changes in eating behavior are very difficult to implement. One of the potential solutions to decrease calories intake without compromising eating habits is by inhibition of lipase activity in fat digestion.[Citation55] Therefore, use of herbal extracts with significance enzyme inhibition potential is considered as one of the most acceptable strategy to treat obesity.[Citation56–Citation58] Inhibition of these lipases, both PL and LPL results in few consequences including: 1) inhibition in the digestion of dietary fats, 2) slow down deposition of fat into adipose tissue, 3) reduces solubility and 3) decreases systemic absorption of dietary fat, leading to suppression of weight gain.[Citation56,Citation59] In the present study, out of eight herbs (Cosmos caudatus, Pluchea indica, Lawsonia inermis, Carica papaya, Piper betle, Andrographis paniculata, Pereskia bleo, and Melicope lunu) investigated for their antioxidant and anti-obesity potential, Cosmos caudatus was depicted to exhibit optimal anti-obesity activities. The anti-obesity activities demonstrated by Cosmos caudatus was comparable to that of Momordica. charanthia, Morinda citrifolia, and Centella Asiatica extracts.[Citation60] Cosmos caudatus and Lawsonia inermis also showed higher anti-obesity activities compared to most of the plant extracts tested by Zheng et al.[Citation61]
The antioxidant activities demonstrated by the extracts were considerable, and to some extent, quite potent in inhibiting peroxidation of lipids. The high antioxidant capacity of Cosmos caudatus, Pluchea indica, Lawsonia inermis, Piper betle, and Mammea suriga are also reported in various studies.[Citation62–Citation67] The presence of antioxidative components, which are capable of reducing the extent of ß-carotene destruction by neutralizing the linoleate-free radicals and other free radicals might be responsible for the high antioxidant activity observed. In the present study, multiple procedures were used for the estimation of antioxidant potential of the understudy samples. β-carotene bleaching method is very helpful for investigations of lipophilic antioxidants, while DPPH method is highly useful for both the hydrophilic and lipophilic substances. High antioxidant activity of the extract based on β-carotene bleaching assay ascertained its ability to work at the lipid–water interface.[Citation68] However, variation in the results may be due to the differences in the mechanism of the assays and distinctive phytochemicals involved in each of the plant samples.[Citation67]
The presence of various bioactive metabolites in plant extracts especially polyphenols play an imperative role in their inhibitory effects toward PL activity. Therefore, high phenolic content of Cosmos caudatus, Pluchea indica, and Lawsonia inermis might be related to the high inhibitory activity of PL.[Citation69] The higher levels of TPC in Cosmos caudatus and Pluchea indica are supported by other studies,[Citation35,Citation65,Citation66] whereas the lower phenolic contents of Pereskia bleo and Andrographis paniculata were comparable with the findings of Sim et al.,[Citation70] Qader et al.,[Citation71] and Hassanbaglou et al.[Citation72] The variation in the results for different extracts might be attributed by the presence of different types of phenols and/or presence of other constituents.
Out of all the tested herbs, Cosmos caudatus was depicted to be the most potent herb; therefore, it was selected for further optimization studies. Extracting solvent composition was optimized for Cosmos Caudatus with optimal antioxidant and anti-obesity activities. Hundred (100)% ethanolic extract (at 1000 ppm) showed the highest pancreatic lipase inhibitory effect. At 0.1 mg/mL levels, different extracts of Cosmos caudatus showed higher anti-obesity activity compared to most of the crude plant extracts tested by Roh and Jung.[Citation73] In another study, Gholamhoseinian et al.[Citation74] screened 400 ethanolic plant extracts and revealed that 44 extracts significantly inhibited PL activity ranging from (1.80 ± 0.4%) to (38.0 ± 1.9%). Even at higher concentration of 5 mg/mL, most of these plant extracts showed lower inhibition of PL activities when compared to 100% ethanolic Cosmos caudatus extract at lower concentration of 1 mg/mL. A comparable result was also shown by lyophilized juice (LJ) of Stellaria media (Linn.) Vill. at 1 mg/mL level which PL inhibition activity of about 23%.[Citation75]
LPL and its tissue-specific regulation are of significant importance, aside from PL, that plays an important role in the pathogenesis of obesity.[Citation76] LPL catalyzes hydrolysis of lipoprotein (which includes VLDLs and chylomicrons) releasing free fatty acids for uptake into adipose tissue.[Citation77,Citation78] Therefore, inhibition of LPL may help in preventing deposition of fat into adipose tissue. The ability of plant extracts to inhibit LPL activity has been reported in previous studies. Grape seed extract (GSE) has shown moderate effect on inhibition of LPL in vitro.[Citation79] At concentration of 0.1 mg/mL, GSE showed lower inhibition (2%) when compared to all five different extracts of Cosmos caudatus tested in this study. The inhibitory effects of peanut (Arachis hypogaea) shell extract (PSE) on LPL activity have also been reported by the Moreno et al.[Citation80] At 0.1 mg/mL concentration, the PSE showed comparable result (11%) to that of 60, 50 and 40% ethanolic Cosmos caudatus extracts with LPL inhibition levels 12.9 ± 1.6, 13.2 ± 2.6 and 11.9 ± 1.1%, respectively. Comparatively, at higher concentration (1 mg/mL), the PSE only showed 25% inhibition, which was comparable to that of 100% ethanol Cosmos caudatus extracts (19.9 ± 1.1%) at a lower concentration (0.1 mg/mL). The results of present study were also comparable with the findings of Sahib et al.[Citation60] Morinda citrifolia fruit, Momordica charantia, and Centella asiatica extracts at 0.1 mg/mL concentration levels showed 16.9 ± 2.6, 10.2 ± 1.2, and 18.2 ± 0.2% inhibition, respectively, which was in compliance with the LPL inhibition potential of different ethanolic extracts of Cosmoa caudatus used in present study. Our findings indicated that Cosmos caudatus extract inhibits both PL and LPL activities. Inhibition of these enzymes helps not only in the reduction of fat digestion and absorption (as seen in clinical use of Orlistat), but also slow down deposition of fat into adipose tissue. However, in vivo studies are needed to further address the mechanisms associated with anti-obesity potential of Cosmos caudatus extract.
Flavonoids usually exhibits higher antioxidant potentials as compare to the phenolic acids.[Citation34] The inhibitory activity of different Cosmos caudatus extracts on PL and LPL probably attributed by the phenolic and flavonoid compounds. Results showed that extracts with higher phenolic and flavonoid content (100% > 80% > 60% > 50% > 40% ethanol extracts) exhibited better inhibition of lipases. TPC and TFC were positively correlated with PL and LPL inhibitory activities. Various researchers have also reported the ability of polyphenolic compounds to inhibit PL and LPL activities.[Citation79–Citation81] Cosmos caudatus extracted with 100% ethanol showed exceptionally high antioxidant activity with a nonsignificant difference (p > 0.05) to that of BHA and ascorbic acid. A study by Othman et al.[Citation82] also showed that cocoa beans extracted with ethanol exhibited lower IC50 value compared to cocoa beans extracted with water. On the contrary, 50% ethanol extract of two cultivars of papaya showed highest antioxidant activity compared to that of 70% and 100% ethanol extracts.[Citation83]
Generally, obese subjects are at a risk of high oxidative stress due to the disrupted antioxidant defense systems and strong free radicals production. Obesity is also associated with decrease in antioxidant capacity indicated by low levels of antioxidant enzymes.[Citation84,Citation85] Matsuzawa-nagata et al.[Citation86] in a study reported that the production of ROS and oxidatives stress was equally increased in adipose tissues and liver of mice fed with high-fat diet. Since oxidative stress is related to obesity and leads to other degenerative diseases such as diabetes, hypertension, and hyperlipidemia. Therefore, it might be beneficial to consume multifunctional plants that have both antioxidant and anti-obesity effects. Phenolic compounds play an important role as dietary antioxidants for the prevention of oxidative stress damages.[Citation86] In the present study, antioxidant-rich extracts demonstrated significant anti-obesity effects.
Conclusion
The study revealed that some of the herbs such as Cosmos caudatus, Pluchea indica, and Lawsonia inermis exhibited high free radical scavanging activities, high total phenolic content, and potent pancreatic lipase inhibitory effects. The 100% Cosmos caudatus ethanolic extract was proven to be the most efficient extract enriched with complex mixture of phenolic and flavonoid compounds. Strong positive correlation between bioactive compounds and that of free radical scavenging and anti-lipase activity was observed. The identified phenolic and flavonoid compounds (quercetin-3-rhamnoside, catechin, rutin, 1-caffeyolquinic acid, kaempherol, kaempherol glucoside, quercetin-3-glucoside, quercetin-O-pentoside, and quercetin-rhamnosylgalactoside) may be responsible for the observed antioxidant and anti-obesity activities of Cosmos caudatus extract. This research may provide a basis for in vivo study and strong foundation for future development of standardized herbal medications or active ingredients with great applications in prevention and treatment of obesity.
Acknowledgments
The authors would like to acknowledge the Ministry of Science, Technology and Innovation (MOSTI) of Malaysia for financing the project, Faculty of Food Science and Technology, University Putra Malaysia, for the laboratory facilities and Agricultural Farm, Universiti Putra Malaysia, Serdang, Selangor, Malaysia for kind donation of the plants used in this research.
References
- Mohamed, G.A.; Ibrahim, S.R.M.; khayat, E.S.E.; Dine, R.S.E. Natural Anti-Obesity Agents. Bulletin of Faculty of Pharmacy. Cairo University 2014, 52, 269–284.
- Must, A.; Spadano, J.; Coakley, E.H.; Field, A.E.; Colditz, G.; Dietz, W.H. The Disease Burden Associated with Overweight and Obesity. Journal of American Medical Association 1999, 282, 1523–1529.
- Poulos, S.P.; Dodson, M.V.; Hausman, G.J. Cell Line Models for Differentiation: Preadipocytes and Adipocytes. Experimental Biology and Medicine 2010, 235, 1185–1193.
- Sweeting. A.N.; Hocking, S.L.; Markovic, T.P. Pharmacotherapy for the Treatment of Obesity. Molecular Cell Endocrinology 2015, 418, 173–183.
- Hanefeld, M.; Sachse, G. The Effects of Orlistat on Bodyweight and Glycaemic Control in Overweight Patients with Type 2 Diabetes: A Randomized, Placebo-Controlled Trial. Diabetes, Obesity and Metabolism 2002, 4, 415–423.
- Chakrabarti, R. Pharmacotherapy of Obesity: Emerging Drugs and Targets. Expert Opinion on Therapeutic Targets 2009, 13, 195–207.
- Kusunoki, M.; Tsutsumi, K.; Tana, C.; Sato, D.; Nakamura, T. Lipoprotein Lipase Activation Improves the Cachexia and Obesity. Journal of Obesity and Weight Loss Therapy 2013, 3, Article ID 1000177.
- Gargouri, Y.; Ransac, S.; Verger, R. Covalent Inhibition of Digestive Lipases: An In Vitro Study. Biochimica et Biophysica Acta 1997, 1344, 6–37.
- Newman. D.J.; Cragg, G.M. Natural Products as Sources of New Drugs Over the Last 25 Years. Journal of Natural Products 2007, 70, 461–477.
- Veeru, P.; Kishor, M.P.; Meenakshi, M. Screening of Medicinal Plant Extracts for Antioxidant Activity. Journal of Medicinal Plants Research 2009, 3, 608–612.
- Proestos, C.; Lytoudi, K.; Mavromelanidou, O.K.; Zoumpoulakis, P.; Sinanoglou, V.J. Antioxidant Capacity of Selected Plant Extracts and Their Essential Oils. Antioxidant 2013, 2, 11–22.
- Verma, R.K.; Paraidathathu, T. Herbal Medicines Used in the Traditional Indian Medicinal System as a Therapeutic Treatment Option for Overweight and Obesity Management: A Review. International Journal of Pharmacy and Pharmaceutical Sciences 2013, 6, 40–47.
- Dai, J.; Mumper, R.J. Plant Phenolics: Extraction, Analysis and their Antioxidant and Anticancer Properties. Molecules 2010, 15, 7313–7352.
- Butterfield, D.A. Amyloid b-peptide (1–42)-induced Oxidative Stress and Neurotoxicity: Implications for Neurodegeneration in Alzheimer’s disease Brain. Free Radical Research 2002, 36, 307–1313.
- Lopes, H.F.; Martin, K.L.; Nashar, K.; Morrow, J.D.; Goodfriend, T.L.; Egan, B.M. DASH Diet Lowers Blood Pressure and Lipid-Induced Oxidative Stress in Obesity. Hypertension 2003, 41, 422–430.
- Furukawa, S.; Fujita, T.; Shimabukuro, M.; Iwaki, M.; Yamada, Y.; Nakajima, Y.; Nakayama, O.; Makishima, M.; Matsuda, M.; Shimomura, I. Increased Oxidative Stress in Obesity and its Impact on Metabolic Syndrome. Journal of Clinical Investigations 2004, 114, 1752–1761.
- Halliwell, B. Role of Free Radicals in the Neurodegenerative Diseases: Therapeutic Implications for Antioxidant Treatment. Drugs Aging 2002, 18, 685–716.
- Lee, C.K.; Allison, D.B.; Brand, J.; Weindruch, R.; Prolla, T.A. Transcriptional Profiles Associated with Aging and Middle Age-Onset Caloric Restriction in Mouse Hearts. Proceedings of the National Academy of Sciences of the United States of America 2002, 99, 14988–14993.
- Liu, Q.; Raina, A.K.; Smith, M.A.; Sayre, L.M.; Perry, G. Hydroxynonenal, Toxic Carbonyls, and Alzheimer Disease. Molecular Aspects of Medicine 2003, 24, 305–313.
- Keaney, J.F.Jr.; Larson, M.G.; Vasan, R.S.; Wilson, P.W.F.; Lipinska, I.; Corey, D.; Massaro, J.M.; Sutherland, P.; Vita, J.A.; Benjamin, E.J. Obesity and Systemic Oxidative Stress Clinical Correlates of Oxidative Stress in the Framingham Study. Arteriosclerosis, Thrombosis, and Vascular Biology 2003, 23, 34–439.
- Sfar, S.; Boussoffara, R., Sfar, M.T.; Kerkeni, A. Antioxidant Enzymes Activities in Obese Tunisian Children. Nutrition Journal 2013, 12, doi: 10.1186/1475-2891-12-18.
- Chang, S.S.; Ostric-Matijasevic, B.; Hsieh, O.A.L.; Li Huang, C. Natural Antioxidants from Rosemary and Sage. Journal of Food Science 1977, 42, 1102–1106.
- Fox, P.F.; Stepaniak, L. Isolation and Some Properties of Extracellular Heat-Stable Lipases from Pseudomonas fluorescens Strain AFT 36. Journal of Dairy Research 1983, 50, 77–89.
- Fukumoto, J.; Iwai, W.; Tsujiska, Y. Studies on Lipase Purification and Rystallization of a Lipase Secretion by Aspergillus niger. Journal of General and Applied Microbiology 1963, 9, 353–361.
- Schotz, Μ.C.; Garfinkel, A.S.; Huebotter, R.I.; Steart, J.Ε. A Rapid Assay for Lipoprotein Lipase. Journal of Lipid Research 1970, 11, 68–69.
- Chung, J.; Scanu, A.M. Continuous pH-stat Titration Method for the Assay of Lipoprotein Lipase Activity in vitro. Analytical Biochemistry 1974, 62, 134–148.
- Verza, S.G.; Kreinecker, M.T.; Reis, V.; Henriques, A.T.; Ortega, G.G. Evaluation of Analytical Variables of the Folin-Ciocalteu Method for the Quantitation of the Total Tannins Content Using a psidium guajava l. Leaves Aqueous Extract as a Model. Quimica Nova 2007, 30, 815–820.
- Quettier-Deleu, C.; Gressier, B.; Vasseur, J.; Dine, T.; Brunet, C.; Luyckx, M.; Cazin, M.; Cazin, J.C.; Bailleul, F.; Trotin, F. Phenolic Compounds and Antioxidant Activities of Buckwheat (Fagopyrum esculentum Moench) Hulls and Flour. Journal of Ethnopharmacology 2000, 72, 35–42.
- Brand-Williams, W.; Cuvelier, M.E.; Berset, C. Use of a Free Radical Method to Evaluate Antioxidant Activity. Food Science and Technology 1995, 28, 25–30.
- Velioglu, Y.S.; Mazza, G.; Gao, L.; Oomah, B.D. Antioxidant Activity and Total Phenolics in Selected Fruits, Vegetables and Grain Products. Journal of Agriculture and Food Chemistry 1998, 46, 4113–4117.
- Crozier. A.; Jensen, E.; Lean, M.E.J.; McDonald, M.S. Quantitative Analysis of Flavonoids by Reversed Phase High-Performance Liquid Chromatography. Journal of Chromatography A 1997, 761, 315–321.
- Oktay, M.; Gülçin, I.; Küfrevioglu, Ö.I. Determination of in Vitro Antioxidant Activity of Fennel (Foeniculum vulgare) Seed Extracts. Lebenson Wiss Technol 2013, 36, 263–271.
- Labreche, F.P.; Goldberg, M.S. Exposure to Organic Solvents and Breast Cancer in Women: A Hypothesis. American Journal of Industrial Medicine 1997, 32, 1–14.
- Dian-Nashiela, F.; Noriham, A.; Nooraain, H.; Azizah, A.H. Antioxidant Activity of Herbal Tea Prepared from Cosmos caudatus Leaves at Different Maturity Stages. International Food Research Journal 2015, 22, 1189–1194.
- Mediani, A.; Abas, F.; Ping, T.C.; Khatib, A.; Lajis, N.H. Influence of Growth Stage and Season on the Antioxidant Constituents of Cosmos caudatus. Plant Foods for Human Nutrition 2012, 67, 344–350.
- Perumal, V.; Hamid, A.A.; Ismail, A.; Saari, K.; Abas, F.; Ismail, I.S.; Maulidiani Lajis, N.H.; Khatib, A. Effect of Cosmos caudatus Kunth Leaves On The Lipid Profile Of A Hyperlipidemia-Induced Animal Model. Journal of Food Chemistry and Nutrition 2014, 2, 43–51.
- Shui, G.; Leong, L.P.; Shih, P.W. Rapid Screening and Characterization of Antioxidants of Cosmos Caudatus Using Liquid Chromatography Coupled with Mass Spectrometry. J Chromatography B 2005, 827, 127–138.
- Abas, F.; Shaari, K.; Lajis, N.H.; Israf, D.A.; Umi Kalsom, Y. Antioxidative and Radical Scavenging Properties of the Constituents Isolated from Cosmos caudatus Kunth. Natural Product Sciences 2003, 9, 245–248.
- Sukrasno, S.; Fidriany, I.; Anggadiredja, K.; Handayani, W.A.; Anam, K. Influence of Drying Method on Flavonoid Content of Cosmos caudatus (Kunth) Leaves. Research Journal Medicinal Plant 2011, 5, 189–195.
- Mediani, A.; Abas, F.; Khatib, A.; Ping, T.C. Cosmos Caudatus as a Potential Source of Polyphenolic Compounds: Optimisation of Oven Drying Conditions and Characterisation of Its Functional Properties. Molecules 2013, 18, 10452–10464.
- Mediani, A.; Abas, F.; Khatib, A.; Tan, C.P.; Ismail, I.S.; Shaari, K.; Ismail, A; Lajis, N.H. Phytochemical and Biological Features of Phyllanthus Niruri and Phyllanthus Urinaria Harvested at Different Growth Stages Revealed by 1H NMR-based Metabolomics. Industrial Crops and Products 2015, 77, 602–613.
- Nuengchamnong, N.; Boonpathanasak, S.; Tepwitukkij, P. Rapid Screening of Antioxidant Compounds in Homemade Fruit Fermented Juice Using an On Line LC-ESI-MS/MS and DPPH Assay. Chiang Mai Journal of Science 2011, 38, 430–438.
- Clifford, M.N.; Knight, S.; Kuhnert, N. Discriminating Between the Six Isomers of Dicaffeoylquinic Acid by LC-MS(n). Journal of Agriculture and Food Chemistry 2005, 53, 3821–32.
- Kardel, M.; Taube, F.; Schulz, H.; Schütze, W.; Gierus, M. Different Approaches to Evaluate Tannin Content and Structure of Selected Plant Extracts–Review and New Aspects. Journal of Applied Botany and Food Quality 2013, 86, 154–166.
- Lee, S.Y.; Abasa, F.; Khatib, A.; Ismail, I.S.; Shaari, K.; Zawawi, N. Metabolite Profiling of Neptunia Oleracea and Correlation with Antioxidant and A-Glucosidase Inhibitory Activities Using 1H NMR- Based Metabolomics. Phytochemistry Letters 2016, 16, 23–33.
- Ogita, T.; Vallejo Manaois, R.; Wakagi, M.; Oki, T.; Takano Ishikawa, Y.; Watanabe, J. Identification and Evaluation of Antioxidants in Japanese Parsley. International Journal of Food Science and Nutrition 2016, 67, 431–40.
- Del Rio, D.; Stewart, A.J.; Mullen, W.; Burns, J.; Lean, M.E.; Brighenti, F.; Crozier, A. HPLC-MSn Analysis of Phenolic Compounds and Purine Alkaloids in Green and Black Tea. Journal of Agriculture and Food Chemistry 2004, 52, 2807–2815.
- Jaiswal, R.; Jayasinghe, L.; Kuhnert, N. Identification and Characterization of Proanthocyanidins of 16 Members of the Rhododendron Genus (Ericaceae) by tandem LC-MS. Journal of Mass Spectrometry 2012, 47, 502–15.
- Ado, M.A.; Abas, F.; Ismail, I.S.; Ghazali, H.M.; Shaari, K. Chemical Profile and Antiacetylcholinesterase, Antityrosinase, Antioxidant and α-glucosidase Inhibitory Activity of Cynometra Cauliflora L. Leaves. Journal of the Science of Food and Agriculture 2015, 95, 635–42.
- Maulidiani, A.; Abasa, F.; Khatiba, A.; Shaaria, K.; Lajis, N.H. Chemical Characterization and Antioxidant Activity of Three Medicinalapiaceae Species. Industrial Crops and Products 2014, 55, 238–247.
- 44Loli, H.; Narwal, S.K.; Saun, N.K.; Gupta, R. Lipases in Medicine: An Overview. Mini Reviews in Medicinal Chemistry 2015, 15, 1209–1216.
- 45Sukhdev, S.; Singh, K.S. Therapeutic Role of Phytomedicines on Obesity: Importance of Herbal Pancreatic Lipase Inhibitors. International Research Journal of Medical Sciences 2013, 1, 15–26.
- Havel, R. McCollum Award Lecture, 1993: Triglyceride-rich Lipoproteins and Atherosclerosis–New Perspectives. American Journal of Clinical Nutrition 1994, 59, 795–796.
- Bray, G.A.; Popkin, B.M. Dietary Fat Intake does Affect Obesity! American Journal of Clinical Nutrition 1998, 68, 1157–1173.
- De la Garza, A.L.; Milagro, F.I.; Boque, N.; Campion, J.; Martinez, J.A. (2011). Natural Inhibitors of Pancreatic Lipase as New Players in Obesity Treatment. Planta Medica 2011, 77, 773–785.
- Dechakhamphu A, Wongchum N. Screening for Anti-Pancreatic Lipase Properties of 28 Traditional Thai Medicinal Herbs. Asian Pacific Journal of Tropical Biomedicine 2015, 5, 1042–1045.
- Yun, J.W. Possible Anti-Obesity Therapeutics from Nature-A Review. Phytochemistry 2010, 71, 1625–1641.
- Martin, K.A.; Mani, M.V.; Mani, A. New Targets to Treat Obesity and the Metabolic Syndrome. European Journal of Pharmacology 2015, 15, 64–74.
- Embleton, J.K.; Pouton, C.W. Structure and Function of Gastrointestinal Lipases. Advanced Drug Delivery Reviews 1997, 25, 15–32.
- Sahib, N.G.; Hamid, A.A.; Saari, N.; Abas, F.; Pak Dek, M.S.; Rahim, M. Anti-Pancreatic Lipase and Antioxidant Activity of Selected Tropical Herbs. International Journal of Food Properties 2012, 15, 569–578.
- Zheng, C.D.; Duan, Y.Q.; Gao, J.M.; Ruan, Z.G. 2010. Screening for Anti-Lipase Properties of 37 Traditional Chinese Medicinal Herbs. Journal of Chinese Medical Association 2010, 73, 319–324.
- Abas, F.; Nordin, H.L.; Israf, D.A.; Khozirah, S.; Umi Kalsom, Y. 2006. Antioxidant and Nitric Oxide Inhibition Activities of Selected Malay Traditional Vegetables. Food Chemistry 2006, 95, 566–573.
- Mustafa, R.A.; Hamid, A.A.; Mohamed, S.; Bakar, F.A. Total Phenolic Compounds, Flavonoids, and Radical Scavenging Activity of 21 Selected Tropical Plants. Journal of Food Science 2010, 75, 28–35.
- Noridayu, A.R.; Hii, Y.F.; Abas, F.; Shaari, K.; Lajis, N. Antioxidant and Antiacetylcholinesterase Activities of Puchea indica Less. International Food Research Journal 2011, 18, 925–929.
- Mohd Nazri, N.A.; Ahmat, N.; Adnan, A.; Syed Mohamad, S.A.; Syaripah Ruzaina, S.A. In-vitro Antibacterial and Radical Scavenging Activities of Malaysian Table Salad. African Journal of Biotechnology 2011, 10, 5728–5735.
- Mediani, A.; Abas, F.; Tan, C.P.; Khatib, A. Effects of Different Drying Methods and Storage Time on Free Radical Scavenging Activity and Total Phenolic Content of Cosmos caudatus. Antioxidants 2014, 3, 358–370.
- Poojary, M.M.; Vishnumurthy, K.A.; Adhikarin, A.V. Extraction, Characterization and Biological Studies of Phytochemicals from Mammea suriga. Journal of Pharmaceutical Analysis 2015, 5, 182–189.
- Bouhdid, S.; Skali, S.N.; Idaomar, M.; Zhiri, A.; Baudoux, D.; Amensour, M.; Abrini, J. Antibacterial and Antioxidant Activities of Origanum Compactum Essential Oil. African Journal of Biotechnology 2008, 7, 563–1570.
- Lei, F.; Zhang, X.N.; Wang, W.; Xing, D.M.; Xie, W.D.; Su, H.; Du, L.; J. Evidence of Anti-Obesity Effects of the Pomegranate Leaf Extract in High-Fat Diet Induced Obese Mice. International Journal of Obesity 2007, 31, 1023–1029.
- Sim, K.S.; Sri Nurestri, A.M.; Norhanom, A.W. Phenolic Content and Antioxidant Activity of Crude and Fractionated Extracts of Pereskia bleo (Kunth) DC. (Cactaceae). African Journal of Pharmacy and Pharmacology 2010, 4, 193–201.
- Qader, S.W.; Abdulla, M.A.; Chua, L.S.; Najim, N.; Zain, M.M.; Hamdan, S. Antioxidant, Total Phenolic Content and Cytotoxicity Evaluation of Selected Malaysian Plants. Molecules 2011, 16, 3433–3443.
- Hassanbaglou, B.; Hamid, A.A.; Roheeyati, A.M.; Mohd Saleh, N.; Abdulamir, A.S.; Khatib, A.; Sabu, M. Antioxidant Activity of Different Extracts from Leaves of Pereskia bleo (Cactaceae). Journal of Medicinal Plants Research 2012, 6, 2932–2937.
- Roh, C.; Jung, Y. Screening of Crude Plant Extracts with Anti-Obesity Activity. International Journal of Molecular Science 2012, 13, 1710–1719.
- Gholamhoseinian, A.; Shahouzehi, B.; Sharififar, F. Inhibitory Effect of Some Plant Extracts on Pancreatic Lipase. International Journal of Pharmacology 2010, 6, 18–24.
- Rani, N.; Vasudeva, N.; Sharma, S.K. Quality Assessment and Anti-Obesity Activity of Stellaria media (Linn.) Vill. BMC Complementary and Alternative Medicine 2012, 12, 145, doi:10.1186/1472-6882-12-145.
- Zechner, R.; Strauss, J.; Frank, S.; Wagner, E.; Hofmann, W.; Kratky, D. The Role of Lipoprotein Lipase in Adipose Tissue Development and Metabolism. International Journal of Obesity Related Metabolic Disorders 2000, 24, 53–56.
- Goldberg, I.J.; Merkel, M. Lipoprotein Lipase: Physiology, Biochemistry, and Molecular Biology. Frontiers in Bioscience 2001, 6, 388–405.
- Wong, H.; Schotz, M.C. The Lipase Gene Family. Journal of Lipid Research 2002, 43, 993–999.
- Moreno, D.A.; Ilic, N.; Poulev, A.; Brasaemle, D.L.; Fried, S.K.; Raskin, I. Inhibitory Effects of Grape Seed Extract on Lipases. Nutrition 2003, 19, 876–879.
- Moreno, D.A.; Ilic, N.; Poulev, A.; Raskin, I. Effects of Arachis hypogaea nutshell Extract on Lipid Metabolic Enzymes and Obesity Parameters. Life Sciences 2006, 78, 2797–2803.
- Shimura, S.; Itoh, Y.; Yamashita, A.; Kitano, A.; Hatano, T.; Yoshida, T.; Okuda, T. Inhibitory Effects of Flavonoids on Lipase. Nippon Shokuhin Kogyo Gakkaishi 1994, 41, 847–850.
- Othman, A.; Ismail, A.; Abdul Ghani, N.; Adenan, I. Antioxidant Capacity and Phenolic Content of Cocoa Beans. Food Chemistry 2007, 100, 1523–1530.
- Addai, Z.R.; Abdullah, A.; Abd Mutalib, S. Effect of the Extraction Solvents on the Phenolic Content and Antioxidant Properties of Two Papaya Cultivars. Journal of Medicinal Plants Research 2013, 7, 3354–3359.
- Asayama, K.; Nakane, T.; Dobashi, K.; Kodera, K.; Hayashibe, H.; Nakazawa, S. Effect of Obesity and Troglitazone on Expression of Two Glutathione Peroxidases: Cellular and Extracellular Types in Serum, Kidney and Adipose Tissue. Free Radical Research 2001, 34, 337–347.
- Carmiel-Haggai, M.; Cederbaum, A.I.; Nieto, N. A High-Fat Diet Leads to the Progression of Non-Alcoholic Fatty Liver Disease in Obese Rats. FASEB Journal 2005, 19, 136–138.
- Matsuzawa-Nagata, N.; Takamura, T.; Ando, H.; Nakamura, S.; Kurita, S.; Misu, H.; Ota, T.; Yokoyama, M.; Honda, M.; Miyamoto, K.; Kaneko, S. Increased Oxidative Stress Precedes the Onset of High-Fat Diet-Induced Insulin Resistance and Obesity. Metabolism 2008, 57, 1071–1077.