ABSTRACT
Peroxidases have wide applications in different areas including chemical synthesis, medicine, food industry, bioremediation of wastewater, biosensing, and biotechnology. Amorphophallus paeoniifolius (elephant foot yam) is an attractive source of enzymes; however, no effort has been made to assess peroxidase enzyme from this source. Therefore, the objectives of this study were to evaluate and compare peroxidases from corms of elephant foot yam and roots of Dacus carota (carrot) and Aramoracia rusticana (horseradish). Elephant foot yam corm peroxidase (ECP) demonstrated 4.5 times higher specific activity compared with carrot root peroxidase (CRP). ECP showed retention of high activity over a broad pH range and had higher temperature optima and thermal stability compared with CRP and horseradish peroxidase (HRP). The calculated KM values of ECP, CRP, and HRP for the substrates guaiacol and hydrogen peroxide were 4.5 mM and 307.8 µM, 4.6 mM and 55.5 µM, and 3.5 mM and 413.0 µM, respectively. Peroxidases are used for the bioremediation of wastewater contaminated with hazardous aromatic compounds. Heavy metals such as cadmium (Cd2+), lead (Pb2+), and toxic compounds such as sodium azide (NaN3) are often present in wastewater along with aromatic compounds. Results showed activation of ECP and inhibition of HRP at 250 and 500 µM concentrations of Cd2+ and Pb2+. Treatment with 1 mM NaN3 led to 8.4%, 55.5%, and 11.0% inhibition in the activities of ECP, CRP, and HRP, respectively. Overall, our results suggest that elephant foot yam can be used as a rich and convenient source of peroxidase for various applications including wastewater remediation.
Introduction
Peroxidases (E.C.I.II.I.7) are present ubiquitously in the plant kingdom. They have the ability to catalyse the oxidation of a wide variety of phenolic compounds and therefore have broad applications in many areas including chemical synthesis, diagnostic, medicine, pulp, and paper industries, food industry, and bioremediation of wastewater.[Citation1,Citation2] Peroxidases are used in the treatment of wastewater contaminated with aromatic compounds including phenols, cresols, and chlorinated phenols due to various sources, including petroleum refining, wood preservation, metal coating, dyes and other chemicals, textiles, mining, and dressing [Citation3,Citation4]. For the commercial production of peroxidase, horseradish (Aramoracia rusticana) roots are used as a traditional source. Commercially available horseradish peroxidase (HRP) accessibility and cost restrict its application. Therefore, stable, active, and less-expensive peroxidases from sources other than horseradish are required.
Many studies have been carried out in the search for an alternative source of peroxidase, which has higher availability, activity, and stability. These include white cabbage,[Citation5] sweet gourd,[Citation6] oil palm leaf,[Citation7] sweet potato tubers,[Citation8] turnip,[Citation9,Citation10] melon,[Citation11] cabbage,[Citation12] Brussels sprout,[Citation13] okra,[Citation14], oranges,[Citation15] tea leaves,[Citation16] pepper fruits,[Citation17] carrot roots,[Citation18] tobacco,[Citation19] tomato,[Citation20] green asparagus,[Citation21] strawberry,[Citation22] etc. Spring cabbage peroxidase was reported to be a useful tool in biocatalysis and bioelectrocatalysis.[Citation23] Microbes are the potential source of peroxidase enzyme and a number of such reports are available in the literature, but the production in a stirred tank fermenter is not suitable as these microbes are known to be susceptible to shear stress.[Citation24] Research is still going on to find novel peroxidases of high stability and properties suitable for various biotechnological, biomedical, and other applications.
Short growing period, drought resistance, large corm size, and storability for longer period after harvesting make elephant foot yam an attractive candidate source of peroxidase. Moreover, it is less expensive and easily grown in tropical and subtropical countries. To the best of our knowledge, there is no report available regarding the assessment and characterization of peroxidase from elephant foot yam. Therefore, this study was carried out to evaluate and compare elephant foot yam corm peroxidase (ECP), carrot peroxidase (CRP), and HRP. Enzymatic properties such as kinetic parameters (KM and Vmax) and the effects of pH, temperature, and possible inhibitors (Cd2+, Pb2+, and NaN3) on the activities of ECP, CP, and HRP were studied. The main purpose of this study was to find out if elephant foot yam could be used as a convenient and rich source of peroxidase.
Materials and methods
Elephant foot yam corm and carrot roots were purchased from the local market. HRP was obtained from Sigma. All other chemicals and solvents used were of analytical grade (Hi-media or Sigma).
Enzyme extraction
For the preparation of crude enzyme extract, fresh samples (5 g) were chopped into small pieces and homogenized in 5 ml of 50 mM potassium phosphate buffer (pH 7.0) containing 1 mM phenylmethylsulfonyl fluoride (PMSF) and 1% polyvinylpyrrolidone (PVP) under chilled condition. Homogenates were centrifuged at 13,500 rpm for 20 min at 4°C and the supernatant was collected.
Peroxidase activity assay
The assay mixture consisted of a total volume of 3 ml 50 mM potassium phosphate buffer (pH 7.0) containing 2 mM H2O2 and 5 mM guaiacol as substrates.[Citation25] The crude enzyme was added just before the measurement. The changes in absorbance were recorded every 30 s for 2 min in a spectrophotometer at 470 nm for tetraguaiacol formation by enzymatic reaction, using a Thermo Scientific 10S UV-Vis spectrophotometer. Enzyme activity was expressed as μmol or nmol H2O2 reduced min−1 mg−1 protein.
Protein determination
Total soluble protein was quantified according to the Bradford method[Citation26] using bovine serum albumin as standard.
Effect of pH and temperature on enzyme activity
The pH optimum was determined by measuring the activity of the enzyme in the pH range 3.0–8.0 using buffer prepared with H3PO4/KH2PO4/K2HPO4. The temperature optimum was determined by measuring the activity of the peroxidase in the temperature range 30°C–90°C at fixed pH 7.0. At each temperature, the enzyme was incubated in a water bath for 15, 30, 45, and 60 min and the activity was taken to measure its stability at a particular temperature.
Steady-state enzyme kinetics
The steady-state kinetics of the enzyme was studied for ECP, CRP, and HRP using guaiacol and H2O2 as the variable substrates. The values of Km and Vmax for guaiacol were determined by varying the concentration of guaiacol (0.5, 1, 2, 3, 4, 5, 6 mM) and keeping the concentration of H2O2 constant at enzyme saturating value 2 mM. The values of Km and Vmax for H2O2 were determined by varying the concentrations of H2O2 (0.05, 0.1, 0.15, 0.2, 0.3, 0.4, 0.5, 1, 2, 3 mM) and keeping the concentration of guaiacol constant at the enzyme saturating value 5 mM. The Km and Vmax values were calculated in both cases (guaiacol and H2O2) using linear regression analyses of the data points of double-reciprocal plots.
Effect of Cd2+, Pb2+, and NaN3 on peroxidase activity
The constituents of the assay mixture were the same as earlier and in addition contained increasing concentrations of CdCl2 (0, 250, and 500 μM), Pb(NO3)2 (0, 250, and 500 μM), and NaN3 (0, 1, 5, and 10 mM). The mixture of enzyme and inhibitor was incubated at room temperature for 10 min and the enzyme activity was assayed.
Results and discussion
HRP is used extensively in industries; however, its availability and high cost confine its application. Therefore, alternative peroxidases with commercially viable properties such as low cost, high stability, and activity are required. Elephant foot yam is an attractive candidate source of enzymes; however, no effort has been made to assess peroxidase from it. In this study, we evaluated and compared peroxidases from the corm of elephant foot yam and roots of carrot and horseradish. In our study, ECP (289.7 nmol min−1 mg−1 protein) showed 4.5 times higher specific activity than CRP (64.8 nmol min−1 mg−1protein) at neutral pH.
One of the determining factors in the expression of enzymatic activity is pH, which alters the ionization states of amino acid side chains or substrate. Therefore, enzymes with high activity over a broad pH range are desirable for industrial applications. The effect of pH on the activities of ECP, CRP, and HRP are displayed in –). Both, HRP and ECP had pH optima around 6.0 and retained high activities over a broad pH range ( and ). In contrast, CRP had a sharp peak in its pH-activity curve at pH 6.5 (Fig. 1c). Similar to ECP and HRP, peroxidase from Brassica olerace[Citation27] also showed pH optima at 6.0 and retained high activity over a broad pH range. Peroxidases from Brassica vulgaris and Citrus medica, however, showed a sharp peak at 6.0 in their pH-activity curve.[Citation28,Citation29] Retention of high activity over a broad pH range indicates that ECP can be used effectively for various industrial applications.
Figure 1. Effect of pH (A, B, C) and temperature (D, E, F) on the activities of peroxidases obtained from elephant foot yam, carrot, and horseradish. Values are mean ± SD based on three independent determinations and bars indicate standard deviations.
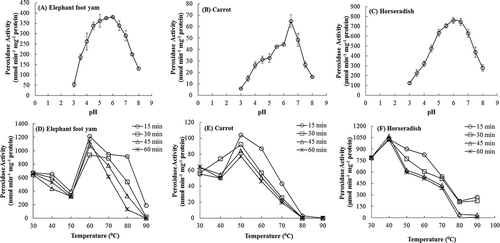
Peroxidase is one of the most thermostable enzymes in plants. The temperature–activity profiles of ECP, CP, and HRP are depicted in –). ECP, CP, and HRP showed the highest activities at 60°C, 50°C, and 40°C, respectively. The temperature optima reported for the peroxidases from B. vulgaris,[Citation28] B. olerace,[Citation27] and C. medica[Citation29] are 60°C, 35°C–40°C, and 50°C, respectively. The ECP was more thermostable than HRP and CRP and retained a significantly higher fraction of catalytic activity even at 70°C. Thermostable enzymes offer major advantages due to their ability to withstand elevated temperatures during industrial processing and possibilities for prolonged storage at room temperature.
All three peroxidases followed the Michaelis–Menton kinetics and showed first-order reaction kinetics for substrates H2O2 and guaiacol (–)). The KM and Vmax values were calculated for these peroxidases using the Lineweaver–Burk plot. Double-reciprocal plots for ECP, CRP, and HRP with guaiacol as the variable substrate are shown in –). KM indicates affinity between the enzyme and the substrate and is therefore an important kinetic parameter for industrial applications. The calculated KM values of ECP, CRP, and HRP for guaiacol and hydrogen peroxide were 4.5 mM and 307.8 µM, 4.6 mM and 55.5 µM, and 3.5 mM and 413.0 µM, respectively. The reported KM values with respect to guaiacol and hydrogen peroxide for peroxidase extracted from citron (8 mM and 1800 µM), sweet corn (11 mM and 2850 µM), waxy corn (23 mM and 330 µM), garlic bulb (9.5 mM and 2000 µM), and eggplant (6.5 mM and 330 μM) were higher than ECP.[Citation29–Citation32] The Vmax values of ECP for guaiacol and hydrogen peroxide (24 and 10 nmol min−1 mg−1 protein, respectively) were around 4.5 times higher than CRP (5.2 and 2.7 nmol min−1 mg−1 protein, respectively).
Figure 2. Double-reciprocal plots for the peroxidases obtained from elephant foot yam, carrot, and horseradish using guaiacol (A, B, C) and hydrogen peroxide (D, E, F) as variable substrates. Values are the mean of three independent determinations.
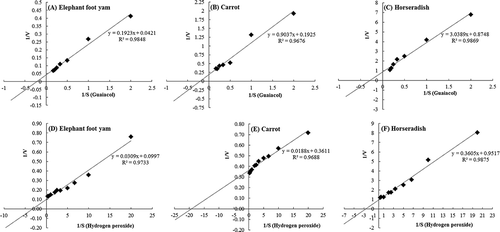
Peroxidases have application in the bioremediation of wastewater contaminated with hazardous aromatic compounds. Heavy metals such as Cd2+, Pb2+ and toxic compounds such as NaN3, generally present in wastewater along with aromatic compounds have the capability to inhibit peroxidases. Therefore, the effects of increasing concentrations of Cd2+ and Pb2+ and NaN3 on the activities of ECP, CRP, and HRP were studied. Cd2+ treatments of 250 and 500 µM led to enhanced ECP and CRP activities but decreased HRP activity (–). Pb2+ treatment (250 and 500 µM) also led to increased ECP activity but decreased CP and HRP activities (–). Activation as well as inhibition of peroxidases due to transition metals has been reported previously.[Citation33–Citation35] Citurs reticulate peroxidase activity was slightly increased after 1 h of incubation with 2 mM Cd2+, but decreased with 2 mM Pb2+.[Citation36] Coordination of metal ions to active-site residues can lead to the activation of enzymes; however, such coordination may block substrate interaction, causing inhibition.[Citation37] In general, metal ions at low concentrations activate enzyme whereas at higher concentrations, they inhibit enzyme activity. Our results suggested that the concentrations of metal required to activate or inhibit peroxidases depend on both the type of metal and the source of peroxidase. ECP activity showed 89.6% and 26.3% increase after 10 min incubation with 500 µM Cd2+ and Pb2+, respectively. Mahmoudi et al.[Citation38] showed that incubation of the enzyme with the metal ion at activating concentration leads to the long-term functional stability of peroxidase. The effects of increasing concentrations of NaN3 on ECP, CP, and HRP are shown in –). NaN3 concentrations of 1 mM led to 8.4%, 55.5%, and 11% inhibition in the activities of ECP, CRP, and HRP, respectively. Peroxidase from jatropha leaves showed 36.9%, 95.7%, and 98.8% inhibition after 2 h incubation with 1, 5, and 10 mM NaN3, respectively.[Citation39] The decrease in the relative peroxidase activity of 26.6% and 40.0% was reported after incubation with 2 mM NaN3 for 1 h in C. medica and B. vulgaris, respectively. These results suggested that ECP could be efficiently used in the remediation of wastewater contaminated with aromatic compounds along with Cd2+, Pb2+, and NaN3.
Conclusion
Elephant foot yam is relatively a less-expensive vegetable that is widely grown in tropical as well subtropical countries. ECP showed high activity over a broad pH range and was more thermally stable than CRP and HRP. It showed high affinity for guaiacol as well as hydrogen peroxide. The investigation also demonstrated the activation of ECP and the inhibition of HRP by 250 and 500 μM concentrations of Cd2+ and Pb2+, respectively. Overall, our results suggested that elephant foot yam corm can serve as an alternative rich and convenient source of peroxidase for wide applications including wastewater remediation.
Acknowledgements
The authors are thankful to DBT Builder project No. BT/PR-9028/INF/22/193/2013 for their financial support. PS is thankful to UGC-FRP.
References
- Agostini, E.; Hernandez-Ruiz, J.; Arnao, M.B.; Milrand, S.R.; Tigier H.A.; Acosta, M. A Peroxidase Isoenzyme Secreted by Turnip (Brassica napus) Hairy-Root Culture Inactivation by Hydrogen Peroxide and Application in Diagnostic Kits. Biotechnology and Applied Biochemistry 2002, 35, 1–7.
- Moreira, P.R.; Almeida-Vara, E.; Malcata, F.X.; Duarte, J.C. Lignin Transformation by a Versatile Peroxidase from a Novel Bjerkandera Sp. Strain. International Biodeterioration and Biodegradation 2007, 59, 234–238.
- Chiong, T.; Lau, S.Y.; Khor, E.H.; Danquah, M.K. Enzymatic Approach to Phenol Removal from Wastewater using Peroxidases. OA Biotechnology 2014, 10, 3–9.
- Bansal, N.; Kanwar, S.S. Peroxidase(s) in Environment Protection. The Scientific World Journal 2013, Article ID 714639, 9 pages.
- Erdem, H.U.; Kalın R.; Özdemir, N.; Özdemir H. Purification and Biochemical Characterization of Peroxidase Isolated from White Cabbage (Brassica Oleracea var. capitata f. alba). International Journal of Food Properties 2015, 18, 2099–2109.
- Koksal, E.; Bursal, E.; Aggul, A.G.; Gulcin, I. Purification and Characterization of Peroxidase from Sweet Gourd (Cucurbita moschata Lam. Poiret). International Journal of Food Properties 2012, 15, 1110–1119.
- Deepa, S.S.; Arumughan, C. Purification and Characterization of Soluble Peroxidase from Oil Palm (Elaeis guineensis Jacq.) Leaf. Phytochemistry 2002, 61, 503–511.
- Leon, J.C.; Alpeeva, I.S.; Chubar, T.A.; Galaev, I.Y.; Csoregi, E.I.; Sakharov, I.Y. Purification and Substrate Specificity of Peroxidase from Sweet Potato Tubers. Plant Science 2002, 163, 1011–1019.
- Hamed, R.R.; Maharem, T.M.; Abdel Fatah, M.M.; Ataya, F.S. Purification of Peroxidase Isoenzymes from Turnip Roots. Phytochemistry 1998, 48, 1291–1294.
- Duarte-Va´zquez, M.A.; García-Almenda´rez, B.E.; Regalado, C.; Whitaker, J.R. Purification and properties of a neutral peroxidase isozyme from turnip (Brassica napus L. var. purple top white globe) roots. Journal of Agricultural and Food Chemistry 2001, 49, 4450–4456.
- Rodriguez Lopez, J.N.; Espin, J.C.; Amor, F.del Tudela, J.; Martinez, V.; Cerda, A.; Garcia-Canovas, F. Purification and Kinetic Characterization of an Anionic Peroxidase from Melon (Cucumis melo L.) Cultivated Under Different Salinity Conditions. Journal of Agricultural and Food Chemistry 2000, 48, 1537–1541.
- Regalado, C.; Perez Arvizu, O.; Garcia Almendarez, B.E.; Whitaker, J.R. Purification and Properties of Two Acid Peroxidases from Brussels Sprouts (Brassica oleraceae L.). Journal of Food Chemistry 1999, 23, 435–450.
- McLellan, K.M.; Robinson, D.S. Purification and Heat Stability of Brussels Sprout Peroxidase Isoenzymes. Food Chemistry 1987, 23, 305–319.
- Yemenicioglu, A.; Ozkan, M.; Cemeroglu, B. Partial Purification and Thermal Characterization of Peroxidase from Okra (Hibiscus esculentum). Journal of Agricultural and Food Chemistry 1998, 46, 4158–4163.
- Clemente, E. Purification and Thermostability of Isoperoxidase from Oranges. Phytochemistry 1998, 49, 29–36.
- Kvaratskhelia, M.; Winkel, C.; Thorneley, R.N.F. Purification and Characterization of a Novel Class III Peroxidase Isoenzyme from Tea Leaves. Plant Physiology 1997, 114, 1237–1245.
- Pomar, F.; Bernal, M.A.; Diaz, J.; Merino, F. Purification, Characterization and Kinetic Properties of Pepper Fruit Acidic Peroxidase. Phytochemistry 1997, 46, 1313–1317.
- Nair, A.R.; Showalter, A.M. Purification and Characterization of a Wound-Inducible Cell Wall Cationic Peroxidase from Carrot Roots. Biochemical and Biophysical Research Communications 1996, 226, 254–260.
- Gazaryan, I.G.; Lagrimini, L.M. Purification and Unusual Kinetic Properties of a Tobacco Anionic Peroxidase. Phytochemistry 1996, 41, 1029–1034.
- Jen, J.J.; Seo, A.; Flurkey, W.H. Tomato Peroxidases Purification via Hydrophobic Chromatography. Journal of Food Science 1980, 45, 60–63.
- Wang, Z.; Luh, B.S. Characterization of Soluble and Bound Peroxidases in Green Asparagus. Journal of Food Science 1983, 48, 1412–1417.
- Civello, P.M.; Martinez, G.A.; Chaves, A.R.; Anon, M.C. Peroxidase from Strawberry Fruit (Fragaria ananassa-Duch)s Partial-Purification and Determination of Some Properties. Journal of Agricultural and Food Chemistry 1995, 43, 2596–2601.
- Belcarz, A.; Ginalska, G.; Kowalewska, B., Kulesza, P. Spring Cabbage Peroxidases Potential Tool in Biocatalysis and Bioelectro-Catalysis. Phytochemistry 2008, 69, 627–636.
- Mtui, G.; Nakamura, Y. Lignocellulosic Enzymes from Flavodon Flavus, a Fungus Isolated from Western Indian Ocean off the Coast of Dar es Salaam, Tanzania. African Journal of Biotechnology 2008, 7, 3066–3072.
- Egley, G.H.; Paul, R.N. Jr.; Vaughn, K.C; Duke, S.O. Role of Peroxidase in the Development of Water-Impermeable Seeds Coats in Sida spinosa L. Planta 1983, 157, 224–232.
- Bradford, M.M. A Rapid and Sensitive Method for Quantitation of Microgram Quantities of Protein Utilizing the Principle of Protein Dyebinding. Analytical Biochemistry 1976, 72, 248–254.
- Shetty, P.; Banu, U.; Kedilaya, T.; Patheja, M. Characterization of Peroxidase from Brassica oleracea Gongylodes Gives a Lead for Use of Bromocresol Purple as a Novel Substrate for Peroxidase Assay. Journal of Biochemical Technique 2012, 4(1), 502–507.
- Chaurasia, P.K.; Bharati, S.L.; Singh, S.K.; Yadav, R.S.S. Potential Peroxidase Obtained from the Juice of Beta Vulgaris (Beet). American Journal of Food Science and Technology 2013, 1, 30–35.
- Mall, R.; Naik, G.; Mina, U.; Mishra, S.K. Purification and Characterization of a Thermostable Soluble Peroxidase from Citrus Medica Leaf. Preparative Biochemistry & Biotechnology 2013, 43, 137–151.
- Liu, F.; Niu, L.; Li, D.; Liu, C.; Jin, B. Kinetic Characterization and Thermal Inactivation of Peroxidase in Aqueous Extracts from Sweet Corn and Waxy Corn. Food and Bioprocess Technology 2012, 6, 2800–2807.
- Marzouki, S.M.; Limam, F.; Smaali, M.I.; Ulber, R.; Marzouki, M.N. A New Thermostable Peroxidase from Garlic Allium sativum. Applied Biochemistry and Biotechnology 2005, 127, 201–214
- Vernwal, S.K.; Yadav, R.S.S.; Yadav, K.D. Purification of a Peroxidase from Solanum melongena Fruit Juice. Indian Journal of Biochemistry and Biophysics 2006, 43, 239–243.
- Haschke, R.H.; Friedhoff, J.M. Calcium-Related Properties of Horseradish Peroxidase. Biochemical and Biophysical Research Communications 1978, 80, 1039–1042.
- Converso, D.A.; Fernandez, M.E.; Tomaro, M.L. Cadmium Inhibition of a Structural Wheat Peroxidase. Journal of Enzyme Inhibition 2000, 15, 171–183.
- Youngs, H.L., Sundaramoorthy, M.; Gold, H. Effects of Cadmium on Manganese Peroxidase. European Journal of Biochemistry 2000, 267, 1761–1769.
- Nouren, S.; Bhatti, H.N.; Bhatti, I.A.; Asgher, M. Kinetic and Thermal Characterization of Peroxidase from Peels of Citrus reticulata var. Kinnow. The Journal of Animal & Plant Sciences 2013, 23, 430–435.
- Shi, X.; Dalal, S.; Kasprzak, K.S. Generation of Free Radicals from Lipid Hydroperoxides by Ni2+ in the Presence of Oligopeptides. Archives of Biochemistry and Biophysics, 1992, 299, 154–162.
- Mahmoudi, A.; Nazari, K.; Mohammadian, N.; Moosavi-Movahedi, A.A. Effect of Mn2+, Co2+, Ni2+, and Cu2+ on Horseradish Peroxidase. Applied Biochemistry and Biotechnology, 2003, 104, 81–94.
- Cai, F.; OuYang, C.; Duan, P.; Gao, S.; Xu, Y.; Chen, F. Purification and Characterization of a Novel Thermal Stable Peroxidase from Jatropha Curcas Leaves. Journal of Molecular Catalysis B: Enzymatic, 2012, 77, 59–66.