Abstract
Low glycaemic index (GI) rice flour was prepared by the enzymatic hydrolysis method. The protein content of treated (high-protein, low GI) rice flour was about 3.5 times higher than untreated rice flour, suggesting its use in infant formula or other protein-fortified food products. The GI of untreated and treated rice flour was found to be 88.0 ± 0.2 and 46.1 ± 0.2, respectively. High-protein, low-GI rice flour was studied for thermal and structural properties, which showed significant (p < 0.05) differences from untreated rice flour. The results of thermal properties showed a decrease in transition temperatures and enthalpy of gelatinization. The ratio of peaks at 1045 cm−1 and 1015 cm−1 of treated rice flour was significantly (p<0.05) lower than that of untreated rice flour. This could be ascribed to the loss of crystallinity due to enzymatic degradation. These results further support the decrease in transition temperatures and enthalpy of gelatinization. The results of the rheological studies of high-protein, low-GI rice flour revealed that both untreated and treated rice flour samples exhibited non-Newtonian shear-thinning flow behaviour (n < 1), which is characterized by a decrease in viscosity with an increase in shear rate.
Introduction
Rice (Oryza sativa L.) is a principal cereal[Citation1] and is the staple food for more than half of the world’s population.[Citation2] Rice is the second most consumed cereal grain after wheat.[Citation3] The predominant type of rice consumed worldwide is white rice, which can also come in the form of starch and rice flour. Rice is very nutritious and is an excellent source of carbohydrates, low in fat and salt, cholesterol-free, a good source of vitamins and minerals such as niacin, thiamine, iron, riboflavin, vitamin D, calcium, gluten-free, and non-allergenic.[Citation4] Due to being nutritious, consumption of white rice is still limited because it has a high glycaemic index (GI) compared with other starches. Hu et al.[Citation5] have reported the GI of rice from 54 to 121. Worldwide, rice flours and starches are important ingredients used in both traditional and novel foods.[Citation1,Citation6] Absence of gluten provides an additional advantage, making rice a suitable alternative to wheat flour in bakery products, particularly to those suffering from celiac disease.[Citation1,Citation7] The GI was proposed to classify the carbohydrate-based foods according to their glucose response after consumption for 2 h and is presented as a value relative to that of glucose or white bread.[Citation8] High consumption of white rice has been found to be associated with a significantly increased risk of type-2 diabetes, especially in Asians.[Citation9] McGonigal & Kapustin.[Citation10] have reported in a study that the risk of type-2 diabetes was increased by 59% in women and 37% in men, if the panellists usually consumed a food with GI in a range of 79–82.
In addition, the starch content of rice is the major determinant of its GI. Starch is the most abundant storage carbohydrate in cereals, roots, and tubers[Citation11,Citation12] and plays a special role in glucose homeostasis.[Citation13] Consumption of easily digestible food results in a rapid rise in blood glucose and substantial fluctuation of hormones, which places high stress on the regulatory system.[Citation14,Citation15] Physical, chemical, and enzymatic methods, however, may be used to modify the molecular structure of starch,[Citation16] thereby delaying its rate of digestion. Among these, enzymatic methods are of special interest as they are safer both to the consumer and to the environment and the reaction can be more specifically controlled under mild conditions and result in fewer by-products.[Citation17]
Rice constitutes about 6–10% protein, which has a relatively high protein efficiency ratio (PER) of 2.18, almost equivalent to that of beef (2.30), a considerably more costly protein source.[Citation18] Efforts have, therefore, been made to improve the protein level in rice involving the selective breeding of new rice varieties, but it did not improve the protein content to the extent that it could be used in rice-based infant formula or other protein-enriched/fortified foods. Another approach has been to increase the protein content of rice flour by gelatinizing and enzymatically digesting rice starch with carbohydrates commonly known as amylases. This treatment hydrolyses the starch to soluble saccharides of various molecular weights such as glucose, maltose, oligosaccharides, and dextrins from which the insoluble protein-enriched rice flour is separated, for example by centrifugation.[Citation18] Thus, by the partial removal of solubilized carbohydrate, the protein content of the processed rice flour is correspondingly enhanced to provide high-protein rice flour (HPRF). Therefore, in the present study, an attempt was made to improve the protein content and lower the GI of rice flour so that it could be used for the development of various high-protein, low-GI food products.
Materials and methods
Raw material
The paddy (Jehlum) was obtained from Mountain Research Center for Field Crops (MRCFC), Khudwani, Anantnag, J&K, India. Paddy was subjected to milling in modern rice mill (ASR RM 209). The small broken grains were ground to fineness that passed through a 200 μm sieve.
Preparation of low-GI rice flour
High-protein, low-GI rice flour was prepared by following the procedures of Chen & Chang[Citation19] with some modifications. The temperature maintained during liquefaction and saccharification was maintained at 60°C instead of 96°C used by Chen & Chang.[Citation19]
Protein content
The protein content of untreated and treated (high-protein, low-GI) rice flour was determined by the Kjeldahl method and calculated according to Eq. (1):[Citation20]
In vitro starch digestibility and GI
The in vitro starch digestibility and GI of the sample were determined by following the procedures of Goni et al.[Citation21] All of the samples except for white bread were cooked at 80°C for 30 min in tap water (5 mL) in capped tubes at which stage complete gelatinization of the sample occurred. Then, 10 mL of HCl–KCl buffer (pH 1.5) was added and homogenized for 2 min. Next, 0.2 mL of a solution containing 1 g of pepsin in 10 mL HCl–KCl buffer (pH 1.5) was added to the sample and incubated in a shaking water bath at 40°C for 60 min. Then, 15 mL of trismaleate buffer (pH 6.9) was used for adjusting the volume of the sample to 25 mL. Around 5 mL of trismaleate buffer containing 2.6 IU of α-amylase (Sigma Aldrich) from porcine pancreas was added to the sample to obtain maltodextrins; the flask was placed in a shaking water bath at 37°C. Aliquots of 0.1 mL were taken from each flask at 0, 10, 20, 30, 60, 90, 120, 150, and 180 min into the test tube and boiled in water for 5 min for inactivating the α-amylase. Then, 1 mL of 0.4 M sodium-acetate buffer (pH 4.75) and 60 μL of amyloglucosidase (Sigma Aldrich) were added and incubated at 60°C for 45 min for hydrolysing maltodextrins to glucose. The glucose concentration was measured by a glucose oxidase-peroxidase kit (Sigma Aldrich). The glucose was converted to starch according to Eq. (2):
The rate of starch digestion was expressed as the percentage of total starch hydrolysed at different times (0, 10, 20, 30, 60, 90, 120, 150, and 180 min). The kinetics of starch hydrolysis, the area under the hydrolysis curve (AUC), hydrolysis index (HI), and GI were calculated by Eqs. (3)–(6), respectively:
where C is the percentage of starch hydrolysed at time t, C∞ is the percentage of starch hydrolysed after 180 min, k is the kinetic constant (min−1), t is the time (min), tf is the final time (180 min), t0 is the initial time (0 min), and HI is the hydrolysis index, which is defined as the percentage of AUC of the treated sample divided by the corresponding area of white bread and is expressed as the percentage.[Citation22]
Thermal properties—Differential scanning calorimetry (DSC)
Thermal properties were determined by Differential Scanning Calorimeter (DSC) (Mettler Toledo) equipped with a refrigerated cooler using the method described by Mir et al.[Citation23] The moisture of the samples was adjusted to 70% by the addition of distilled water. The hydrated samples were weighed (25 ± 5 mg) into aluminium DSC pans (120 mL) and hermetically sealed. The DSC analysis was run by scanning from 20 to 120°C, ramping at 10°C/min and a hermetically sealed empty pan was used as a reference. Nitrogen was used as a purging gas. The software used for the analysis of the resulting thermograms was Stare software (ver. 9.20, Mettler Toledo). Thermal transitions of samples for gelatinization were characterized by onset temperature (To), peak temperature (Tp), endset temperature (Te), and enthalpy change (ΔH). Gelatinization enthalpy (ΔHg) was evaluated based on the area of the main endothermic peak by the Universal Analysis Program. The percent degree of gelatinization (%DG) and endotherm peak width (EPW) were estimated using Eqs. (7) and (8), respectively.
As the endotherms are essentially symmetrical, the total gelatinization temperature range (R) and peak height index (PHI) were determined using Eqs. (9) and (10), respectively.
Structural properties—Fourier Transform Infrared (FTIR) spectroscopy
Structural properties were elucidated by FTIR analysis. FTIR analysis was carried out following the methods described by Mir et al.[Citation3] About 2 mg of a properly dried sample was thoroughly mixed with 50 mg desiccated potassium bromide powder in mortar and pestle before pressing into a thin pellet. The infrared absorption spectra of the sample were obtained using an FTIR spectrometer (Thermo Nicolet 6700, Thermoscientific, UK). The equipment was operated with a resolution of 0.1 cm−1 and a scanning range of 3800–400 cm−1.
Rheological characteristics
Dough of required consistency was prepared with deionized water for measurement of rheological properties. The rheological characteristics (storage modulus G’ and loss modulus G”) of the dough were obtained using Modular Compact Rheometer (MCR-101, Anton Paar, Austria), equipped with a parallel-plate system (50 mm diameter) at a gap of 0.5 mm at 27°C. To determine the rheological data, frequency sweeps over the range of 1–100 rad/s at 3% strain were performed.[Citation24] As anticipated, the systems were non-Newtonian in nature; the indicated viscosities are to be taken as apparent viscosities. The shear rate (γ̇) and shear stress (σ) relationship of doughs was tested to see its fit for various rheological models with the correlation coefficient (R2) value at p < 0.05 using the in-built software provided by the manufacturer with the equipment. The flow curves were evaluated using Power law (Ostwald–Waele model), Herschel–Bulkley, Bingham, and Casson models. All viscosity measurements were carried out in triplicates. The following equations were used for different models:
where γ̇ is the shear rate, s−1; σ is shear stress, Pa; σo is yield shear stress, Pa; k is consistency index, Pa s; n is flow behaviour index, dimensionless. B represents the Bingham model; C represents the Casson model; HB represents the Hershel–Bulkley model; P represents the power law model; η is apparent viscosity, Pa s
Statistical analysis
The results are expressed as mean of triplicates with standard deviation. The data was analysed by ANOVA using SPSS software (SPSS PASW 18.0) at a significance level of p < 0.05. Mean values with standard deviations were reported and student’s t-test was used as the post hoc test.
Results and discussions
Protein content
shows the protein content of the untreated and treated (high-protein, low-GI) rice flour samples. It is clear from that the protein content of the treated (high-protein, low-GI) rice flour was almost 3.5 times as high as the untreated rice flour. The results are in accordance with the results shown by Chen & Chang[Citation19] wherein they have reported the protein content of HPRF to be about three times as high as the untreated rice flour.
In vitro starch digestibility
shows the in vitro starch digestibilities of untreated rice flour, treated (high-protein, low-GI) rice flour, and white bread (reference). The starch hydrolysis of both samples and white bread (reference) increased as the digestion time was increased. The white bread showed a digestion value of 75.9% after 180 min, which is in agreement with the digestion value reported by Goni et al.[Citation21]. However, some of the previous works have reported 50% digestion value within 180 min,[Citation22] which is less than the value as found by our present study. This difference could be ascribed to different methodologies used as we used an unrestricted system by including pepsin in the hydrolysis. The starch hydrolysis pattern of the treated (high-protein, low-GI) rice flour was much slower than the untreated rice flour (p < 0.05), which in turn was much slower than the white bread (p < 0.05) ().
HI and estimated GI
shows the hydrolysis kinetics of rice flour samples and white bread. There were significant differences in the kinetic constants (k) of the rice flour samples. Treated (high-protein, low-GI) rice flour had a lower kinetic constant than the untreated rice flour sample, indicating that hydrolysis occurred more rapidly in untreated rice flour than the treated rice flour. This slower hydrolysis in the treated (high-protein, low-GI) rice flour sample may be ascribed to less amount of starch due to the breakdown of starch during its preparation.
Table 1. Equilibrium concentration (C∞), kinetic constant (k), hydrolysis index (HI) and estimated GI of untreated rice flour, treated rice flour and white bread.
The HI values of untreated and treated (high-protein, low-GI) rice flour samples were found to be 87.6 ± 0.2 and 11.6 ± 0.7, respectively (), and the estimated GI values based on HI were 88 ± 0.2 and 46.1 ± 0.2, respectively. There was a significant (p < 0.05) decrease in GI. It could, therefore, be inferred that the developed (treated) rice flour is a low-GI product and may be recommended for patients suffering from type-2 diabetes. According to the World Health Organization (WHO), foods with GI < 55 are categorized as low-GI foods.[Citation25]
Thermal properties—DSC
Starch gelatinization properties of both untreated and treated rice flour samples were assessed by DSC because of its accuracy. Starch granule is semi-crystalline in nature.[Citation26] It is swollen when treated in an endothermic process and its crystallinity order becomes disordered.[Citation27] It was, therefore, important to study the gelatinization characteristics of both samples. The DSC thermograms of untreated and treated rice flour samples are presented in . The untreated rice flour shows a typical endotherm with the onset temperature (To) of 90.70 ± 0.02°C, peak temperature (Tp) of 104.3 ± 0.02°C, and endset temperature (Te) of 138.8 ± 0.02°C and enthalpy of gelatinization (ΔHg) of 10.8 ± 0.04 J/g () . However, To, Tp, Te, and ΔHg of the treated (high-protein, low-GI) rice flour were 73.80 ± 0.03°C, 76.9 ± 0.03°C, 80.3 ± 0.02°C, and 2.16 ± 0.03 J/g, respectively.
Table 2. Thermal behavior of samples.
Figure 3. Differential Scanning Calorimetry thermograms of untreated (A) and treated rice flour (B).
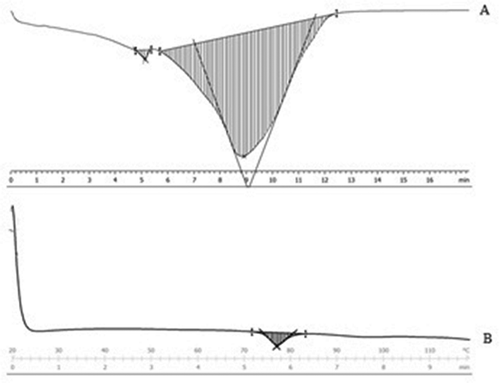
shows the thermal behaviour of the samples. It is evident from that there is a decrease in To and Tp. Enzymatic activity leads to a higher available surface area for the heating process; consequently, the pyrolysis process starts to occur at lower temperatures,[Citation25] thereby supporting our results. The decrease in Tp has also been reported by Lacerda et al.[Citation28] Also, the ΔHg of the treated (high-protein, low-GI) rice flour is less than the untreated rice flour, which may be ascribed to a decrease in crystallinity.[Citation28] Starch crystallinity is associated with the presence of amylopectin,[Citation29,Citation30] which probably did not undergo enzymatic attack with the same intensity as it has occurred in the amorphous region of the amylose.
The gelatinization temperature (Tp) reflects heat-moisture-induced transition from a crystalline to a more amorphous state.[Citation30] The gelatinization temperature (Tp) of the treated (high-protein, low-GI) rice flour samples was lower than the untreated rice flour sample; however, the degree of hydrolysis () of the former was higher than that of the latter. It clearly indicates that there exists a clear relationship between gelatinization temperature (Tp) and susceptibilities to enzyme attack. Crystalline arrangement of the starch granule plays an important role in its susceptibility to α-amylase attack,[Citation30] with treated rice flour having high gelatinization temperature starch being less susceptible to enzyme attack.
In short, as is evident from the results (), there was a decrease in transition temperatures and the values of enthalpy of gelatinization (ΔHg). The decrease in transition temperatures and the enthalpy values of gelatinization (ΔHg) for hydrolysed starch may be due to the attacked amylose in the amorphous region influenced by amylase.[Citation31] ΔHg values primarily reflect the loss of a double helical order rather than the loss of crystalline order.[Citation32] The decrease in transition temperatures and ΔHg values of the hydrolysed starch after amylase treatment may also be due to the reason that some of the double helices present in the crystalline and non-crystalline regions of the granule may have got disrupted under the conditions prevailing during amylase treatment. The decrease in ΔHg values indicates that hydrolysed starch required less thermal energy for gelatinization compared to the native starches (untreated rice flour). The decrease in transition temperatures may also be attributed to the presence of protein since proteins inhibit gelatinization by absorbing water, which would otherwise be used for gelatinization.[Citation33] Referring to our present study, treated (high-protein, low-GI) rice flour may require less thermal energy for gelatinization than that required for untreated rice flour. Heat of gelatinization denotes the energy required for disrupting the starch granule structure and since the structure of the starch of the treated (high-protein, low-GI) rice flour is already disrupted during its preparation, less heat will be required for gelatinization.
Untreated rice flour sample shows bimodal starch gelatinization endotherm () characterized by a low temperature shoulder followed by the main endotherm. In contrast, the treated (high-protein, low-GI) rice flour yielded a unimodal starch gelatinization endotherm (). The small endotherms are attributed to the melting of an amylose–lipid complex formed during heating in the calorimeter or already present in the flour.[Citation34] Since most of the starch content of the treated rice flour sample was hydrolysed during its preparation, therefore it contains less amylose. Thus, the treated rice flour sample apparently does not have sufficient lipid-associated amylose to produce a detectable small endotherm. Melting of these complexes likely occurred in treated (high-protein, low-GI) rice flour. The presence of these thermal curves was probably observed by the much gelatinization endotherms or occurred at temperatures higher than could be recorded by the calorimeter.[Citation35]
The degree of gelatinization (DG%) was found to be 80±0.3%, indicating that 20% of the starch remained ungelatizned. Differences in EPW and gelatinization temperature range (R) () between untreated (34.5±0.005°C and 27.3±0.07°C, respectively) and treated (3.4±0.01°C and 6.2±0.1°C, respectively) rice flour samples may be due to the presence of crystallites, which are composed of small crystallites, each possessing slightly different crystal strength. Therefore, the extent of decrease in EPW and R could be attributed to disrupted crystallites by heating and enzymatic treatment.[Citation36] The higher value of R in the untreated rice flour suggests the presence of crystallites of varying stabilities within the crystalline domains of its granule.[Citation37,Citation38] PHI, a measure of uniformity of gelatinization, was found to be the lowest in the treated rice flour sample (0.7 ± 0.02), which was significantly (p < 0.05) different from the untreated rice flour (0.8 ± 0.00).
Structural properties—FTIR spectroscopy
The FTIR spectrum of starch is reported to be sensitive to the changes in structure at a molecular level (short-range order), such as starch chain conformation, crystallinity, and retrogradation.[Citation38,Citation39] The FTIR spectra of the untreated and treated (high-protein, low-GI) rice flour are presented in . The FTIR spectra at 1045 cm−1 and 1015 cm−1 are known to be sensitive to changes in the crystalline and amorphous regions, respectively, near the granular surface of starch.[Citation40] The ratio of peaks at 1045 cm−1 and 1015 cm−1 of the treated rice flour was significantly (p < 0.05) lower than the untreated rice flour (), which could be ascribed to the dissociation and unravelling of double helices that form the crystalline structure.[Citation39] These results suggest that the crystallinity of flour was lost on enzymatic degradation. These results further support the thermal properties—a decrease in transition temperatures and enthalpy of gelatinization.
As depicted in and , at 1150 cm−1 and 1077 cm−1, there is a reduction in the intensity of the bands from 269.125 (untreated rice flour) to 9.053 (treated rice flour) and 137.481 (untreated rice flour) to 7.312 (treated rice flour), respectively. Furthermore, there is an increase in the intensity of the bands at 1208 cm−1 ( and ). These results suggest that the ordered structure of native starch (in treated rice flour) was disrupted due to the enzymatic degradation and the starch of the treated rice flour is more amorphous in nature. Shanava[Citation41] has reported the same results for cassava starch treated with α-amylase. Again, a very interesting finding from our results was that the native starch (of untreated rice flour) showed a prominent band at 929 cm−1 (0.139) compared to the treated rice flour (151.702). This band is sensitive to water and the characteristic index of hydrophilicity of starches[Citation42] and the intensity of this band is decreased upon enzymatic modification.[Citation41] The results presented in suggest the decrease in the ordered structure of starch and the formation of an amorphous structure.[Citation43]
Rheological properties
Rice starches are believed to be pseudoplastic (shear-thinning) as has been reported in previous studies.[Citation44] We also obtained similar results as both the untreated and treated rice flour samples were found to be non-Newtonian (shear-thinning), since the value of n is less than 1. It is known that the farther the value of n departs from 1, the greater is the deviation from the Newtonian flow.[Citation45] Thus, in our present study, the value of n is very much less than 1, deviating more from Newtonian behaviour. It could, therefore, be inferred that both the samples (untreated and treated) exhibited non-Newtonian shear-thinning (pseudoplastic) behaviour, which is characterized by a decrease in viscosity with an increase in shear rate.
Dynamic rheology
Dynamic frequency sweep tests were performed in the limit of the linear viscoelastic region to determine the frequency dependency of elastic (G´) and viscous (G´´) moduli. The viscoelastic behaviour was obtained over a frequency range of 0.1–100Hz. shows the changes in storage modulus (G´) (5A) and dissipation/loss modulus (G´´) (5B) as a function of frequency. (G´/ω) and 5b (G´´/ω) represents the frequency sweep of rice flour samples. The magnitude of G´ increased with an increase in the frequency with less frequency dependency. As shown in , G´ was greater in untreated rice flour than that of treated rice flour, suggesting a more elastic behaviour of untreated rice flour than the treated one. shows the increase in G´´ with an increase in frequency (ω) and shows the frequency dependency of the two. As can be seen in , G´´ first decreases and then increases with an increase in the frequency (ω). The Herschel–Bulkley model gave the best fit for both untreated rice flour (R2 = 0.9922) and treated rice flour (R2 = 0.9997) () . Knowledge of the flow behaviour of rice dough in terms of γ̇ and σ and the models to which they fit is important for the design of flow systems, selection of pumps, scale-up, and mechanization of the process.[Citation24]
Table 3. Rheological modeling parameters.
Figure 5. Frequency sweep of rice flour. 5A: Storage modulus versus angular frequency; 5B: Loss modulus versus angular frequency, 5C: Damping factor (tanδ) versus angular frequency (ω).
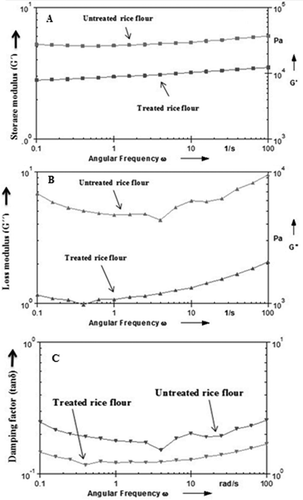
shows the relationship between the damping factor (tanδ) and the angular frequency (ω). Damping factor (tanδ) is lower for the untreated rice flour that that of the treated rice flour, suggesting a more elastic behaviour of the latter than former. The increase in peak in G´ and G´´ occurs due to the decrease in associative forces within the starch granule.[Citation39] The reduction in tanδ of the untreated rice flour sample suggests that G´ increases more strongly than G´´. The untreated rice flour samples are less frequency dependent than the treated rice flour; therefore, it is suggested that the untreated rice flour dough is strong and resistant to stresses or conditions used in the industry.[Citation46]
Conclusion
It was concluded from the present study that the protein content of rice flour was correspondingly increased, suggesting its use in infant formula and/or other protein-fortified foods. Also the GI of rice flour was reduced from 88 ± 0.16 to 46 ±0.17, suggesting its use in food products meant for diabetic patients as foods with GI less than 55 are considered to be low-GI foods and safe for diabetic patients as recommended by WHO. Treated rice flour showed a slower hydrolysis pattern than untreated flour possibly due to the breakdown of starch during its preparation. Further, the transition temperatures and enthalpies of gelatinization were reduced by enzymatic hydrolysis. Untreated as well as treated rice flour exhibited non-Newtonian pseudoplastic (shear-thinning) behaviour, which is characterized by the decrease in apparent viscosity with increase in shear rate. The Herschel–Bulkley model gave the best fit for both samples.
References
- Prasad, K.; Singh, Y.; Anil, A. Effects of Grinding Methods on the Characteristics of Pusa 1121 Rice Flour. Journal of Tropical Agriculture and Food Science 2012, 40 (2), 193–201.
- Srikaeo, K.; Arranz-Martínez, P. Formulating Low Glycaemic Index Rice Flour to be Used as a Functional Ingredient. Journal of Cereal Science 2015, 61, 33–40.
- Mir, S.A.; Bosco, S.J.; Shah, M.A.; Mir, M.M.; Sunooj, K.V. Process Optimization and Characterization of Popped Brown Rice. International Journal of Food Properties 2015. doi:10.1080/10942912.2015.1105254.
- Umadevi, M.; Pushpa, R.; Sampathkumar, K.P.; Bhowmik, D. Rice-Traditional Medicinal Plant in India. Journal of Pharmacognosy and Phytochemistry 2012, 1(1), 6–12.
- Hu, P.; Zhao, H.; Duan, Z.; Linlin, Z; Wu, D. Starch Digestibility and the Estimated Glycemic Score of Different Types of Rice Differing in Amylose Contents. Journal of Cereal Science 2004, 40, 231–237.
- Villareal, C.P.; Juliano, B.O.; Hizukuri, S. Varietal Differences in Amylopectinstaling of Cooked Waxy Milled Rices. Cereal Chemistry 1993, 70, 753–758.
- Prasad, K.; Prakash, P.; Prasad, K.K. Rice Based Functional Cookies for Celiac: Studies on its Formulation; LAP Lambert Academic Publishing: Saarbrucken, Germany, 2010; 128 pp.
- Rattanamechaiskul, C.; Soponronnarit, S.; Prachayawarakorn, S. Glycemic Response to Brown Rice Treated by Different Drying Media. Journal of Food Engineering 2014, 122, 48–55.
- Hu, E.A.; Pan, A.; Malik, V.; Sun, Q. White Rice Consumption and Risk of Type 2 Diabetes: Meta-Analysis and Systematic Review. British Medical Journal 2012, 344, e1454.
- McGonigal, A.; Kapustin, J. Low-Glycemic Index Diets: Should they be Recommended for Diabetics? Nurse Practitioners 2008, 4, 688–696.
- Jobling, S. Improving Starch for Food and Industrial Applications. Current Opinion in Plant Biology 2004, 7, 210–218.
- Zhang, G.Y.; Hamaker, B.R. Slowly Digestible Starch: Concept, Mechanism, and Proposed Extended Glycemic Index. Critical Reviews in Food Science and Nutrition 2009, 49, 852–867.
- Mann, J.; Cummings, J.H.; Englyst, H.N.; Key, T.; Liu, S.; Riccardi, G.; Summerbell, C.; Uauy, R.; Dam, R.M.v.; Venn, B.; Vorster, H.H.; Wiseman, M. FAO/WHO Scientific Update on Carbohydrates in Human Nutrition: Conclusions. European Journal of Clinical Nutrition 2007, 61, S132–S137.
- Brownlee, M. Biochemistry and Molecular Cell Biology of Diabetic Complications. Nature 2001, 414, 813–820.
- Ludwig, D.S. The Glycemic Index: Physiological Mechanisms Relating to Obesity, Diabetes, and Cardiovascular Disease. Journal of American Medical Association 2002, 287, 2414–2423.
- Lee, C.K.; Le, Q.T.; Kim, Y.H.; Shim, J.H.; Lee, S.J.; Park, J.H.; Lee, K.P.; Song, S.H.; Auh, J.H.; Lee, S.J.; Park, K.H. Enzymatic Synthesis and Properties of Highly Branched Rice Starch Amylose and Amylopectin Cluster. Journal of Agricultural and Food Chemistry 2008, 56, 126–131.
- Le, Q.T.; Lee, C.K.; Kim, Y.; Lee, S.J.; Zhang, R.; Withers, S.G.; Kim, Y. R.; Auh, J.H.; Park, K.H. Amylolytically-Resistant Tapioca Starch Modified by Combined Treatment of Branching Enzyme and Maltogenic Amylase. Carbohydrate Polymers 2009, 75, 9–14.
- Euber, J.R.; Puski, G.; Hartman, G.H. Patent No. 4,990,344. United States 1991.
- Chen, W.P.; Chang, Y.C. Production of High-Fructose Rice Syrup and High-Protein Rice Flour from Broken Rice. Journal of the Science of Food and Agriculture 1984, 35, 1128–1135.
- Amin, T.; Thakur, M.A. Comparative Study on Proximate Composition, Phytochemical Screening, Antioxidant and Antimicrobial Activities of Linum usitatisimum L. (flaxseeds). International Journal of Current Microbiology and Applied Sciences 2014, 3 (4), 465–481.
- Goni, I.; Garcia, A.A.; Saura, C.F. A Starch Hydrolysis Procedure to Estimate Glycemic Index. Nutrition Research 1997, 17, 427–437.
- Granfeldt, Y.; Bjorck, I.; Drews, A.; Tovar, J. An In-Vitro Procedure Based on Chewing to Predict Metabolic Response to Starch in Cereal and Legume Products. European Journal of Clinical Nutrition 1992, 46, 649–660.
- Mir, S.A.; Bosco, S.J.; Bashir, M.; Shah, M.A.; Mir, M.M. Physicochemical and Structural Properties of Starches Isolated from Corn Cultivars Grown in Indian Temperate Climate. International Journal of Food Properties 2016. doi:10.1080/10942912.2016.1184274.
- Guha, M.; Ali, S.Z. Changes in Rheological Properties of Rice Flour During Extrusion Cooking. Journal of Texture Studies 2011, 42(6), 451–458.
- Nayak, B.; Berrios, J.D.; Tang, J. Impact of Food Processing on the Glycemic Index (GI) of Potato Products. Food Research International 2014, 56, 35–46.
- Naknaen, P.; Tobkaew, W.; Chaichaleom, S. Properties of Jackfruit Seed Starch Oxidized with Different Levels of Sodium Hypochlorite. International Journal of Food Properties 2016. doi:10:1080/10942912.2016.1191868.
- Gujral, H.S.; Sharma, P.; Kaur, H.; Singh, H. Physicochemical, Pasting and Thermal Properties of Starch Isolated from Different Barley Cultivars. International Journal of Food Properties 2013, 16, 1494–1506.
- Lacerda, L.G.; Filho, M.A.; Demiate, I.M.; Bannach, G.; Ionashiro, M.; Schnitzler, E. Thermal Behaviour of Corn Starch Granules Under Action of Fungal Alpha-Amylase. Journal of Thermal Analysis and Calorimetry 2008, 93 (2), 445–449.
- Yu, L.; Christie, G. Measurement of Starch Thermal Transitions Using Differential Scanning Calorimetry. Carbohydrate Polymers 2001, 46, 179.
- Zhang, G.; Ao, Z.; Hamaker, B.R. Slow Digestion Property of Native Cereal Starches. Biomacromolecules 2006, 7, 3252.
- Xiao, H.; Lin, Q.; Liu, G. Q. Effect of Cross-Linking and Enzymatic Hydrolysis Composite Modification on the Properties of Rice Starches. Molecules 2012, 17, 8136–8146.
- Cooke, D.; Gidley, M.J. Loss of Crystalline and Molecular Order During Starch Gelatinization: Origin of the Enthalpic Transition. Carbohydrate Research 1992, 227, 103–112.
- Iturriaga, L.; Lopez, B.; Anon, M. Thermal and Physicochemical Characterization of Seven Argentine Rice Flours and Starches. Food Research International 2004, 37, 439–447.
- Biliaderis, C.G.; Page, C.M.; Maurice, T.J.; Juliano, B.O. Thermal Characterization of Rice Starches: A Polymeric Approach to Phase Transitions of Grnular Starch. Journal of Agricultural and Food Chemistry 1986, 34, 6.
- Marshall, W.E.; Normand, F.L. Exothermic Transitions in Whole Grain Milled Rice and Milled Rice Flour Sudied by Differential Scanning Calorimetry. Cereal Chemistry 1991, 68, 606.
- Miao, M.; Zhang, T.; Mu, W.; Jiang, B. Effect of Controlled Gelatinization in Excess Water on Digestibility of Waxy Maize Starch. Food Chemistry 2010, 119, 41–48.
- Hoover, R.; Li, Y.X.; Hynes, G.; Senanayake, N. Physicochemical Characterization of Mung Bean Starch. Food Hydrocolloids 1997, 11, 401–408.
- Sandhu, K.S.; Singh, N. Some Properties of Corn Starches II: Physicochemical, Gelatinization, Retrogradation, Pasting and Gel Textural Properties. Food Chemistry 2007, 101, 1499–1507.
- Chung, H.J.; Liu, Q.; Hoover, R. The Impact of Single and Dual Hydrothermal Modifications on the Molecular Structure and Physicochemical Properties of Normal Corn Starch. International Journal of Biological Macromolecules 2009, 44, 203–210.
- Bian, L.; Chung, H.J. Molecular Structure and Physicochemical Properties of Starch Isolated from Hydrothermally Treated Brown Rice Flour. Food Hydrocolloids 2016, 60, 345–352.
- Shanava, S. Studies on Modified Tuber Starches Useful for Tablets and Capsules. Division of Crop Utilization Central Tuber Crops Research International (Indian Council of Agricultural Research) Thiruvanthapuram: Kerala, India, 2013; 695017 pp.
- Alexandar, R.J. Maltodextrins: Production, Properties and Applications. In Starch Hydrolysis Products: Worldwide Technology, Production and Applications; Schenck, F.W.; Hebesds, R.E.; Eds.; VCH Publishers: New York, 1992; 233–276.
- Sevenou, O.; Hill, S.E.; Farhat, I.A.; Mitchell, J.R. Organization of the External Region of the Starch Granule as Determined by Infrared Spectroscopy. International Journal of Biological Molecules 2002, 32, 79–85.
- Zhang, T.; Oates, C. Relationship Between Alpha-Amylase Degradation and Physico-Chemical Properties of Sweet Potato Starches. Food Chemistry 1999, 65, 157–163.
- Bourne, M.C. Food Texture and Viscosity: Concept and Measurement (Second Edition). Academic Press: London, 2002; 89 pp.
- Pepe, L.S.; Moraes, J.; Albano, K.M.; Telis, V.R.N.; Franco, C.M.L. Effect of Heat-Moisture Treatment on the Structural, Physicochemical, and Rheological Characteristics of Arrowroot Starch. Food Science and Technology International 2015, 22 (3), 256–265.