ABSTRACT
In this work, Geobacillus sp. TF16 phytase was separately immobilized in chitosan and Ca-alginate with the efficiency of 38% and 42%, respectively. These enzymes exhibited broad substrate specificity. Maximal relative phytase activity was measured at pH 5.0 and 95°C and pH 3.0 and 75°C for chitosan and Ca-alginate, respectively. The enzymes were highly stable in a wide pH and temperature range. Values of Km and Vmax were determined as 2.38 mM and 3401.36 U/mg protein for chitosan, and 7.5 mM and 5011.12 U/mg protein for Ca-alginate. The immobilized enzymes showed higher resistance to proteolysis. After 4 h incubation, hydrolysis capacities of chitosan- and Ca-alginate immobilized enzymes for soymilk phytate were calculated as 24% and 33%, respectively. The chitosan- and Ca-alginate immobilized phytases conserved its original activity after 8 and 6 cycles of reuse, respectively. The features of the enzymes were very attractive and they might be useful for some industrial applications.
Introductıon
Phytic acid is the most important storage form of inositol and phosphate in plants and constitutes approximately 5% of the dry weight of seeds in legumes and cereal grains.[Citation1] Phytic acid can form complexes with proteins and ions such as magnesium, calcium, zinc, and iron. For this reason, it is an antinutritional factor in animals.[Citation2–Citation4] Because of the insufficient amount of phytate degrading enzymes, monogastric animals such as swine, chickens, and humans are unable to benefit from phytate phosphorus. In this way, unutilized phytate phosphorus is excreted and becomes a pollutant for the environment in the areas of livestock units.[Citation5] Addition of phytases to animal feed can be an effective strategy to decrease phosphorous contamination and increase the bioavailability of phosphorous and essential minerals to animals.[Citation6,Citation7]
Phytases catalyze the hydrolysis of phytic acid to phosphoric acid and myo-inositol. This activity produces a non-metal chelator compound and available phosphate. They are valuable enzymes to upgrade the nutritional quality of feeds and foods containing high amount of phytate.[Citation8] In addition, phytases have important applications in ameliorating human nutrition[Citation9–Citation11] and aquaculture.[Citation12] Phytase activity also reduces the pollution in ground- and surface-water caused by the phosphorus and phytate in manure in extended livestock regions.[Citation13] Addition of phytase to diets of monogastric animals reduces the fecal phosphate excretion approximately by 50%.[Citation14]
Although phytases are found widely in nature and can be taken from a variety of plants and animals, microbial sources are more effective for phytase production on a large scale because of thermal stability, resistance to proteolysis, wide pH profile, and substrate specificity.[Citation15–Citation17] Therefore, finding new microbial phytases containing desired enzymatic characteristics is very important. Nowadays, especially thermal stabile phytases have an important role, as feed pelleting process requires temperatures higher than 50°C.[Citation14]
Enzymes used in industrial processes have to have some features such as stability at processing and storage conditions. Also they can be produced cheaply. The uses of phytases to produce specific myo-inositol phosphate isomers for use in medicine are an exciting avenue. However, the reuse of phytases is hampered by their low stability under operational conditions and their difficulties to be recovered from the reaction mixtures after catalysis. These problems have been overcome by immobilization of phytases on a variety of matrices.[Citation18] Immobilized enzymes have some advantages. They have high stability than free enzymes and can be easily separated from the reaction mixture. Thus, enzyme consumption is considerably reduced.[Citation19]
In this study, a novel phytase purified from thermophilic Geobacillus sp. TF16 strain was separately immobilized in chitosan by covalent binding and Ca-alginate by entrapment. Chitosan has many characteristics like hydrophilicity, biocompatibility, and low biodegradability, high permeability toward water, good adhesion and high affinity toward proteins. It is an inexpensive, inert, non-toxic, high mechanical strength support.[Citation20–Citation22] For these reasons, chitosan is known as an ideal support material for enzyme immobilization.
Entrapment method differs from other immobilization techniques in that enzyme molecules are free in solution, but restricted in movement by the lattice structure of the gel.[Citation23] Entrapment of enzyme within insoluble calcium alginate gel is recognized as a rapid, nontoxic, inexpensive, and versatile method for immobilization of enzymes and cells.[Citation24]
This is the first report immobilizing a phytase from a Geobacillus strain and focuses on this enzyme as an additive for the food/feed industry.
Material and methods
Geobacillus sp. TF16 isolated from Germencik Ömerbeyli Jeotermal in Aydın city (Turkey) was provided from Department of Biology, Karadeniz Technical University (Trabzon/Turkey). The accession number is KY013619 (Genbank).
Purification of Geobacillus sp. TF16 phytase
The enzyme was previously precipitated by using ammonium sulfate and purified with DEAE-Sepharose Fast Flow ion-exchange chromatography.[Citation25]
Immobilization of purified enzyme in chitosan particles by covalent binding
The purified enzyme was immobilized in chitosan by covalent binding.[Citation26–Citation28] For this purpose, 1 g chitosan was stirred with 75 mL, 0.1 M HCl solution containing 2.5% (v/v) glutaraldehyde for 2 h at 30°C. Then 10 mL, 0.1 M NaOH solution was added and the precipitate formed was collected by filtration. Then, chitosan molecules were washed two times with deionized water for removing of the non-binding glutaraldehyde from the mixture. Wet chitosan was slowly stirred with 1 mg of pure free phytase at 4°C for 1 h. After filtration of the mixture, immobilized enzyme–chitosan molecules were washed several times with distilled water to remove the non-binding enzyme until the absorbance was lower than 0.01 at 280 nm. Total protein concentration in the filtrate was determined and percentage of the phytase binding efficiency (E) was defined as follows:
where C1 is protein concentration of solution before immobilization, and C0 is protein concentration of solution after immobilization.[Citation29]
Entrapment of purified phytase in alginate/CaCl2 beads
To entrap the enzyme, first sodium alginate solution was prepared in deionized water at a concentration of 2% (w/v). Then, 2 mg of protein was added to 10 mL of sodium alginate solution. The final mixture was dropped using a syringe into 100 mL solution of 2% (w/v) CaCl2. The beads were stirred for 15–20 min and left for 24 h at 4°C to get the final hardened form. The final macro beads were removed and washed several times with deionized water for removal of excess CaCl2. Total protein concentration in the filtrate was determined, and the percentage of the binding protein was calculated.[Citation30]
Determination of protein concentration
Protein concentration was determined according to the Bradford method using bovine serum albumin (BSA) as standard.[Citation31]
Enzyme activity assay and substrate specificity
Phytase activity was spectrophotometrically determined by using sodium phytate, 4-methylumbelliferil phosphate, glucose 6-phosphate, ADP, and ATP as the substrates.[Citation32] The mixture of 200 μL immobilized enzyme solution and 600 μL of substrate solution (2 mM substrate in 50 mM, pH 4.0 Mcilvaine buffer containing 2 mM CaCl2.2H2O) were incubated at appropriate temperature for 30 min. The reaction was stopped by adding 800 μL of trichloroacetic acid (TCA, 15% (w/v)). Then, 800 μL of the coloring reactive (6 N H2SO4:2.5% ammonium molybdate:10% ascorbic acid:deionized water in the ratio of 1:1:1:2) was added to the mixture. After incubation at 25°C for 10 min, the absorbance at 625 nm was determined. A reference standard (KH2PO4, 0.5–7.5 μg/mL) was simultaneously assayed with the samples. One unit (U) of phytase activity was defined as the amount of enzyme required to release 1 μmol of Pi from Na-phytate per minute under the standard assay conditions. All the samples were assayed in triplicates.
Optimum pH and pH stability of enzymes
Optimum pH of the immobilized enzyme was determined at 55°C by using sodium phytate and 50 mM buffers: glycine–HCl (pH 2.0), Mcilvaine (pH 3.0–7.0), and glycine–NaOH (pH 8.0–9.0). The maximum activity was taken as 100%, and the relative activities were calculated by comparing with it.[Citation33] pH stability of the chitosan-immobilized enzyme was determined by incubating the enzyme separately at 4°C, 35°C, and 95°C for different times in the buffer solutions of pH 2.0 (50 mM glycine–HCl), pH 5.0 (50 mM sodium acetate), and pH 7.0 (50 mM Tris-HCl). For the enzyme immobilized in alginate/CaCl2 beads, the incubation was made at 4°C, 35°C, and 75°C for different times in the buffer solutions of pH 3.0 (50 mM glycine–HCl), pH 5.0 (50 mM sodium acetate), and pH 7.0 (50 mM Tris-HCl). After incubation, the phytase activity was determined under standard conditions. The residual activities (%) were calculated by comparison with the unincubated enzyme.[Citation33]
Optimum temperature and thermal stability of enzymes
The optimum temperature was determined at an optimal pH value at various temperatures in the range of 25–95°C by using sodium phytate as substrate. The maximum activity was taken as 100% and the relative activities were calculated by comparison.[Citation34] The enzyme solutions in 50 mM pH 5.0 sodium acetate buffer were separately incubated at 4°C, 35°C, and 95°C for chitosan, and 4°C, 35°C, and 75°C for Ca-alginate at different times to determine the thermal stability of the phytase. After then, the enzyme activity was assayed at optimum conditions, the residual activity (%) was calculated by comparison with unincubated phytase.[Citation33]
Kinetic parameters
Kinetic parameters of the immobilized enzymes were determined by measuring the rate of phytate hydrolysis at the concentrations of Na-phytate ranging from 0.08 to 6.67 mM in optimum conditions. Km and Vmax values were calculated from the Lineweaver–Burk plot.
Effect of some metal ions and chemicals
Chloride salts of Na+, K+, Li+, Co2+, Fe2+, Mg2+, Cu2+, Zn2+, Mn2+, Ca2+, Hg2+, Ni2+, Al3+, and Fe3+ were separately added to the reaction mixture in final concentrations of 1, 5, and 10 mM. Activity determined at standard reaction conditions without metal ion was taken as 100%.[Citation34] To study the effect of some chemicals on the enzyme activity, SDS, triton X-100, triton X-114, Tween-20, ethanol, methanol, acetone, and 2-propanol were added separately into reaction mixtures in the final concentrations of 1%, 5%, and 10%. Also the effect of EDTA and phenylmethanesul-fonylfluoride (PMSF) was investigated at the concentration of 1, 5, and 10 mM. The residual activities (%) were calculated by comparison with standard assay mixture without any chemicals.[Citation35]
Resistance of immobilized enzymes to proteolysis
To measure the resistance of immobilized phytase against trypsin and chymotrypsin, the enzyme (0.1 mg/mL final concentration) was incubated with protease mix (in the final concentration of 0.1 mg/mL) (trypsin–chymotrypsin; Sigma-Aldrich, Protease Type VIII; bacterial from Bacillus licheniformis) in 50 mM, pH 7.5 Tris-HCl buffer at the ratio of 1:1 (w/w) for 30, 60, and 90 min at 37°C. The residual activities (%) were calculated by comparison with the standard assay mixture without any proteases.[Citation36]
Dephytinization of soymilk by immobilized enzymes
The potential application of the immobilized phytases in food industry was assessed according to Pi release from soymilk. Therefore soybean seeds were incubated overnight in deionized water and thoroughly crushed in a mixer. The slurry was filtered by using double-layered muslin cloth and the liquid was used as soymilk. The obtained soymilk was incubated with the enzyme (10 U/mL) at standard assay conditions. The samples were drawn at desired periods and centrifuged. Then samples were analyzed with standard activity assay for inorganic phosphate released. A standard calibration curve was used to calculate the phosphate released. For this purpose, K2HPO4 between 0.5 and 5.0 mM were used as the substrate, and standard assay was performed.[Citation37]
Determination of reusability
The stabilities of immobilized phytases on repeated use were examined by measuring the enzyme activity after each successive run. The immobilized phytase was incubated with substrate solution and it was collected by centrifugation at 4000 rpm at the end of the reaction and washed with distilled water. The released Pi in supernatant was calculated according to the standard enzyme assay. For the second cycle, the immobilized phytase was redissolved in appropriate volume of buffer solution and processed the same way as before.[Citation38]
Results and discussion
In this study, a phytase purified previously from the thermophilic bacterium Geobacillus sp. TF16 was separately immobilized in chitosan and alginate/CaCl2 beads. The immobilized enzyme was then characterized biochemically. Dephytinization of soymilk by the immobilized enzymes was also examined.
Immobilization of purified enzyme in chitosan particles and alginate/CaCl2 beads
The binding efficiency of the enzyme to chitosan and alginate/CaCl2 was 38% and 42%, respectively. It was previously reported that the purified Pantoea agglomerans phytase was entrapped in alginate beads with an efficiency of approximately 80%.[Citation18] Aspergillus ficuum phytase was covalently immobilized onto cross-linked agarose by 55% efficiency.[Citation39] Saccharomyces cerevisiae CY cells immobilized in calcium alginate beads were used for the degradation of phytate. Immobilization yield of the phytase reached 43%.[Citation40]
Substrate specificity
Substrate specificity of the immobilized phytase was tested on sodium phytate, 4-methylumbelliferil phosphate, glucose 6-phosphate, ADP, and ATP. Chitosan-immobilized enzyme exhibited broad substrate specificity and was more active to hydrolyze sodium phytate (). Relative activity of the enzyme was 74% and approximately 40% in the presence of 4-methylumbelliferil phosphate and the other substrates, respectively. Ca-alginate-immobilized phytase was also more active in the presence of sodium phytate (). The enzyme also hydrolyzed the other substrate in different ratios.
Table 1. Substrate specificity of Geobacillus sp. TF16 immobilized phytase.
Optimum pH and pH stability of enzymes
Effect of pH on the immobilized enzyme was analyzed at 55°C between pH values of 2.0 and 9.0 using 50 mM buffers. Maximal relative phytase activity was measured at pH 5.0 and 3.0 in the case of chitosan and Ca-alginate, respectively (). Similar results were found before for other phytases. E. coli phytase had an optimum pH of 5.0 after immobilization on nanoclays.[Citation41] The optimum pH for immobilized Saccbaromyces cerevisiae CY phytases in soybean curd whey was determined as 3.5.[Citation40] A concentrated extract from soybean sprout containing an active phytase was immobilized on Sepabead EC-EP, and the optimum pH of immobilized enzyme was found as 5.0.[Citation37]
Figure 1. (a) Effect of pH on Geobacillus sp. TF16 phytase immobilized in chitosan and Ca-alginate. Activity was assayed at 55°C by using sodium phytate as a substrate and 50 mM buffers: glycine–HCl (pH 2.0), Mcilvaine (pH 3.0–7.0), and glycine–NaOH (pH 8.0–9.0). The maximum activity was taken as 100%, and the relative activities were calculated by comparing with it. (b) The optimum temperatures of the enzymes were determined by measuring the activity at temperatures between 25°C and 95°C by using sodium phytate as substrate. The maximum activity was taken as 100% and the relative activities were calculated by comparing with it.
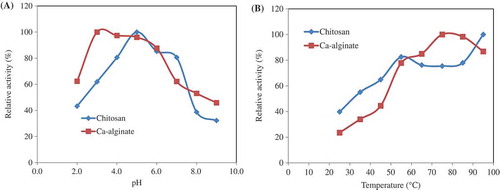
The pH stability of the chitosan-immobilized enzyme was determined at 4°C, 35°C, and 95°C in the buffer solutions of pH 2.0, 5.0, and 7.0. Immobilized phytase was stable at all pH values at 4°C, and retained its original activity approximately 60–90% after 12 h incubation (). Also, the enzyme conserved its original activity approximately 70% after 2 days at pH 5.0. Consequently, it can be said that storage of the chitosan-immobilized enzyme was very suitable at 4°C. The enzyme activity was conserved approximately 70–80% after 6 h incubation at 35°C (). It conserved its original activity approximately 75% and 65% at pHs 5.0 and 7.0, respectively, at 95°C after 12 h incubation. The enzyme displayed 60% activity after 48 h at pH 5.0 (Fig. 2c).
Figure 2. The pH stability of the chitosan-immobilized enzyme was determined at 4°C (a), 35°C (b), and 95°C (c) for different incubation times in the buffer solutions of pH 2.0, pH 5.0, and pH 7.0. At the end of the incubation period, the phytase activity was assayed at optimum conditions. The residual activity (%) was calculated by comparison with unincubated phytase.
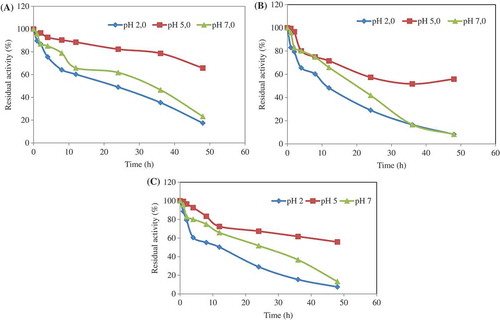
pH stability of the immobilized phytase in alginate/CaCl2 beads was analyzed by incubating the enzyme at 4°C, 35°C, and 75°C in the buffer solutions of pH 3.0, 5.0, and 7.0. After 12 and 36 h incubation, the enzyme retained at approximately 60–80% and 40–70% of its original activity for all pH values at 4°C (). The enzyme activity was conserved approximately 85% after 2 h incubation at 35°C at all pH values (). The enzyme retained nearly 58%, 42%, 45% of its original activity at pH 3.0, 5.0, 7.0, respectively, after 12 h at 75°C. After 1 h incubation, the enzyme activity was conserved at approximately 80% at all pH values (Fig. 3c).
Figure 3. pH stability was separately determined by incubating the enzyme entrapped in alginate/CaCl2 beads at 4°C (a), 35°C (b), and 75°C (c) for different incubation times in the buffer solutions of pH 3.0, pH 5.0, and pH 7.0. At the end of the incubation period, the phytase activity was assayed at optimum pH and temperature values. The residual activity (%) was calculated by comparison with unincubated enzyme.
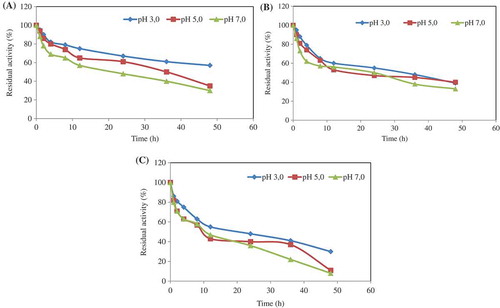
It was reported that the original activity of the immobilized S. cerevisiae phytase lost approximately 80% at pH 2.0 after only 30 min. The loss in activity at pH 4.0 and above was over 50%.[Citation40] Also, immobilized phytase on epoxy-activated Sepabead EC-EP conserved all of its original activity at pH 5.0 and 37°C after 3 h incubation.[Citation37] The immobilized Aspergillus niger phytase was stable at low pH values and retained full of its original activity after incubation at pH 2.0 for 24 h at 4°C and 92% after incubation at pH 2.0 and 37°C.[Citation42] From these results it can be said that the immobilized Geobacillus sp. TF16 phytases were very stable at a wide range of pH and this can be attractive for the industrial applications of the enzymes.
Optimum temperature and thermal stability of enzymes
Enzyme activity was investigated at a range of temperature between 25°C and 95°C. Data showed that the highest activity was at 95°C and 75°C for chitosan and Ca-alginate, respectively (). The optimum temperature of immobilized S. cerevisiae and epoxy-activated Sepabead EC-EP-immobilized phytase was reported to be 50°C and 60°C, respectively.[Citation37,Citation40] Optimum activity of the phytases covalently bound onto Fe3O4 magnetic nanoparticles was found at 60°C (rye), 65°C (A. niger), and 70°C (Escherichia albertii).[Citation43] In line with these results, it is seen that optimum temperatures for chitosan and Ca-alginate-immobilized enzymes are higher than for many other purified phytases so far.
Thermal stabilities of Geobacillus sp. TF16 immobilized phytases were determined by pre-incubating the enzymes at various times at 4°C, 35°C, and 95°C for chitosan and at 4°C, 35°C, and 75°C for Ca-alginate. It was observed that the enzyme immobilized in chitosan was highly stable at all temperatures after 12 h incubation and conserved nearly 80–85% of its activity. On the other hand, the enzyme pre-incubated at 35°C and 95°C for 48 h lost its activity approximately 55% and 80%, respectively (). The enzyme had a half-life (based on residual activity) of approximately 55, 32, and 45 h at 4°C, 35°C, and 95°C, respectively.
Figure 4. In order to determine the thermal stability of the phytase, the enzyme solutions in 50 mM (pH 5.0) sodium acetate buffer were separately incubated at 4°C, 35°C, and 95°C chitosan (a), and 4°C, 35°C, and 75°C for Ca-alginate (b) at different times. The residual activities (%) were calculated by comparison with standard assay mixture containing unincubated enzyme.
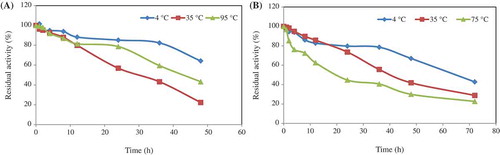
In the case of Ca-alginate, the phytase conserved its original activity approximately 80% at 4°C and 35°C and 45% at 75°C after 24 h (). The immobilized phytase was highly thermostable and had a half-life of approximately 65, 40, and 19 h at 4°C, 35°C, and 75°C, respectively. When exposed at 70°C for 1 h, the immobilized A. niger phytase retained 90% and at 80°C 62% of the initial activity.[Citation42] The immobilized E. albertii phytase retained 83% of its initial activity at 70°C and 34% at 80°C.[Citation43] Activity of the immobilized phytase from A. ficuum protected its original activity approximately 100% after 1 h incubation at 60°C and 70°C, but activity completely disappeared after 30 min at 80°C.[Citation36] A. niger phytase immobilized on montmorillonite was completely inactivated after 30 min at 60°C. However, A. niger immobilized on Al–Si–6%Fe conserved its original activity even after 120 min at 60°C.[Citation41] These results showed that the immobilized phytases were highly thermal-stable and they can be ideal candidates for industry.
Kinetic parameters of immobilized enzymes
Kinetic parameters of the immobilized enzymes were determined by measuring the rate of phytate hydrolysis at various substrate concentrations. Km and Vmax values were determined as 2.38 mM and 3401.36 U/mg protein for chitosan, 7.5 mM and 5011.12 U/mg protein for Ca-alginate, respectively. Km and Vmax values of the phytase increased after immobilization. Because it was previously reported that Km and Vmax values of Geobacillus sp. TF16 free phytase were 1.31 mM and 526.28 U/mg protein, respectively.[Citation25]
It was reported that Km and Vmax values were found to be 10 mM and 1 U/mg for immobilized avocado phytase, respectively.[Citation37] Also, Km values were calculated to be 95 μmol/L (A. niger), 220 μmol/L (E. albertii), and 455 μmol/L−1 (rye).[Citation43] A. ficuum phytase had 32.8 mM and 0.027 mM/min Km and Vmax values after immobilization.[Citation44]
Effect of some metal ions and chemicals on enzyme activity
Enzyme activity was separately investigated by adding different ions to the standard reaction mixture in a final concentration of 1, 5, and 10 mM. In the presence of 1 M metal ions, the chitosan-immobilized enzyme retained almost of its activity. The activity was stimulated approximately twofold by Cu2+ ( in the online supplementary information (SI)). The activity of chitosan-immobilized phytase was fully inhibited at the 10% final concentration of triton X-100, triton X-114, and Tween-20. But, SDS, ethanol, methanol, and 2-propanol stimulated the activity in the 1% final concentration ( in SI).
In the case of Ca-alginate, 10 mM Ca2+ activated the enzyme activity nearly 30%. The activity was inhibited in different ratios by 10 mM K+, Li+, Co2+, Mg2+, Cu2+, Zn2+, Mn2+, Hg2+, Ni2+, Al3+, and Fe3+ ( in SI). Also, triton X-100, triton X-114, and Tween-20 fully inhibited the enzyme at 10% concentration. Organic solvents used inhibited the activity at different ratios ( in SI). It was previously reported that Cu2+ and Hg22+ inhibited the activity of the phytase enzyme from Pinar melkior mushroom immobilized onto magnetite-CTS NPs. While Co2+, Fe2+, and Mg2+ ions increased the activity of immobilized phytase, Zn2+ had no effect.[Citation45]
Resistance of immobilized enzymes to proteolysis
To measure the phytase resistance against trypsin and chymotrypsin contained in the digestive tract, the immobilized enzyme was incubated with protease mix for 30, 60, and 90 min at 37°C. Both of the enzymes showed higher resistance to protases. The original phytase activity was conserved at approximately 78% and 62% after 90 min for chitosan and Ca-alginate immobilized enzyme, respectively (Fig. 5 in SI). A. niger phytase immobilized on Al–Si–6%Fe showed no significant loss of activity even after 120 min at 60°C.[Citation41] It was reported that immobilized phytases showed higher resistance to high temperatures and proteolysis than free enzymes. A similar study was reported for different enzymes immobilized on clays.[Citation46]
Dephytinization of soymilk by immobilized enzymes
The phytate of soymilk was degraded by the immobilized phytases. Phytic acid concentration of soymilk was found to be 28.16 mM. Enzymes and soymilk were incubated at optimal conditions, and examples were taken every 30 min to 4 h. The amount of phosphate liberated was determined by using a standard calibration curve of K2HPO4. After 4 h incubation, the hydrolysis capacity of chitosan- and Ca-alginate immobilized enzyme was calculated as 24% and 33%, respectively. It was reported that immobilized phytase from Lactarius piperatus effectively hydrolyzed the phytate in dry beans and it had the highest activity on wheat and peanuts at the end of 4 h at a rate of 75%.[Citation45] Also, after 8 h incubation, immobilized avocado phytase resulted in 65% reduction in phytate in soymilk.[Citation37]
Determination of reusability
The stability of immobilized phytase was examined by measuring the enzyme activity after each successive run. The chitosan-immobilized phytase conserved its original activity after 8 cycles. In the case of Ca-alginate, the activity began to fall after six cycles (Fig. 6 in SI). These results show that the immobilized phytases can be easily recovered and used repetitively. The reusability of the immobilized phytase is advantageous for the continuous use of the enzyme in industrial applications and also economical because of its significant reduction in operation costs in practical applications. It was previously reported that the phytase immobilized on Sepabead EC-EP retained 52% of its initial activity after 21 cycles of reuse at 37°C and 46% of its initial activity after 7 cycles of reuse at 60°C.[Citation37] Also, the immobilized Penicillium purpurogenu GE1 phytase maintained 100% activity for 12 successive batches and then gradually declined, reaching around 70% activity after 20 cycles.[Citation47]
Conclusion
In this paper, we immobilized the highly thermal- and pH-stabile phytase enzyme from a Geobacillus sp. for the first time. Uses of phytases have been gaining importance in human nutrition and health. The real application of phytase as animal feed additives was relied on three vital factors such as its thermal resistance, its proteolytic resistance, and its activity–pH dependency. In general, the mixture of phytase in feedstuff has to go through ∼1 min thermal pelleting treatment at 70–95°C.[Citation48] Also, the application of phytase as animal feed additives primarily counts on its stability over protease digestion and varying pH media conditions. Free phytase itself might be digested by proteases (pepsin or trypsin) present in the gastric fluid, leading to inactivation. In most poultry and livestock gastrointestinal tracts, the pH gradient of 2.0–9.0 occurred.[Citation49] The enzyme activity of immobilized Geobacillus sp. TF16 phytases in chitosan and Ca/alginate peaked at pH 5.0 and 3.0, respectively, and showed observable activity over a wide pH range. Also, it is highly thermostable at operational temperatures of 70–95°C. The capacity of dephytinization of soymilk and the high reusability of the immobilized phytases also make them very attractive for practical application and they could be used as additive material in the food industry.
LJFP_A_1261151_Supplementary_data.zip
Download Zip (27.2 KB)Acknowledgments
The authors thank assistant professor Dr. Kadriye INAN BEKTAS for providing the bacterium strain. The authors declare no conflicts of interest of any kind.
Funding
This work was supported by TUBITAK (project number 214Z190).
Supplemental data
Supplemental data for this article can be accessed on the publisher’s website.
Additional information
Funding
References
- Reddy, N.R; Sathe, S.K.; Salunkhe, D.K. Phytases in Legumes and Cereals. Advance in Food Research 1982, 28, 1–92.
- Wodzinski, R.J.; Ullah, A.H.J. Phytase. Advance in Applied Microbiololgy 1996, 42, 263–302.
- Bohn, L.; Meyer, A.; Rasmussen, S. Phytate: Impact on Environment and Human Nutrition. A Challenge for Molecular Breeding. Journal of Zhejiang University Science B 2008, 9, 165–191.
- Fugthong, A.; Boonyapakron, K.; Sornlek, W.; Tanapongpipat, S.; Eurwilaichitr, L.; Pootanakit, K. Biochemical Characterization and In Vitro Digestibility Assay of Eupenicillium parvum (BCC17694) Phytase Expressed in Pichia pastoris. Protein Expression and Purification 2010, 70, 60–67.
- Yao, M.Z.; Zhang, Y.H.; Lu, W.L.; Hu, M.Q.; Wang, W.; Liang, A.H. Phytases: Crystal Structures Protein Engineering and Potential Biotechnological Applications. Journal of Applied Microbiology 2011, 112, 1–14.
- Kumar, V.; Sinha, A.K.; Makkar, H.P.S.; De Boeck, G.; Becker, K. Phytate and Phytase in Fish Nutrition. Journal of Animal Physiology and Animal Nutrition 2012, 96, 335–364.
- Sharpley, A.N.; Chapra, S.C.; Wedepohl, R.; Sims, J.T.; Daniel, T.C.; Reddy, K.R. Managing Agricultural Phosphorus for Protection of Surface Waters: Issues and Options. Journal of Environmental Quality 1994, 23, 437–451.
- Martinez, C.; Ros, G.; Periago, M.J.; Lopez, G.; Ortuno, J.; Rincon, F. Phytic Acid in Human Nutrition. Food Science and Technology International 1996, 2, 201–209.
- Vohra, A.; Satyanarayana, T. Phytases: Microbial Sources Production Purification and Potential Biotechnological Applications. Critical Reviews in Biotechnology 2003, 23, 29–60.
- Da Silva, L.G.; Trugo, L.C.; Da Costa, T.S.; Couri, S. Low Phytate Lupin Flour Based Biomass Obtained by Fermentation with a Mutant of Aspergillus niger. Process Biochemistry 2005, 40, 951–954.
- Vohra, A.; Rastogi, S.K.; Satyanarayana, T. Amelioration in Growth and Phosphorus Assimilation of Poultry Birds Using Cell-Bound Phytase of Pichia anomala. World Journal of Microbiology and Biotechnology 2006, 22, 553–558.
- Yoo, G.Y.; Wang, X.J.; Choi, S.Y.; Han, K.; Kang, J.C.; Bai, S.C. Dietary Microbial Phytase Increased the Phosphorus Digestibility in Juvenile Korean Rockfish Sebastes schlegeli Fed Diets Containing Soybean Meal. Aquaculture 2005, 243, 315–322.
- El-Toukhy, N.M.K.; Youssef, A.S.; Mikhail, M.G.M. Isolation Purification and Characterization of Phytase from Bacillus subtilis MJA. African Journal of Biotechnology 2013, 12, 2957–2967.
- Mittal, A.; Singh, G.; Goyal, V.; Yadav, A.; Aggarwal, N.K. Production of Phytase by Acido-Thermophilic Strain of Klebsiella sp. DB-3FJ711774.1 Using Orange Peel Flour under Submerged Fermentation. Innovative Romanian Food Biotechnology 2012, 10, 18–27.
- Pandey, A.; Szakacs, G.; Soccol, C.R.; Rodriguez-Leon, J.A.; Soccol, V.T. Production Purification and Properties of Microbial Phytases. Bioresource Technology 2001, 77, 203–214.
- Kerovuo, J.; Tynkkynen, S. Expression of Bacillus subtilis Phytase in Lactobacillus plantarum 755. Letters in Applied Microbiology 2000, 30, 325–329.
- Oh, B.C.; Choi, W.C.; Park, S.; Kim, Y.O.; Oh, T.K. Biochemical Properties and Substrate Specificities of Alkaline and Histidine Acid Phytases. Applied Microbiology and Biotechnology 2004, 63, 362–372.
- Greiner R, Sajidan. Production of D-Myoinositol(12456)pentakisphosphate Using Alginate-Entrapped Recombinant Pantoea agglomerans Glucose-1-Phosphatase. Brazilian Archives of Biology and Technology 2008, 51, 235–246.
- Tischer, W.; Kasche, V. Immobilized Enzymes: Crystals or Carriers? Trends in Biotechnology 1999, 17, 326–335.
- Liang, Z.P.; Feng, Y.Q.; Meng, S.X.; Liang, Z.Y. Preparation and Properties of Urease Immobilized onto Glutaraldehyde Cross-Linked Chitosan Beads. Chinese Chemical Letters 2005, 16, 135–138.
- Tang, Z.X.; Qian, J.Q.; Shi, L.E. Characterizations of Immobilized Neutral Proteinase on Chitosan Nano-Particles. Process Biochemistry 2006, 41, 1193–1197.
- Yang, K.; Xu, N.S.; Su, W.W. Co-immobilized Enzymes in Magnetic Chitosan Beads for Improved Hydrolysis of Macromolecular Substrates under a Time-Varying Magnetic Field. Journal of Biotechnology 2010, 148, 119–127.
- Guisán, J.M.; Penzol, G.; Armisen, P.; Bastida, A.; Blanco, R.M.; Fernandez-Lafuente, R.; García-Junceda, E. Immobilization of Enzymes Acting on Macromolecular Substrates. In Immobilization of Enzymes and Cells; Bickerstaff, G.F.; Ed.; Humana Press: Totowa, 1997; 261–275.
- Fraser, J.E.; Bickerstaff, G.F. Entrapment of Enzymes and Cells in Calcium Alginate. In Immobilization of Enzymes and Cells. Bickerstaffr, G.F.; Ed.; Humana Press: Totowa, 1997; 61–66.
- Dokuzparmak, E.; Sirin, Y.; Cakmak, U.; Saglam Ertunga, N. Purification and Characterization of a Novel Thermostable Phytase from the Thermophilic Geobacillus sp. TF16. International Journal of Food Properties 2016, In press. doi:10.1080/10942912.2016.1203930.
- Abdel-Naby, M.A.; Hashem, A.M.; Esawy, M.A.; Abdel-Fattah, A.F. Immobilization of Bacillus subtilis a-Amylase and Characterization of Its Enzymatic Properties. Microbiolal Research 1998, 153, 319–325.
- Esawy, M.A.; Mahmoud, D.A.R.; Fattah, A.F.A. Immobilisation of Bacillus subtilis NRC33a Levansucrase and Some Studies on Its Properties. Brazilian Journal of Chemical Engineering 2008, 25, 237–246.
- Abdel-Naby, M.A.; Sherif, A.A.; EI-Tanash, A.B.; Mankarios, A.T. Immobilization of Aspergillus oryzae Tannase and Properties of the Immobilized Enzyme. Journal of Applied Microbiology 1999, 87, 108–114.
- Onem, H.; Nadaroglu, H. Preparation and Properties of Purified Phytase from Oakbug Milkcap (Lactarius quietus) Immobilised on Coated Chitosan with Iron Nano Particles and Investigation of Its Usability in Food Industry. Journal of Food and Nutrition Research 2014, 2, 938–945.
- Raghu, H.S.; Rajeshwara, N.A. Immobilization of α- Amylase (1, 4-α-D-Glucanglucanohydralase) by Calcium Alginate Encapsulation. International Food Research Journal 2015, 22, 869–871.
- Bradford, M.M. A Rapid and Sensitive Method for the Quantitation of Microgram Quantities of Protein Utilizing the Principle of Protein-Dye Binding. Analytical Biochemistry 1976, 72, 248–254.
- Garchow, B.G.; Jog, S.P.; Mehta, B.D.; Monosso, J.M.; Murthy, P.P. Alkaline Phytase from Lilium longiflorum: Purification and Structural Characterization. Protein Expression and Purification 2006, 46, 221–232.
- Yildirim, M.; Colak, A.; Col, M.; Canakci, S. A New Recombinant Phosphotriesterase Homology Protein from Geobacillus caldoxylosilyticus TK4: An Extremely Thermo- and pH-Stable Esterase. Process Biochemistry 2009, 44, 1366–1373.
- Tekincanli, M.A.; Yildirim Akatin, M.; Colak, A. Purification and Characterization of a Novel Thermostable Esterase from Thermus sp. NCCB 100425. Turkish Journal of Biochemistry 2015, 40, 116–124.
- Saglam Ertunga, N.; Turan, A.; Yildirim Akatin, M.; Keskin, S. Partial Purification and Characterization of Armillaria mellea ß-Glucosidase. International Journal of Food Properties 2013, 17, 678–689.
- Zhang, G.Q.; Dong, X.F.; Wang, Z.H.; Zhang, Q.; Wang, H.X.; Tong, J.M. Purification Characterization and Cloning of a Novel Phytase with Low pH Optimum and Strong Proteolysis Resistance from Aspergillus ficuum NTG-23. Bioresource Technology 2010, 101, 4125–4131.
- Bicak Celem, E.; Onal, S. Immobilization of Avocado Phytase on Epoxy-Activated Sepabead EC-EP and Its Application in Soymilk Phytate Hydrolysis. Artificial Cells, Blood Substitutes and Biotechnology 2009, 37, 195–202.
- Lata, S.; Rastogi, S.; Kapoor, A.; Imran, M. Immobilization of Phytase Produced by Fungal Strain Aspergillus heteromorphus MTCC 10685. Research Journal of Pharmaceutical, Biological and Chemical Sciences 2014, 5, 1615–1631.
- Dischinger, H.C.; Ullah, A.H.J. Immobilization of Aspergillus ficuum Phytase by Carbohydrate Moieties onto Cross-Linked Agarose. Annals of the New York Academy of Sciences 1992, 672, 583–587.
- In, M.J.; Kim, K.H.; Oh, N.S. Phytate Degradation by Immobilized Saccbaromyces cerevisiae Phytase in Soybean-Curd Whey. Biotechnology and Bioprocess Engineering 2007, 12, 348–353.
- Menezes-Blackburn, D.; Jorquera, M.; Gianfreda, L.; Rao, M.; Greiner, R.; Garrido, E.; Mora, M.L. Activity Stabilization of Aspergillus niger and Escherichia coli Phytases Immobilized on Allophanic Synthetic Compounds and Montmorillonite Nanoclays. Bioresource Technology 2011, 102, 9360–9367.
- Greiner, R.; Da Silva, L.G.; Couri, S. Purification and Characterisation of an Extracellular Phytase from Aspergillus niger 11T53A9. Brazilian Journal of Microbiology 2009, 40, 795–807.
- Greiner, R.; Konietzny, U.; Menezes-Blackburn, D.; Jorquera, M.A. Production of Partially Phosphorylated Myo-Inositol Phosphates Using Phytases Immobilised on Magnetic Nanoparticles. Bioresource Technology 2013, 142, 375–383.
- Liu, B.L.; Jong, C.H.; Tzeng, Y.M. Effect of Immobilization on pH and Thermal Stability of Aspergillus ficuum Phytase. Enzyme and Microbial Technology 1999, 25, 517–521.
- Onem, H.; Cicek, S.; Nadaroglu, H. Immobilization of a Thermostable Phytase from Pinar melkior (Lactarius piperatus) onto Magnetite Chitosan Nanoparticles. CyTA- Journal of Food 2016, 14, 74–83.
- Nannipieri, P.; Kandeler, E.; Ruggiero, P. Enzyme Activities and Microbiological and Biochemical Processes in Soil. In Enzymes in the Environment: Activity Ecology and Applications; Burns, R.G.; Dick, R.P.; Eds.; Marcel Dekker: New York, 2002; 1–33.
- Awad, G.E.A.; Esawy, M.A.; El-Gammal, E.W.; Ahmed, H.M.; Elnashar, M.M.; Atwa, N.A. Comparative Studies of Free and İmmobilized Phytase Produced by Penicillium purpurogenu GE1 Using Grafted Alginate/Carrageenan Beads. Egyptian Pharmaceutical Journal 2015, 14, 87–93.
- Lundblad, K.K.; Issa, S.; Hancock, J.D.; Behnke, K.C.; Mckinney, L.J.; Alavi, S.; Prestløkken, E.; Fledderus, J.; Sørensen, M. Effects of Steam Conditioning at Low and High Temperature Expander Conditioning and Extruder Processing Prior to Pelleting on Growth Performance and Nutrient Digestibility in Nursery Pigs and Broiler Chickens. Animal Feed Science and Technology 2011, 169, 208–217.
- Zhang, W.; Xu, F. Hierarchical Composites Promoting Immobilization and Stabilization of Phytase Via Transesterification/Silification of Modulated Alginate Hydrogels. ACS Sustainable Chemistry & Engineering 2015, 3, 2694–2703.