ABSTRACT
The effect of heat-treatment on foaming properties of ostrich albumen was investigated. At pH 7.5, coagulation of ovotransferrin (OT) resulted in minimum foaming capacity (FC) and maximum foam density (FD). While both exposure of sulfhydryl groups of ovalbumin (OV) and OT-OV interactions improved FC at lower pH, neither of them had beneficial effect on FC at pH 9. At pH 9, FC and FD were mildly affected by heat-treatment because of less aggregation of OT, then FC decreased due to covalent aggregates composed of ovomucin and OV, but at higher temperatures, higher level of OV unfolding resulted in enhanced FC.
Introduction
Heat treatment of food products is often required to ensure microbial safety. However, depending on the severity of the treatment and intrinsic factors such as composition and pH, significant protein denaturation may occur. This might result in considerable changes in the physicochemical properties of food commodities including functional characteristics such as foaming or gelling. For most applications in food industry, pasteurization of egg white is a prerequisite, because of possible contamination with pathogen Salmonella sp.[Citation1] Many studies have been therefore conducted to find out the influence of processing conditions on egg white proteins.[Citation2–Citation6]
Ostrich egg whites (OEW) are known as rich source of food proteins. Ostrich ovalbumin (OV) and ovotransferrin (OT) have higher molecular masses (approximately 58 and 75 kDa) compared with those of hen (approximately 45 and 70 kDa), indicating higher degree of glycosylation of the former. Ostrich OV showed the most acidic isoelectric point (approximately 3.98) among the OVs from all species. Lysozyme showed the highest concentration in hen egg white, while it was not found in ostrich albumen (egg white).[Citation7] Ostrich egg white has higher proportion of firm albumen than hen egg white, resulting in higher turbidity of OEW compared with that of hen eggs white.[Citation8] Ovomucin represented 2.8% of ostrich egg white’s proteins.[Citation9] Ovomucin is subdivided into three units; β-ovomucin with molecular weight of 523–720 kDa, α1-ovomucin with molecular weight of 135–150 kDa, and α2-ovomucin having molecular weight of 230.9 kDa. Ovomucin also has a great amount of disulphide groups.[Citation10,Citation11] Ostrich avidin exhibited the most acidic isoelectric point among all avian avidins examined.[Citation12]
Ostrich egg is the largest egg of any living bird, and one ostrich egg is equivalent to more than 20 chicken eggs,[Citation13] but there is not enough systemic information in literature about the influence of processing conditions on functional characteristics of OEW. Hence, the objective of the present study was to investigate the effect of heat treatment on foaming properties of OEW, attributed to physiochemical properties (sulfhydryl content and turbidity) and electrophoretic pattern of proteins, including sodium dodecyl sulfate polyacrylamide gel electrophoresis (SDS-PAGE) and native polyacrylamide gel electrophoresis (native-PAGE).
Materials and methods
Materials
Ostrich egg was obtained from a local supermarket. The egg white was carefully removed from the egg yolk and from chalaza. The chalaza free albumen was sieved through a 1 × 1-mm mesh, and thin albumen removed from the firm counterpart.[Citation8] A twofold dilution of thin albumen (50% v/v or 32 mg protein/ml) was prepared in 0.2 M Tris-HCl at pH 7.5, and 9. 5, 5ʹ-dithiobis (2-nitrobenzoic acid) (DTNB) was obtained from Aldrich. Reagent grade chemicals were used to prepare the following materials: Tris-glycine buffer (0.1 M Tris (hydroxymethyl)-aminomethane (Tris), 0.1 M glycine, and 4 mM ethylenediamine-tetra acetic acid disodium salt, pH 8.0) and Ellman’s reagent (4 mg/ml DTNB in Tris-glycine buffer).
Heat treatment
Ostrich egg white solutions (OEWS) were placed in test tubes (10 ml, 32 mg protein/ml and pH 7.5, 9) and placed in a water bath at a certain, constant temperature (in the range of 55–85°C) for 4 min. The required temperature was reached within 1 min of heating. After heating, the samples were immediately cooled in an ice water to stop further denaturation and stored at 4°C until analysis.
Determination of sulfhydryl content
The concentration of sulfhydryl (SH) groups of the OEWS was determined using Elman’s reagent (DTNB).[Citation14] The content of exposed (SH) groups was measured according to the method of van der Plancken et al.[Citation15]
Turbidity measurement
Heat treated samples (32 mg/ml) were diluted 1:1 (v/v) with demineralized water. The solutions were mixed and placed in cuvettes (light path length of 1 cm). The absorbance was measured at 590 nm using a spectrophotometer. (Bioquest, Cecil, United Kingdom). Sample blank was distilled water.[Citation16]
Gel electrophoresis (native PAGE, SDS-PAGE with and without 2-ME)
Sodium dodecyl sulfate polyacrylamide gel electrophoresis (SDS-PAGE) was run according to the method of Laemmi.[Citation17] The electrophoresis was done in both reducing and non-reducing conditions in the presence and absence of 2-mercaptoethanol (2-ME), respectively. Native PAGE without SDS and reducing agent was also performed according to method of Davis.[Citation18]
Determination of foaming properties
Foams were formed by whipping (4 minutes) the heated protein solutions in a household type mixer at ambient temperature. The rotational speed was 1100 rpm. Foaming properties were evaluated by foaming capacity (FC), foam density (FD), and foam stability (FS).
The volume of foam and liquid phase was measured in stoppered graduated cylinders. For the determination of FC, FD, and FS, the following formulae are used[Citation19]:
where FV is volume of foam, ILV is volume of the initial liquid phase, DV is volume of drainage, LVM is volume of the liquid phase at t = 60 min after foaming was finished, LVS is volume of the liquid phase at t = 30 s after foaming was finished, m100F is weight of 100 mL foam (g), and V100F is volume of 100 mL foam (mL).
Statistical analysis
A completely randomized design with three replications was used throughout the study. One-way analysis of variance (ANOVA) was carried out, and mean comparisons were run by Duncan’s multiple range tests to detect significance of differences among means. Confidence levels were set at 95% (p < 0.05). As a result, letters (a, b, c…) have been added on graphs to indicate significant differences between means (means with different letters differ significantly p < 0.05). The data were statistically analyzed by SAS software.
Results and discussion
Changes in sulfhydryl content
At both pH levels, the concentrations of SH groups was not affected by the heat treatment when the samples were heated at temperatures below 65°C (similar letters are shown in ). Nevertheless, at 65°C and above, the concentrations of exposed SH groups was increased significantly. In fact, the exposure of SH groups, hidden in the interior of the Ovalbumin (OV) molecules, was initiated at 65°C. However, denaturation of OV may have begun earlier than this temperature, yet the exposure of masked SH groups did not occur below 65°C. OV, the major protein in OEW, is the only egg white protein to contain (four) free sulfhydryl groups, which are buried in the protein core.[Citation7,Citation20] Heat-induced denaturation of OV results in exposure of these sulfhydryl groups.[Citation14] Thus, high amount of exposed SH groups at higher temperatures (indicated by (a) and (b) in ) indicated a high level of OV unfolding.
Gel electrophoresis
In the electrophoretic pattern of the unheated OEW at both pH values, four major protein bands were shown (–). Based on a previous report, these bands were ovomucin, OV, ovotransferrin (OT), and avidin, respectively.[Citation7,Citation9,Citation10,Citation12] When the intensity of the thermal treatment (until 85°C) was increased, bands corresponding to monomers faded without 2-ME (). On the contrary, the monomeric bands did not show any changes with 2-ME (), proving the key role of disulfide bridges in disappearance of these bands under non reducing conditions ( and ). The decline of monomeric band intensity was more pronounced at pH 9 compared to pH 7.5. On SDS-PAGE under non-reducing conditions, no changes in OT and OV bands were observed until heating up to 60°C ( and ). After heating at 65°C, a slight decrease in intensity of OV band was obtained, while a significant decrease in the intensity of OT band was observed. The monomeric bands of OT completely disappeared after heating at 70°C. The intensities of OV bands gradually decreased with increasing temperature. At 65°C, the sharp decline in OT band intensity coincided with the exposure of SH groups ( and ). This might indicate that thiol–disulfide exchanges between free SH groups of OV and disulfide bonds of OT occurred. At above 70°C, the interaction between OV and α-ovomucin was assumed by the disappearance of ovomucin band (). Both free SH groups of OV and disulfide bridges of ovomucin (has nine disulfide bridges) might be responsible for the interaction.
Figure 2. Effect of heat treatment on SDS-PAGE patterns of OEWS under non-reducing conditions (without 2-ME) at pH 9 (a) and pH 7.5 (b).
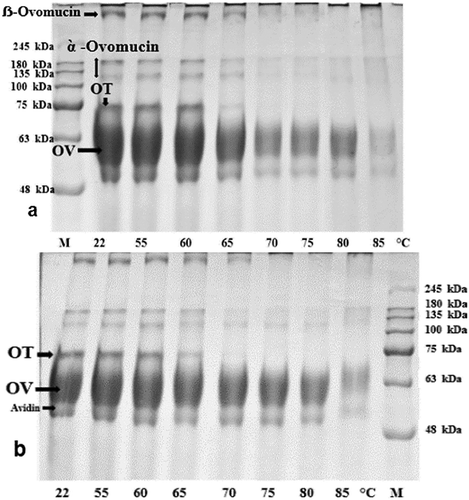
Figure 3. Effect of heat treatment on SDS-PAGE patterns of OEWS under reducing conditions (with 2-ME) at pH 9 (a) and pH 7.5 (b).
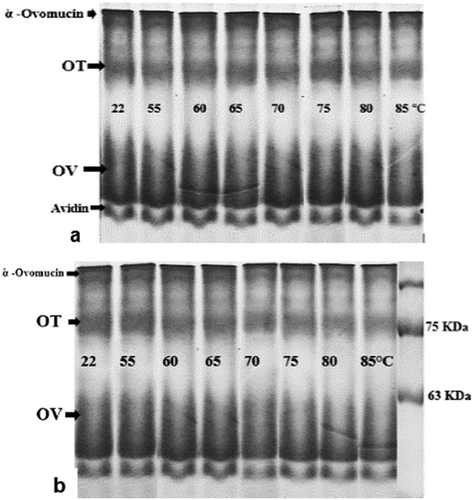
In the native page, no changes in the OT bands were observed until heating up to 55°C (), but at pH 7.5, OT bands disappeared after heating at 60°C (). At pH 9, OT band did not show any change, however (). The denaturation and aggregation of OT molecule caused OT band to disappear in the sample treated at pH 7.5 and 60°C. At pH 9, the OT may be unfolded, yet the aggregation of OT did not occur.
Changes in turbidity
Turbidity development of OEWS was monitored by the increase in absorbance 590 nm (A590), as shown in . Higher A590 was found in samples heated at higher temperatures, indicated by (a) in . Increase in A590 (or turbidity) was due to the formation of a large coagulum of heat denatured egg white protein molecules and the scattering effect of the coagulum. The differences in turbidity were most probably associated with the varying size and rate of protein aggregation.[Citation21] As shown above, the differences between the electrophoregrams obtained with and without 2-ME were more pronounced for samples treated at higher pH. These highlight that disulfide bonds play a more effective role in protein aggregation for OEWP at pH 9 than for OEWP at pH 7.5 (, b and 3a, b). In fact, smaller aggregates were formed by sulfhydryl oxidation at pH 9; however, at pH 7.5, heat treatment resulted in the formation of larger aggregates, which were only partly stabilized by disulfide bonds. Matsudomi et al. reported OT and OV interacted mutually during heating and then produced the soluble aggregates that were composed of OT and OV through disulfide linkage. The formation of such aggregates might inhibit the OT–OT interaction that led to the coagulation upon heating.[Citation22]
Relationship between heat-induced changes in the proteins and foaming properties of OEWP
Temperature and pH dependent changes could be observed in the foaming capacity (FC) of heat-treated OEWS (). At pH 7.5, FC was not affected by heat treatment when the samples heated at 55°C, indicated by same letter (a) in . After heating at 60°C, a marked decrease in the FC was exhibited (from 750% to 300% in ), indicated by change from (a) to (e) in the figure. A dramatic increase in FC of OEWS was observed after heating at 65 and 70°C (from 300% to approximately 700% in the table), shown by change from (e) to (b) and (a) in the figure. However, the FC of OEWS significantly decreased after heating above 80°C. At 60°C, the dramatic reduction in FC coincided with the disappearance of OT band in native PAGE (). Thus, this decrease in FC of OEWS at 60°C (pH 7.5) might be attributed to the coagulation of OT molecules. At pH 7.5, the marked increase in FC of OEWS heated at 65 and 70°C was associated with both exposure of SH groups and the decline in OT band intensity ( and ). The exposure of SH groups after heating above 65°C indicated conformational changes of OV molecules. Furthermore, masked SH groups of OV became free to interact with disulfide bonds of OT. Both thiol–disulfide exchange between OT and OV and some structural changes due to the exposure of SH groups may account for the enhanced FC of OEWP at 65 and 70°C. These results are in contrast to what was observed by Van der Plancken et al. who found that foaming ability of hen egg white (at pH 7.6) initially did not change with increasing temperature up to 65°C and further above 65°C (when the temperature of the second phase in the turbidity increase was reached) foaming ability of heat treated egg white solutions decreased until a minimum was reached at 75°C.[Citation4] The treatment time (20 minute) used by Van der Plancken et al. was much higher than the one used in the present study.[Citation4,Citation23] In the study by the authors, in spite of the fact that the exposure of SH groups of hen egg white initiated above 65°C, the large size aggregates, created under the condition, hindered the formation of voluminous foam. However, in our study, the time treatment was insufficient to form such deteriorating aggregates during heating from 65 to 75°C. For OEW treated at pH 7.5 and 85°C, the high level of OV unfolding was associated with damaged FC ( and ). Van der Plancken et al. found that extensive protein solubility loss at pH 7.6 (maximal at 85°C) did not impair the ability of the egg white proteins to form voluminous foam. They, however, indicated that the loss of soluble protein, able to unfold readily at the interface, was counterbalanced by the other structural changes induced by heat treatment.[Citation4,Citation23] Hagolle et al. clearly demonstrated that lysozyme showed no foaming ability unless it was heated above 80°C. After preheating to 85°C, the foaming behavior of lysozyme was considerably improved.[Citation3] However, in this study, heating at 85°C had detrimental effect on foam volume of the ostrich albumen, contrasting with observation regarding foam volume of hen albumen at the same temperature and pH.[Citation4] This difference between foaming behavior of ostrich and hen albumen at 85°C could be due to the fact that lysozyme was not found in the electrophoretic pattern of OEWP. Significant increase in foaming ability of lysozyme at 85°C probably did not allow foam ability of hen egg white to impair, but owing to the nonexistence of lysozyme in ostrich albumen, largest aggregates had damaging effect on FC of OEWP heated at 85°C. In fact, due to the lack of lysozyme in OEWS, the large aggregates, formed at above 80°C, did not allow large volumes of air to incorporate during the foam formation in this study. On the contrary, in the presence of lysozyme, hen egg white proteins readily unfolded at the interface without being hindered by large aggregates formed at 85°C.
Table 1. Foaming properties (average ± standard deviation) of OEWS after heat treatment.
Figure 6. Effect of heat treatment on foaming capacity of OEWS at pH 7.5 (empty symbols) and pH 9 (full symbols). For each individual pH, means with the same letter are not significantly different at p = 0.05.
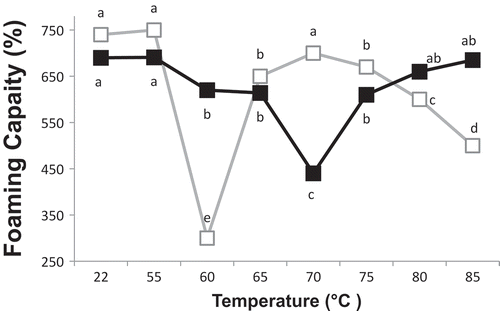
At pH 9, foam volume was only mildly affected by heat treatment up to 65 C. At 70°C, FC decreased substantially (from 630% to 440% in ), however. After heating at 75°C, heat-treated OEWP showed improved foam volume (from 440% to 640% in the table). At 60°C, a marked difference in FC of OEWP was observed between the two pH values. This could be attributed to the difference in aggregation of OT at two pH levels. After heating at 60°C, OT band (in native-PAGE) vanished for OEW with lower pH, while no change in the band was observed at high pH, which may prove that the coagulation of OT was negligible ( and ). Le Floch Fouere et al. stated that OT conformation in the interfacial film is different at pH 6.5 and pH 8.0.[Citation24] At pH 9, neither the exposure of SH groups nor the sulfhydryl–disulfide interaction between OT and OV had beneficial effect on FC of OEW after heating at 65 and 70°C, contrasting with FC of OEW at pH 7.5. The substantial decrease in FC of OEWP treated at 70°C (pH 9) was accompanied by the disappearance of ovomucin bands in SDS-PAGE without 2-ME (). The disappearance of α-ovomucin bands might indicate that disulfide bonding was contributed to the formation of aggregates having high molecular weight aggregates composed of α-ovomucin and OV ( and ). The covalent aggregates, formed through free SH groups of OV and disulfide bonds of α-ovomucin, might be partly responsible for the reduction in FC of OEWP treated at pH 9 and 70°C. Nevertheless, at 75°C, FC was improved, due in part to the higher level of OV unfolding. The observations regarding the foaming ability of hen egg white at pH 8.8 showed increasing temperature resulted in a decrease of the volume of the foams formed, this decrease in foam ability coincided with an increase in turbidity of heat treated egg white solutions, and the first phase in protein solubility loss. With increasing treatment temperature, foams with lower volume were formed until the temperature at which maximum turbidity occurs was reached. From this temperature on, the foaming ability of heat treated egg white solutions increased again. At pH 8.8, changes in protein structure were not accompanied by extensive protein insolubilization. This can explain the improved foaming ability of egg white proteins treated at temperatures above 75°C.[Citation4,Citation23] At pH 9, OEW showed no decrease in the volume of the foam after heating above 80°C, contrasting with FC of OEW at pH 7.5. Probably small aggregates, stabilized by oxidation of SH of OV, and facilitated the formation of voluminous foams at higher temperatures. However, OEW is a complex mixture of proteins, and many interactions and competitions between proteins can occur during heat treatment and foam formation, affecting volume of the foam.
On the other hand, at both pH values, foams with maximum FS invariable at all temperature levels were formed, indicated by similar letters (a) in . Still, for hen egg white at both pH values, foam stability was improved by heat treatment at temperatures above 55°C. Although a relatively strong correlation was observed between the foam stability and the sulfhydryl content of egg white solutions heat-treated at pH 7.6, the amount of exposed SH groups contributed negatively to the foam stability at pH 8.8. The heat-induced exposure of the buried SH groups of OV can facilitate the formation of disulfide bonds at the interface. Strong protein–protein interactions are necessary to form a stable foam. Increased aggregation had a positive influence on the foam stability.[Citation4] Nevertheless, the insignificant changes in the FS of OEWS indicted that heat induced changes in OT and OV molecules had no effect on the stability of the foams. In addition, no effect of large aggregates and free SH groups on FS of OEW was observed for both pH levels. The high stability of the foams could be related to the presence of ovomucin (α, β) in OEW. The difference between our result and the author’s result might be due to the higher concentration of protein in solutions we used. The amount of proteins in the hen egg white solution was 9.64 mg protein/ml,[Citation4] which was about three times less than the one we used (32 mg/ml).
Figure 7. Effect of heat treatment on foam density (♦) and foam stability (▲) of OEWS at pH 7.5 (empty symbols) and pH 9 (full symbols). For each individual pH, means with the same letter are not significantly different at p = 0.05.
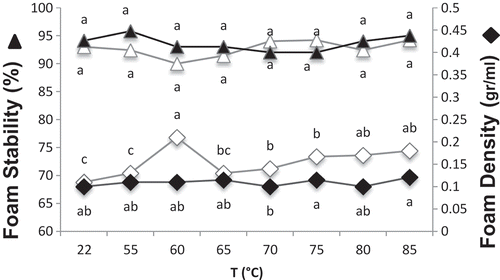
At pH 7.5, maximum foam density (FD) was obtained at the temperature (60°C) in which a minimum FC was observed, indicated by (e) in and () in . It may be hypothesized that after heating at 60°C, coagulation of OT facilitated formation of foam with maximum density (0/21 g/ml in ). As temperature increased from 60 to 65 and 70°C, the FD of OEW decreased. Not only the intermolecular interactions between OT and OV but also some structural changes of OV molecules resulted in the formation of foams with lower density. As discussed above, the denaturation of OV may have begun below 65°C, yet the exposure of masked SH groups occurred only after heating above 65°C. Thus, the structural changes of OV molecules due to the exposure of SH groups were possibly attributed to the reduction in FD. However, for hen albumen and OV foams (pH 7), changes in foam density were not observed at below 70°C.[Citation3,Citation4] At pH 9, FD was only mildly affected by heat treatment, which was attributed to the less aggregation and coagulation of proteins. At 60°C, a significant difference in FD of OEW at different pH values might be due to the fact that at pH 9, non-aggregated OT did not impair the incorporation of large volumes of the air, resulting in the formation of foam with lower density, as evidenced by the important difference in OT bands (in native-PAGE) at pH 7.5 and 9 ( and ). According to Le Floch Fouere et al., some unusual differences between the spectra of purified apo-OT at the air–water interface obtained at pH 6.5 and pH 8.0 suggest the rearrangement of interfacial water and changes in the protein conformation, especially at high concentration, while values of protein surface concentration and surface pressure were similar at these two pH values.[Citation24]
Conclusion
Heat treatment affected the foaming properties of OEWP. At pH 7.5, heat induced coagulation of OT resulted in minimum FC and maximum FD. Then, at 65 and 70°C, the exposure of SH groups of OV and its interaction with OT caused FC to improve. After heating above 80°C, the formation of large aggregates resulted in decreased FC. At pH 9, both FC and FD were only mildly affected by heat treatment, due to less aggregation of OT. Then, FC decreased considerably, due in part to the formation of covalent aggregates composed of ovomucin and OV. Further the higher level of OV unfolding improved foam volume. At both pH values, heat treatment had no effect on stability of the foams.
Acknowledgments
The authors are grateful to K. Khosraviani (Expert of Genetic and Biotechnology of Central Laboratory of College of Agriculture and Natural Resources, University of Tehran) for his assistance to performing gel electrophoresis.
Funding
This work has been funded by a grant provided by “The Research Council of the University of Tehran”.
Additional information
Funding
References
- Cunningham, F.E. Egg Product Pasteurization. In Egg Science and Technology; Stadelman, W.J.; Cotterill, O.J.; Eds.; Haworth Press: New York, 1995; pp. 289–321.
- Kato, A.; Takahashi, A.; Matsudomi, N.; Kobayashi, K. Determination of Foaming Properties of Proteins by Conductivity Measurements. Journal of Food Science 1983, 48, 62–65.
- Hagolle, N.; Relkin, P.; Popineau, Y.; Bertrand, D. Study of the Stability of Egg White Protein-Based Foams: Effect of Heating Protein Solution. Journal of the Science of Food and Agriculture 2000, 80, 1245–1252.
- Van der Plancken, I.; Van Loey, A.; Hendrickx, M. Foaming Properties of Egg White Proteins Affected by Heat or High Pressure Treatment. Journal of Food Engineering 2007, 78, 1410–1426.
- Katekhong, W.; Charoenrein, S. Color and Gelling Properties of Dried Egg White: Effect of Drying Methods and Storage Conditions. International Journal of Food Properties 2016. DOI:10.1080/10942912.2016.1233429.
- Uygun-Sarıbay, M.; Ergun, E.; Kalaycı, Y.; Koseoglu, T. Secondary Structure of Protein in Liquid, Frozen and Dried Egg-White Samples: Effect of Gamma Irradiation Treatment. International Journal of Food Properties 2016. DOI:10.1080/10942912.2016.1241263.
- Miguel, M.; Asuncon Manso, M.; Lopez Fandio, R. Comparative Study of Egg White Proteins from Different Species by Chromatographic and Electrophoretic Methods. Journal of European Food Research and Technology 2005, 221, 542–546.
- Fernandez Lopez, J.; Martinez, A.; Fernandez Gines, J.M.; Sayas Barbera, E.; Sendra, E.; Perez Alvarez, J.A. Gelling and Color Properties of Ostrich (Struthio camelus) Egg White. Journal of Food Quality 2006, 29, 171–173.
- Osuga, D.T.; Feeney, R. Biochemistry of the Egg-White Proteins of the Ratite Group. Archives of Biochemistry and Biophysics 1967, 124, 560–574.
- Itoh, T.; Miyazaki, J.; Sugawara, H.; Adachi, S. Studies on the Characterization of Ovomucin and Chalaza of the Hen’s Egg. Journal of Food Science 1987, 52, 1518–1521.
- Vadehra, D.V.; Nath, K.R. Eggs as Source of Protein. Critical Reviews in Food Science and Nutrition 1973, 4, 193–309.
- Hytonen, V.P.; Laitinen, O.H.; Grapputo, A.; Kettunen, A.; Savolainen, J.; Kalkkinen, N.; Marttila, A.T.; NordLund, A.R.; Nyholm, T.K.M.; Paganelli, G.; Kulomaa, M.S. Characterization of Poultry Egg-White Avidins and Their Potential as a Tool in Pretargeting Cancer Treatment. Biochemical Journal 2003, 372, 219–225.
- Shanawany, M.M. Ostrich Production Systems; FAO, Rome, Italy, 1999.
- Beveridge, T.; Arntfield, S. Heat Induced Changes in Sulphydryl Levels in Egg White. Journal of Dairy Science 1979, 12, 173–176.
- Van der Plancken, I.; Van Loey, A.; Hendrickx, M. Changes in Sulfhydryl Content of Egg White Proteins Due to Heat and Pressure Treatment. Journal of Agricultural and Food Chemistry 2005, 53, 5726–5733.
- Kitabatake, N.; Kinekawa, Y. Turbidity Measurement of Heated Egg Proteins Using a Microplate System. Food Chemistry 1995, 54(2), 201–203.
- LaemmLi, U.K. Cleavage of Structural Proteins during the Assembly of the Head of Bacteriophage. Nature 1970, 227, 680–685.
- Davis, B.J. Disc Electrophoresis. 2. Method and Application to Human Serum Proteins. Annual of New York Academy of Science 1968, 121, 404–427.
- Chang, Y.I.; Chen, T.C. Functional and Gel Characteristics of Liquid Whole Egg as Affected by pH Alteration. Journal of Food Engineering 2000, 45, 237–241.
- Powrie, W.D.; Nakai, S. The Chemistry of Eggs and Egg Products. In Egg Science and Technology; Stadelman, W.J.; Cotterill, O.J.; Eds.; AVI Publishing: Westport, 1968; pp. 97–139.
- Kaewmane, T.; Benjakul, S.; Visessanguan, W. Effect of Nacl on Thermal Aggregation of Egg White Proteins from Duck Egg. Food Chemistry 2011, 125, 706–712.
- Matsudomi, N.; Oka, H.; Sonoda, M. Inhibition against Heat Coagulation of Ovotransferrin by Ovalbumin in Relation to its Molecular Structure. Food Research International 2002, 35, 821–827.
- Van der Plancken, I.; Van Loey, A.; Hendrickx, M. Effect of Heat Treatment on the Physico-Chemical Properties of Egg White Proteins: A Kinetic Study. Journal of Food Engineering 2006, 75, 316–326.
- Le Floch Fouere, C.; Pezennec, S.; Pézolet, M.; Rioux Dube, J.F.; Renault, A. Beaufils, S. Unexpected Differences in the Behavior of Ovotransferrin at the Air–Water Interface at pH 6.5 and 8.0. Journal of Colloid and Interface Science 2011, 356, 614–623.