ABSTRACT
Terminalia arjuna (TA) encapsulated microcapsules were prepared using two different wall materials consisting of a combination of maltodextrin + gelatine (MDG) and maltodextrin + gum acacia (MDGA). Total phenolic, tannin, and flavonoids content of TA ethanolic extract were found to be 19.6 ± 0.7 g/100 g extract, 7.5 ± 0.7 g/100 g extract, and 2.7 ± 0.2 g/100 g extract, respectively. In vitro release of polyphenols increased with the time of stirring in simulated intestinal fluid (SIF; pH 6.8). The encapsulation efficiency (EE) and release of polyphenols (after 60 min) were observed to be 42 ± 4.g/100 g; 77.0 g/100 g; and 31.0 ± 0.5 g/100 g; 72.0 g/100 g, respectively for MDGA and MDG microcapsules. This study concluded that MDGA provided higher EE and stability than MDG for TA extract.
Introduction
Nutraceuticals can be defined as substances that are consumed as food or as part of a food and provide health benefits.[Citation1] Herbal nutraceuticals are commonly used by people who seek alternative health care. The term herb is used loosely to refer not only to herbaceous plants but also to bark, roots, leaves, seeds, flowers, and fruits of trees, shrubs, and woody vines and extracts that are valued for their savoury, aromatic, or medicinal qualities. Herbal remedies are also a part of renewed public interest in seeking health solutions through alternative approach to conventional medication. Herbal medicine is based on the concept that plants contain certain natural substances that can promote health and alleviate illness.[Citation2–Citation4] According to WHO, the estimated world production of herbal products was 62 billion US dollar, which is projected to grow to US $ 5 trillion by 2050.[Citation5]
Terminalia arjuna (TA) is an important medicinal plant used since ages by Indian traditional medicine to cure heart ailments. TA is chemically rich with its varied content of active compounds such as phytosterols, saponins, flavonoids (arjunone, arjunolone, luteolin), gallic acid, tannin, and many other useful polyphenols. The TA plant has been used as an antioxidant, antithrombotic, hypolipidemic, immunomodulatory, liver tonic, diuretic, hypocholesterolmic, anti-carcinogenic, and anti-inflammatory agent.[Citation6] However, the active compounds of TA extract are sensitive and may undergo degradation upon exposure to environmental conditions such as temperature, oxygen, light, and moisture. In addition, TA extracts also possess an unpleasant flavour, bitter/astringent taste, and abnormal red colour characteristics,[Citation7] which limit its applications as a functional bioactive ingredient in dairy and food products.
The development of novel dairy foods requires innovative technologies for incorporating health-promoting ingredients without reducing their bioavailability or functionality. Emulsion and/or encapsulation-based delivery systems are getting more attention nowadays due to their efficacy for targeted delivery and better absorption in the human gut.[Citation8] They offer significant advantages over the solids matrix materials as they can be used in liquid food systems. Microencapsulation or nanoemulsions were reported to provide the necessary protection for the bioactive compounds.[Citation9] However, the stability of these microcapsules or emulsions in the complex food environment has been a challenging work.
The common carrier materials used for encapsulation of the active components are maltodextrin (MD), acacia gums, semi-synthetic cellulose derivatives, and synthetic polymers.[Citation10] In the recent past, green tea polyphenols, silkworm purple oil, and ethyl butyrate were successfully encapsulated using suitable matrix materials viz. binary polymer,[Citation11] soybean protein isolate/β-cyclodextrin,[Citation12] and lactose/MD/WPI (whey protein isolate) matrix,[Citation13] respectively. Each carrier has advantages and disadvantages in terms of properties, cost, and encapsulation efficiency (EE). Several factors like nature and ratio of wall and core materials, their interactions, and the kind of encapsulation method employed may have considerable effect on the EE.[Citation12] Currently, MDs and soluble compound of modified starch derivatives are used alone or in combination with other materials to deliver plant extracts, aromatic additives, carotenoids, and vitamins in food products. However, the main drawback of using MD was reported to be a lack of emulsification capacity and lower retention of volatile compounds. In contrast, gum acacia (GA)[Citation14] and gelatine (G)[Citation15] are regarded as efficient encapsulating materials because of their high water solubility, comparatively low viscosity formation in solutions, and ability to form oil-in-water emulsions. The combination of GA and MD was reported to give better results and higher recovery percentage.[Citation16] In the light of this background, the present study was designed with an objective to assess the stability of TA microcapsules prepared by employing two different wall materials, i.e. combination of MD + G and MD + GA using light microscopy, EE, and in vitro release rate study.
Materials and methods
Materials
TA bark was obtained from the local market of Karnal, Haryana, India. MD (16.5–19.5 DE), acacia gum (gum arabic), G, catechin, gallic acid, and Folin–Ciocalteu reagents were purchased from Sigma Chemical Co. (St. Louis, MO, USA). All other reagents used in this study were procured from reputed chemical manufacturers and of analytical grade.
Preparation of ethanolic extract of TA
TA bark was cleaned properly and finely ground in an electric mill to obtain a coarse powder. TA extract was obtained from this powder by extracting it with alcohol (50 mL/100 mL water) at 5°C for 72 h. The extract was further filtered and the reddish brown syrupy mass thus obtained was finally dried in a tray drier at 50°C to obtain the final product. The yield of alcoholic extract was around 14.5 g/100 g and it was stored in amber-coloured bottles at 4°C until use.
Phytochemical and functionality measurement
Amount of total phenols (TPs) was measured using the Folin–Ciocalteu reagent procedure described by Chaovanalikit and Wrolstad.[Citation17] Quantity of TP was calculated using a calibration curve obtained using gallic acid (2.0–10.0 g/100 g, R2 = 0.9991). The results were expressed as gallic acid equivalents (GAE) per dry matter. Total flavonoids (TFs) were determined following the procedure by Dewanto and co-workers.[Citation18] Quantity of TF was calculated using a calibration curve obtained using catechin (0.2–1.0 g/100 g, R2 = 0.9914). The TF quantity was expressed as catechin equivalents per dry matter. The antioxidant capacity of the alcoholic extract of TA was measured using FRAP (ferric ion reducing antioxidant power, 0.1 mg/mL) assay described by Yen and co-workers.[Citation19] Free radical scavenging activity of the TA extract was measured using the DPPH (2,2-diphenyl-1-picrylhydrazyl, 0.4 mg/mL of methanol) radical assay.[Citation20] The tannin content of the extract was estimated according to the procedure described by Khomdram and co-workers.[Citation21] The calibration curve was prepared using tannic acid (6.0–12.0 g/100 g, RCitation2 = 0.9984) expressed as g 100 g−1 dry weight.
Preparation of microcapsules
MD at 8.0 g/100 mL was dispersed in distilled water and kept overnight in a water bath at 27°C to make an aqueous dispersion. Similarly, GA and gelatin (G) each at 2.0 g/100 mL were dispersed in distilled water (at 50°C for GA and at 60°C for G) separately to form the respective aqueous dispersion. Aqueous dispersions of MD and GA as well as MD and G were then mixed separately to obtain two different wall material solutions. These solutions were cooled to room temperature 2 h prior to encapsulation. The TA extract was dissolved into these wall material solutions with a magnetic stirrer at 2 g/100 mL. The mixtures were then emulsified in a high shear mixer (T25 IKA, Ultra-Turrax®, Germany) operating at 4000 rpm for 5 min. After mechanical homogenisation, the sample was dried in a tray dryer at 50°C to obtain the TA microcapsule powder. The powder was then stored in an amber-coloured glass bottle at 5°C until further use. Flow diagram depicting the encapsulation of TA extract is given in .
Microcapsule powder analysis
Encapsulation efficiency
The EE was determined by estimating polyphenols content in dried powder and in the emulsion mixture before drying. EE was expressed as percentage and calculated by using the following equation[Citation22]:
where SPC is the surface polyphenol content in the dried powder (after drying) and TPC is the total polyphenol content in the emulsion.
In vitro release rate study
In vitro release rate study of the TA extract from the prepared microcapsules was studied using simulated intestinal fluid (SIF). SIF was prepared by the method given in U.S. Pharmacopeia.[Citation23] In order to prepare the SIF, monobasic potassium phosphate (6.8 g) was dissolved in 250 mL of water. Then, 77 mL of 0.2 N sodium hydroxide and 500 mL of water was added followed by homogenous mixing of the contents. To this mixture 10.0 g of pancreatin was added and then the final volume was made up to 1000 mL using distilled water. Finally the pH of the resulting solution was adjusted to 6.8 ± 0.1 using 0.2 N sodium hydroxide and/or 0.2 N hydrochloric acid. The test conditions described by Liu and co-workers[Citation24] were followed to find out the in vitro release profile. About 5000 µg of TA microcapsules was placed in a glass beaker containing 90 mL phosphate buffer medium (pH 6.8) and the temperature was maintained at 37 ± 0.5°C. The sample was stirred using a stirrer adjusted at 50 rpm. After certain (30 min) intervals, 5 mL of the sample was withdrawn and the polyphenol content was determined by the method given by Chaovanalikit and Wrolstad.[Citation17]
Morphology
Solution of TA microcapsules powder (0.5 g/100 mL) was prepared in distilled water and then it was observed under a light microscope (Laibo, Bioplan H.L, 87240) to find out the morphology.
Statistical analysis
All the data were expressed as mean ± standard error mean (SEM). All the experiments were carried out three times to avoid practical errors. Significant differences among the samples were determined by one-way analysis of variance (ANOVA) using SYSTAT software version 6.0.1 (SYSTAT Software Inc., CA, USA). The means were tested for significance by adjusted Bonferroni post hoc test. Statistical significance was set at p < 0.05.
Results and discussion
Phytochemical screening
The TP, tannin, and flavonoid contents of ethanolic extract of TA were estimated to be 19.6 ± 0.7 g/100 g, 7.5 ± 0.7 g/100 g, and 2.65 ± 0.2 g/100 g, respectively (). The alcoholic extracts of herbs often contain higher amounts of phenolic and flavonoids due to their more polar nature.[Citation18] The results obtained in the present study were corroborated with the study of Patil and Gaikwad,[Citation25] who observed that the middle portion of TA bark consisted of 19.14 g of polyphenols per 100 g of extract, which was close to the results obtained in this study. However, the contents of tannin (7.5 ± 0.7 g/100 g) and flavonoids (2.65 ± 0.2 g/100 g) obtained in the alcoholic extract of TA were higher in the present study as compared to the results of Patil and Gaikwad.[Citation25]
Table 1. Contents of total phenolics (gallic acid equivalent), tannins, and flavonoids (catechin equivalents) in ethanolic extract of TA.
Antioxidant capacity
Antioxidant capacity or reducing potential of TA extract was determined using FRAP and DPPH methods. The reducing potential of different concentrations (0.05–0.2 g/100 mL) of ethanolic extract of TA measured using the FRAP method is presented in . The reducing potential of the TA extract increased significantly (p < 0.05) with increase in its concentration. Sultana and co-workers[Citation26] also found that with increase in the concentration of alcoholic extract of TA (2.5 mg/mL to 10.0 mg/mL), it’s reducing potential increased correspondingly. The reducing potential of the TA extract measured by DPPH radical scavenging activity also showed a significant (p < 0.05) increase in reducing activity with increase in its concentration from 0.01 to 0.09 g/100 mL (). This could be due to the increase in total polyphenol content (TPC) and total flavonoid content (TFC) in the TA extract as the concentration increased. Sultana and co-workers[Citation26] also observed higher correlation (r = 0.846) between TPC of TA extract and its reducing potential. Mety and Mathad[Citation27] advocated that the high amount of ascorbic acid content in TA also might be responsible for the antioxidant activity of TA.
Figure 2. Reducing potential TA extract using the FRAP method. Data are presented as means ± SEM (n = 3). Means in each bar with different superscripts (a, b, c, d, e) were significantly different (p < 0.05) from each other. Statistical comparisons were made between samples using ANOVA single factor.
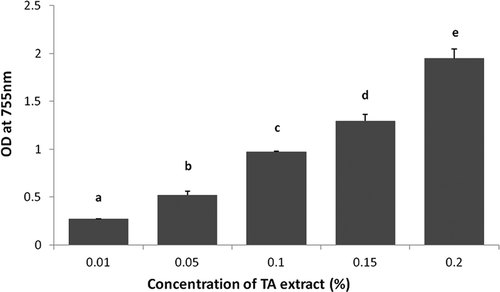
Figure 3. DPPH radical scavenging activity of various concentrations of TA extracts. Data are presented as means ± SEM (n = 3). Means in each bar with different superscripts (a, b, c, d and e) were significantly different (p < 0.05) from each other. Statistical comparisons were made between samples using ANOVA single factor.
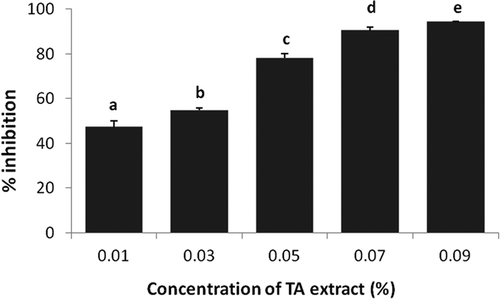
Microcapsule analysis
Two combinations of encapsulating materials, viz. MD (8.0 g/100 mL) + GA (2.0 g/100 mL) (MDGA) and MD (8.0 g/100 mL) + G (2.0 g/100 mL) (MDG), were used to encapsulate TA extract (0.5 g/100 mL). The light microscopic images of the encapsulated herb are illustrated in . It was found that the encapsulated herb had an irregular spherical shape. Some microcapsules were full with the core of the TA extract, but some were not completely full. However, all the TA microcapsules showed a smooth outer dark surface. MDGA combination obtained a better microcapsule core in terms of shape and size than MDG. The major limitations of MDs reported in the literature for use in encapsulation were lack of emulsifying capacity and low retention of volatile compounds.[Citation28] In the present study, G or GA were added along with MD to overcome these limitations. The formation of a smooth outer surface of microcapsules observed in the present study can probably be attributed to the addition of G or GA in the formulation, which could retain homo-dispersity. These results were in agreement with the work reported by Zheng and co-workers.[Citation29] The authors added lecithin (1.0 g/100 g) in edible ethyl cellulose to successfully develop a spherical and smooth-surfaced microcapsule of bayberry polyphenols.
Figure 4. Light microscopic images of (a) MDG microcapsule and (b) MDGA microcapsule containing TA extract.
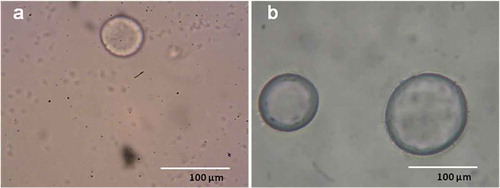
Determination of surface phenolic content of the capsule was needed in order to calculate the EE. Less quantity of phenolic content on the surface of the capsule shows more efficient encapsulation. The percent polyphenol retained by the encapsulated matrix was observed to be 42 ± 4.2 g/100 g and 31 ± 0.5 g/100 g in case of MDGA and MDG, respectively (). These results were supported by earlier studies of Cilek and co-workers[Citation30] and Malacridaa and Telisa[Citation31]. High EE, i.e. 97%, was obtained when gum arabic was mixed (at 2.0 g/100 mL) with MD for encapsulating phenolic extract from the sour cherry[Citation30] and 51–79% EE was observed when G was added (at 0.9–6.0 g/100 mL) to MD for encapsulating turmeric oleoresin.[Citation31] The MD influence on EE was less, probably due to a lack of emulsification and film-forming capacity. The gum arabic and G were added to the MD as they are known to perform the following functions during the encapsulation: they may lower the surface tension between the interfaces that was formed during the emulsification process; they may form a macromolecular layer surrounding the dispersed particles, which structurally stabilises the emulsions by reducing the rate of coalescence.
Figure 5. Encapsulation efficiency of TA microcapsules. Data are presented as means ± SEM (n = 3). Means in each bar with different superscripts (a, b) were significantly different (p < 0.05) from each other. Statistical comparisons were made between samples using ANOVA single factor.
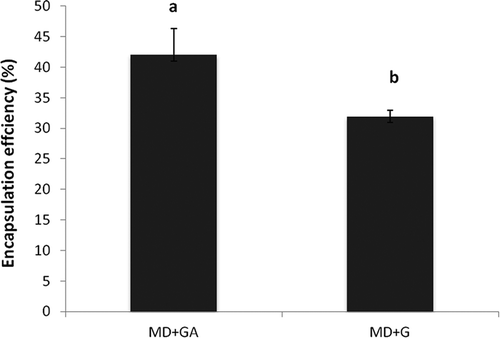
In the present study, the EE observed was less compared to other studies. It could be ascribed to the use of the tray drying process for encapsulation instead of the spray/freeze drying technique. It was also observed that the retention capacity of polyphenol was more in case of MDGA than MDG. This indicated that GA acted as a better emulsifier than G for encapsulation.
Release of active components
In vitro polyphenol release rate from TA microcapsule measured using SIF (pH 6.8) is depicted in . It was observed that as time of incubation (0, 30, 60, and 90 min) in SIF increased, the release of polyphenols from microcapsules was also increased significantly (p < 0.05) (~40.0, 49.0, 72.0, and 82.0 g/100 g in case of MDGA and ~70.0, 71.0, 77.0, and 82.0 g/100 g in case of MDG, respectively at 0, 30, 60, and 90 min intervals). Also significant (p < 0.05) difference in the release rate was observed between MDG and MDGA up to 60 min of incubation. Combination MDG had obtained higher release rates of polyphenols in comparison to combination MDGA. In case of MDG, the release of polyphenol was initially high (70.0 g/100 g) at 0 min and then with increase in time interval, the release of polyphenol was slowed down (71.0 g/100 g at 30 min, 77.35 g/100 g at 60 min, and 82.0 g/100 g at 90 min). Whereas in case of MDGA, the release rate of polyphenol was the lowest (40.0 g/100 g) at 0 min and then it increased dramatically further and finally reached 82.0% at 90 min of stirring.
Figure 6. In vitro release profile of polyphenolic content from TA microcapsule. Data are presented as means ± SEM (n = 3). Means in each bar with different superscripts (a, b) were significantly different (p < 0.05) between the samples. Means in each bar with different superscripts (A, B, C, D) were significantly different (p < 0.05) among the samples. Statistical comparisons were made between samples using ANOVA single factor.
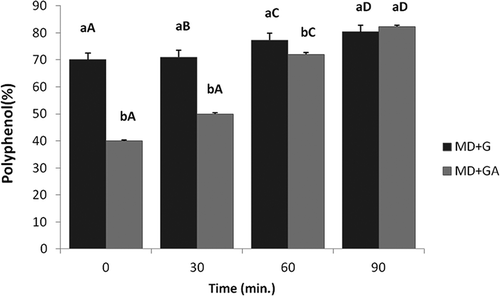
The results are in accordance with those observed by Zheng and co-workers,[Citation29] who observed that with increase in time of stirring (0, 20, and 40 min), the release rate of polyphenols increased (20.0, 40.0, and 90.0%) at pH 7 from ethyl cellulose microcapsules. Seok and co-workers[Citation32] found that most of the isoflavones microencapsulated by polyglycerolmonostearate were released in SIF at pH 7 and 8. This behaviour could be described by an increase in water interaction, wettability, and solubility of the microcapsules at the pH of the SIF.[Citation32] An improvement in wettability, due to the hydrophilic nature of gum arabic and G, was also observed by these authors. Sansone and co-workers[Citation33] stated that encapsulation not only improved the digestion stability but also led to effective absorption of the encapsulated phenolic compounds in the small intestine. In addition, the capsules provided an extra barrier and helped deliver the inner material in specific conditions at a specific time.
Conclusion
Results showed that the alcoholic extract of TA contained an appreciable amount of phytochemicals and also possesses good antioxidant activity. These phyto-constituents may act as a resource of pharmacologically active agents and natural antioxidants. Furthermore, TA extract encapsulation in MD and GA/gelatin matrix showed that it is possible to produce a proper emulsion using the discussed technique and also to obtain a dry product using the tray drying method. The light microscopy also showed that MD in combination with GA or gelatin was capable of forming a better smooth layer around TA polyphenol. In addition, the release rate study showed that the developed matrix materials, viz. MDG or MGGA, were capable of releasing the active constituents in SIF, indicating their better absorption efficiency in the small intestine. MDGA gave better EE and morphology than MDG. Thus, this study revealed that microencapsulation of TA using appropriate carrier materials and technique will facilitate the incorporation of TA into dairy and food products for making functional beverages while masking the undesirable flavour characteristics of herbs/herbal extracts.
LJFP_A_1280677_Supplementary_file.pdf
Download PDF (86.6 KB)Acknowledgement
The first author is thankful to the Director, ICAR-NDRI, for providing the necessary facilities and scholarship in the form Institute Fellowship to carry out the work smoothly.
Supplemental data
Supplemental data for this article can be accessed on the publisher’s website.
References
- Brower, V. Nutraceuticals: Poised for a Healthy Slice of the Healthcare Market? Nature Biotechnology 1998, 16, 728–732.
- Sawale, P.D.; Singh, R.R.B.; Arora, S. Stability and Quality of Herb (Pueraria tuberosa) – Milk Model. Journal of Food Science Technology 2015, 52(2), 1089–1095.
- Sawale, P.D.; Singh, R.R.B.; Kapila, S.; Arora, S.; Rastogi, S.; Rawat, A.K.S. Immunomodulatory and Antioxidative Potential of Herb (Pueraria tuberosa) in Mice Using Milk as the Carrier. International Journal of Dairy Technology 2013, 66(2), 202–206.
- Hussain, S.A.; Patil, G.R.; Reddi, S.; Yadav, V.; Pothuraju, R.; Singh, R.R.B.; Kapila, S. Aloe vera (Aloe barbadensis Miller) Supplemented Probiotic lassi Prevents Shigella Infiltration from Epithelial Barrier into Systemic Blood Flow in Mice Model. Microbial Pathogenesis 2017, 102, 143–147.
- Singh, H. Prospects and Challenges for Harnessing Opportunities in Medicinal Plants Sector in India. Law, Environmental Development Journal 2006, 2 (2), 196.
- Hussain, S.A.; Panjagari, N.R.; Singh, R.R.B.; Patil, G.R. Potential Herbs and Herbal Nutraceuticals: Food Applications and Their Interactions with Food Components. Critical Reviews in Food Science and Nutrition 2015, 55, 94–122.
- Sawale, P.D.; Pothuraju, R.; Hussain, S.A.; Kumar, A.; Kapila, S.; Patil, G.R. Hypolipidaemic and Anti‐Oxidative Potential of Encapsulated Herb (Terminalia arjuna) Added Vanilla Chocolate Milk in High Cholesterol Fed Rats. Journal of the Science of Food and Agriculture 2016, 96, 1380–1385.
- Dasgupta, N.; Ranjan, S.; Mundra, S.; Ramalingam, C.; Kumar, A. Fabrication of Food Grade Vitamin E Nanoemulsion by Low Energy Approach, Characterization and its Application. International Journal of Food Properties 2016, 19, 700–708.
- McClements, D.J.; Decker, E.A.; Park, Y.; Weiss, J. Structural Design Principles for Delivery of Bioactive Components in Nutraceuticals and Functional Foods. Critical Review of Food Science and Nutrition 2009, 49, 577–606.
- Williams, P.A.; Phillips, G.O. Gum Arabic. In Hanbook of Hydrocolloids; Phillips, G.O., Williams, P.A., Eds.; CRC Press: Boca Raton, FL, 2000; pp. 252–273.
- Belščak-Cvitanović, A.; Jurić, S.; Đorđević, V.; Barišić, L.; Komes, D.; Ježek, D.; Bugarski, B.; Nedović, V. Chemometric Evaluation of Binary Mixtures of Alginate and Polysaccharide Biopolymers as Carriers for Microencapsulation of Green Tea Polyphenols. International Journal of Food Properties, 2016. DOI:10.1080/10942912.2016.1225762.
- Liu T.; Pan W.; Zhang J.; and Wei, Z. Microencapsulation and Properties of the Silkworm Pupal Oil with Soybean Protein Isolate/β-Cyclodextrin. Journal of Chemical and Pharmaceutical Research 2014, 6(7), 295–301.
- Li, R.; Roos, Y. H.; Miao, S. Characterization of Mechanical and Encapsulation Properties of Lactose/Maltodextrin/WPI Matrix. Food Hydrocolloids 2017, 63, 149e159
- Patel, S.; Goyal, A. Applications of Natural Polymer Gum Arabic: A Review. International Journal of Food Properties 2015, 18, 986–998.
- Chandra, M.V.; Shamasundar, B.A. Texture Profile Analysis and Functional Properties of Gelatin from the Skin of Three Species of Fresh Water Fish. International Journal of Food Properties 2015, 18, 572–584.
- Chiou, D.; Langrish, T.A. Development and Characterisation of Novel Nutraceuticals with Spray Drying Technology. Journal of Food Engineering 2007, 82, 84–91.
- Chaovanalikit, A.; Wrolstad, R.E. Total anthocyanins and Total Phenolics of Fresh and Processed Cherries and Their Antioxidant Properties. Journal of Food Science 2004, 69, FCT67–FCT72.
- Dewanto, V.; Wu, X.; Adom, K.K.; Liu, R.H. Thermal Processing Enhances the Nutritional Value of Tomatoes by Increasing Total Antioxidant Activity. Journal of Agricultural Food Chemistry 2002, 50, 3010–3014.
- Yen, G.C.; Duh, P.D.; Chuang, D.Y. Antioxidant Activity of Anthraquinones and Anthrone. Food Chemistry 2000, 70, 307–315.
- Dudonné, S.; Vitrac, X.; Coutiere, P.; Woillez, M.; Mérillon, J.M. Comparative Study of Antioxidant Properties and Total Phenolic Content of 30 Plant Extracts of Industrial Interest Using DPPH, ABTS, FRAP, SOD, and ORAC Assays. Journal of Agricultural Food Chemistry 2009, 57, 1768–1774.
- Khomdram, S.D.; Salam, J.S.; Singh, P.K. Estimation of Nutritive Indices in Eight Lamiaceae Plants of Manipur. American Journal of Food Technology 2011, 6, 924–931.
- Luca, A.; Cilek, B.; Hasirci,V.; Sahin, S.; Sumnu G. Effect of Degritting of Phenolic Extract from Sour Cherry Pomace on Encapsulation Efficiency-Production of Nano-Suspension. Food and Bioprocess Technology 2013, 6, 2494–2502.
- U. S. Pharmacopeia. http://www.pharmacopeia.cn ( accessed August 5, 2016).
- Liu, S.Q.; Tong, Y.W.; Yang, Y.Y. Incorporation and In Vitro Release of Doxorubicin in Thermally Sensitive Micelles Made from Poly (N-Isopropylacrylamide-co-N, N-Dimethylacrylamide)-b-Poly (D, L-Lactide-co-Glycolide) with Varying Compositions. Biomaterials 2005, 26, 5064–5074.
- Patil, U.H.; Gaikwad, D.K. Pharmacognostical Evaluation of Stem Bark of Terminalia arjuna. International Journal of Pharmacy and Pharmaceutical Sciences 2011, 3, 98–102.
- Sultana, B.; Anwar, F.; Przybylski, R. Antioxidant Activity of Phenolic Components Present in Barks of Azadirachta indica, Terminalia arjuna, Acacia nilotica, and Eugenia jambolana Lam. Trees. Food Chemistry 2007, 104, 1106–1114.
- Mety, S.S.; Mathad, P. Antioxidative and Free Radical Scavenging Activities of Terminalia Species. International Research Journal of Biotechnology 2011, 2, 119–127.
- Reineccius, G.A. Flavor Encapsulation. Food Reviews International 1989, 5, 147–176.
- Zheng, L.; Ding, Z.; Zhang, M.; Sun, J. Microencapsulation of Bayberry Polyphenols by Ethyl Cellulose: Preparation and Characterization. Journal of Food Engineering 2011, 104, 89–95.
- Cilek, B.; Luca, A.; Hasirci, V.; Sahin, S.; Sumnu, G. Microencapsulation of Phenolic Compounds Extracted from Sour Cherry Pomace: Effect of Formulation, Ultrasonication Time and Core to Coating Ratio. European Food Research and Technology 2012, 235 (4), 587–586.
- Malacrida, C.R.; Telis, V.R.N. In Effect of Different Ratios of Maltodextrin/Gelatin and Ultrasound in the Microencapsulation Efficiency of Turmeric Oleoresin, Proceedings of the 11th International Congress on Engineering and Food, Athens, Greece, FPE915, 2011.
- Seok, J.S.; Kim, J.S.; Kwak, H.S. Microencapsulation of Water-Soluble Isoflavone and Physico-Chemical Property in Milk. Archives Pharmacal Research 2003, 26, 426–431.
- Sansone, F.; Picerno, P.; Mencherini, T.; Villecco, F.; D’ursi, A.M.; Aquino, R.P.; Lauro, M.R. Flavonoid Microparticles by Spray-Drying: Influence of Enhancers of the Dissolution Rate on Properties and Stability. Journal of Food Engineering 2011, 103, 188–196.