ABSTRACT
Starches isolated from eight oat cultivars were tested for their physicochemical, structural, and rheological properties. The isolated starches had low levels of ash and nitrogen contents with amylose ranging from 21.8 to 32.3 g/100 g. The amount of water released from starch gels decreased significantly (p < 0.05) with increase in freeze thaw cycle. The scanning electron micrographs revealed the presence of polygonal to irregularly shaped starch granules. All the starches exhibited A-type X-Ray diffraction pattern typically characteristic of cereal starches. The rheological properties of pastes were well described by the Herschel-Bulkley model at a shear rate of 0–100 s−1 (R2 > 0.99). The starch pastes behaved like a pseudoplastic fluid and exhibited shear thinning fluid characteristics with values of flow behaviour index considerably less than 1. Both the storage and loss moduli of the pastes increased sharply initially and then dropped after reaching the gelatinization peak. The magnitude of the dynamic rheological parameters varied significantly (p < 0.05) between the cultivars. All the starch pastes were highly elastic than viscous as evidenced by the lower tan δ values. Structure property relationships were established between starches using principal component analysis.
Introduction
Oats (Avena sativa L.) have been cultivated since ancient times for human consumption, animal feeding, and industrial use. There has been an increase in human consumption of oats owing to its high β-glucan content and antioxidant activity.[Citation1,Citation2] About 70 species of oats have been discovered around the world, and among them Avena sativa L. (hulled oat) and Avena nuda L. (naked oat) are the most cultivated species.[Citation3] Oats are rich in phenolic compounds, protein, dietary fibre, unsaturated fatty acids, and starch (~ 60%).[Citation4,Citation5] In comparison to wheat, oat grains have 2–5 times more lipids and are rich in hydrolytic enzymes.[Citation6]
Starch is the most abundant storage reserve carbohydrate in plants. It is mainly found in seeds, fruits, tubers, and roots and acts as a major source of energy during the period of dormancy and growth.[Citation7] Starches from different sources vary, particularly in their qualitative and quantitative make up as well as in some of the physicochemical and functional properties.[Citation8] Starch granules are synthesized and stored in terminally differentiated plastids known as amyloplasts within oat endosperms.[Citation9] Oat starch has higher bound lipid content, higher relative crystallinity, and smaller amylose chain length and granule size (6–10 µm).[Citation10,Citation11] In food processing, pasting and thermal properties of starch play an important role, specifically the amylose and amylopectin ratio.[Citation12] The increasing demand of high-quality oat as feed, food, and industrial raw material has led to the development of many different cultivars of oat. Though oat is not the most suitable cereal as a commercial source of starch, limited research suggests that oat starch possesses some unique chemical, physical, and structural properties over other commercially available starches.[Citation11] Starches from different oat cultivars have been found to differ in their physicochemical, thermal, and rheological properties. [Citation6,Citation10,Citation11,Citation13–Citation15] Oat starch utilization has been extensively investigated except in case of food applications.[Citation16] Oat starch can be used as a raw material to manufacture thin starch films.[Citation17] Oat starch in combination with oat hydrolysate or with xanthan gum has been used for thickening of sweet and sour sauces.[Citation18] The properties of starch pastes that play the important role in commercial application involve transparency, texture, viscosity, tendency to retrograde, and resistance to shear.[Citation18]
The science of rheology studies the flow and deformation of substances during food processing and handling. The method and intensity of various food processing operations such as cooking and mixing determine the structural, sensory, and functional properties of final starch-based products.[Citation19] Rheological characteristics are mostly based on stress (force per area) and strain (deformation per length). The relationship between stress and strain is analysed for loss modulus, complex shear modulus, and dynamic viscosity.[Citation20] Few models are used to explain the flow of fluids involved in food industries, such as linear (Newtonian or Bingham), power law (Ostwald-de-Waele), power law with yield stress (Herschel-Bulkley) and Casson models.[Citation21] For Non Newtonian fluids, power law model is most widely used to describe the flow properties of liquids in theoretical analysis as well as in practical engineering applications.[Citation22] The rheological properties of starch pastes or gels may be investigated as flow behaviour, viscoelasticity, creep, and gel strength.[Citation23,Citation24]
The significance of specific adjustment of rheological properties is in regulation of production processes and optimization of stability applicability and sensory properties of food product.[Citation25] Rheological properties determine the use of starch as gelling agent or thickeners.[Citation13] The most basic rheological characteristic of starch paste is viscosity, which changes upon applied shearing in a broad range. In many cases, viscosity of food products depends not only on shear rate but is also changing in time.[Citation13]
Flow behaviour of oat starches both under dynamic and shear conditions has not been the subject of the detailed study. Keeping this in mind, the present study was undertaken to investigate physicochemical, morphological, and rheological properties of starch from different oat cultivars for the selection of appropriate cultivar to see its suitability in different processes.
Materials and methods
Materials
Representative samples of eight Indian oat cultivars, OL-9, OL-10, Kent (PAU, Ludhiana.), OS-6, OS-7, OS-364, HFO-114 (CCS HAU, Hisar), and PLP-1 (CSK HPKV, Palampur), were procured. Seeds were cleaned for dirt, damaged seeds, and foreign material and stored at 20°C until further use. All the reagents used in the study were of analytical grade. Oat starch was isolated using the method of Hoover and Vasanthan with some modifications.[Citation15] Oat grains (500 g) were steeped in water (50°C) for 3 h and then ground in a laboratory blender at low speed for 5 min. The resultant slurry was then passed through a nylon cloth and centrifuged at 5000g for 15 min. The supernatant was discarded, and the sediment was suspended in excess 0.02 g/100 g NaOH. After standing for 1 h, the supernatant was removed. This procedure was repeated four times. The final sediment was suspended in distilled water and centrifuged in wide mouthed cups at 3000 rpm for 5 min. The upper non-white layer was scrapped off. The white layer was suspended in distilled water and centrifuged for 3–4 times. The resultant starch was collected and dried in an oven at 40°C for 12 h.
Physicochemical properties
The moisture, ash, and protein contents of oat starches were estimated using standard methods.[Citation26] The amylose content of oat starches was determined by the method of Williams et al. [Citation27] Swelling power and solubility was determined at 90°C using the method of Leach et al. [Citation28] Light transmittance of starch pastes was determined using method given by Craig et al. [Citation29] Freeze–thaw stability of starches was determined by method of Hoover & Ratnayake.[Citation30]
X-ray diffraction and morphological properties
The crystalline order in starches was determined using X-ray diffractometer (Shimadzu-7000, Japan) operated at 40 kV and 30 mA. The diffraction curves were obtained from 4° (2θ) to 30° (2θ) with the scanning speed of 5°/min. For measurement of morphological properties, starch suspension (1%) in ethanol was applied on an aluminium stub using double-sided adhesive tape. The sample was then coated with a thin gold palladium film using sputter coat (Ion sputter JFC-1100, JEOL, USA) and was observed in JSM-6100 Scanning Microscope (JEOL, USA) at an accelerating potential of 15 kV.
Rheological properties
A small amplitude oscillatory rheological measurement was made for starches with a Modular Compact Rheometer (Anton Paar, Austria) equipped with parallel plate system (4 cm diameter). The gap size was set at 1000 µm. The strain and frequency was set at 2% and 10 Hz, respectively, for all determinations. The dynamic rheological properties, such as storage modulus (G’), loss modulus (G’’), and loss factor (tan δ), were determined for starches. Starch suspension of 15% (w/w) concentration was loaded onto the ram of the rheometer and covered with a thin layer of low density silicon oil (to minimize evaporation losses). The starch samples were subjected to temperature sweep testing and were heated from 45–95°C at the rate of 2°C/min.[Citation31] For frequency sweep measurement, the starch slurry (15%) was prepared, manually stirred, and then heated at 85°C in a water bath followed by 3 min stirring. The sample was allowed to cool at room temperature and then loaded on the ram of rheometer. Frequency sweep tests from 0.1–100 rad/s were performed at 25°C. The storage modulus (G’), loss modulus (G’’), and loss tangent (tan δ) were derived at 25°C.
Steady shear properties were determined using the method described by Park et al with slight modifications.[Citation32] The starch suspension (10%) was sheared continuously from 1 to 1000s−1. To describe the variation in rheological properties of samples under steady shear, the data was fitted to the well-known Herschel-Bulkley (Eq 1) model:
σ=σ0+Κ.γn → (1)
Where σ is the shear stress (Pa), γ is the shear rate(s−1), Κ is the consistency index (Pa.sn), n is flow behaviour index (dimensionless), and σ0 is yield stress. TA rheometer Data Analysis software (version VI. 1.76) was used to obtain the experimental data and to calculate storage modulus (G’), loss modulus (G’’), and complex viscosity (ƞ*).
Statistical analysis
The data reported in all tables are average of triplicate observations. The data were subjected to statistical analysis using Minitab Statistical Software version. 13 (Minitab Inc., USA), and Pearson correlation coefficient between the properties analysed was established. Principal component analysis of measured properties was carried out to provide difference and similarities among different oat cultivars.
Results and discussion
Physicochemical properties
Differences in the starch physicochemical characteristics have significant impact on their functional and rheological behaviour, affecting their suitability for specific use.[Citation33] The isolated starches from different oat cultivars had moisture, ash, and nitrogen content in the ranges of 6.43 – 10.22, 0.11–0.81, and 0.02–0.5 g/100g, respectively (). Small amount of nitrogen in starches is due to lyophospholipids, the endosperm storage proteins that are located within the starch granules.[Citation10] Amylose content is responsible for number of factors that affect the swelling power, solubility, and gel forming properties of starch.[Citation34] Amylose content of oat starches was in the range of 21.8–32.3 g/100g agreeing with the previously reported values of 19–32.3 g/100g[Citation10] and 28.5–28.7 g/100g[Citation11] in oat starches. The lowest and the highest amylose content for OL-10 and PLP-1 were observed.
Table 1. Proximate composition, amylose content, swelling power, and solubility of starches from different oat cultivars.
Swelling power (SP) is used to study the bonds between the crystalline portions of starch. Starch granules with large crystalline area and strong bond swell less in cold water.[Citation35] SP of oat starches ranged between 13.1 and 19.1 g/g with the highest value shown by OS-7 cv. starch. Starch swelling occurs concomitantly with loss of birefringence and precedes solubilization.[Citation36] Solubility is used as an indicator of destruction within the starch components.[Citation35] Starch solubility was found to be the highest for HFO-114 (22.04 g/100g). Among all the cultivars, OL-9 cv. starch showed the lowest SP and solubility values. The low swelling power of starches may be attributed to the presence of a large number of crystallites formed by the association between long amylopectin chains.[Citation36] Ratnayke et al concluded that amylose content primarily influences solubility while amylopectin influences the SP.[Citation37] Lipid-amylose complexes, starch chains in amylopectin ratio, molecular weight, and relative distribution of amylose and amylopectin are reported to affect the SP and solubility of starch.[Citation38]
The Light transmittance value of starch pastes from different cultivars is presented in . All the starch pastes showed a decrease in light transmittance values with the increase in storage duration. The decrease in light transmittance can be attributed to the retrogradation of starches while storage.[Citation39] OS-346 cv. starch exhibited highest transmittance values, whereas OS-7 starch showed the lowest values at different duration of storage.
Freeze thaw stability
Freeze thaw stability of different oat starches after five freeze thaw cycles is presented in . Freeze thaw stability is an important property of starch in food industry as it helps to evaluate the ability of starch to withstand the physical changes that occur during freezing and thawing. The amount of water released on reassociation of starch chains during freeze thaw cycles determines the stability of starch gel.[Citation15] The amount of water released from starch gels decreased significantly with increase in freeze thaw cycle. OS-346 cultivar was significantly (p < 0.05) different from other cultivars in exhibiting higher freeze thaw stability after five freeze thaw cycles, whereas OS-7 showed least freeze thaw stability.
X- ray diffraction
X-ray diffractograms of starches from different oat cultivars are shown in . All the starches showed A-type X-ray diffraction pattern typically found in many cereal starches. In the diffraction spectra, there were strong diffraction peaks at 15 and 23° (2θ) and an unresolved doublet at 17 and 18° (2θ). All the starches showed similar diffraction patterns but varied in their values of diffraction intensity. The intensity of the major diffractions peaks was the highest in OS-6 cv. starch. Studies by Hoover et al.[Citation10] on Canadian oat starch reported that no peak was observed at 2θ of 20°, which is due to the presence of highly crystalline structure of amylose–lipid complexes.[Citation10] It has been proved, using solid-state NMR, that amorphous amylose–lipid complexes are present in native maize, rice, and oat starches.[Citation40]
Morphological properties
Scanning electron micrographs (SEM) of starches from different oat cultivars is shown in . SEM showed that the starch granules were irregular to polygonal in their shape. The surface of the granules appeared to be smooth with no evidence of pores. These results are in agreement with that reported earlier for Canadian oat starch.[Citation41] The starches separated from different oat cultivars differed significantly in their granule size which was determined by taking averages of 20 measurements. OS-7 cv. starch showed the presence of large size granules with granule length and width ranges of 4.2–11.3 µm and 3.9–11.3 µm, respectively. The smallest granule length (3.9–7.3 µm) and width (3.1–7.6 µm) was observed in OS-346 cv. starch. The variation in granule morphology may be attributed to climatic and agronomic conditions during processing. The complexity of starch biosynthesis results in natural variability in amylose and amylopectin molecules, which is reflected in diversity of granules.[Citation39]
Rheological properties
Steady shear properties
The plots of shear stress (σ) versus shear rate (γ) for starch pastes from different oat cultivars are depicted in . From the graph, it can be seen that with increase in shear rate, shear stress increased rapidly and then levelled off as shear rate further increased. This type of shear thinning behaviour is a typical characteristic of pseudoplastic fluids. The consistency index (K), flow behaviour (n) index, and the yield stress (σ0) were derived by fitting the flow curves by Herschel-Bulkley model (). The data fitted well with the model with high values of coefficients of determination (R2=0.999). The consistency and flow behaviour indices ranged from 0.06 to 3.08 Pa.sn and 0.59 to 0.94, respectively (). Starch paste from OS-6 cv. was significantly (p < 0.05) different from all other cultivars in exhibiting the highest K and the lowest n value, whereas OS-7 starch paste exhibited the lowest K and the highest n value. The higher K value indicates higher structural strength resulting in a less thixotropic behaviour.[Citation39] The n value, which indicates the extent of shear thinning behaviour as it deviates from 1, ranged from 0.59 to 0.94. The shear thinning behaviour can be explained by breaking of an entangled polysaccharide molecule network during shearing.[Citation32]Yield stress is the minimum stress required to initiate flow and indicates that there is a cross-linked or other interactive structure in a material that must be broken down before flow can occur at an appreciable rate.[Citation42,Citation43] All the investigated starch pastes were pseudoplastic fluids that differed in their values of Herschel-Bulkley yield stress. Starch paste from OS-6 cv. was significantly different from others in its lowest n value. This indicates its more shear thinning behaviour with high magnitude of yield stress and consistency coefficient. A decrease in viscosity with increase in shear rates irrespective of starch cultivars was observed.
Table 2. Herschel-Bulkley model parameters for starch pastes from different oat cultivars.
Figure 5. (a) Flow curves of starch pastes (10%) from different oat cultivars. (b) Changes in storage modulus (G′) and changes in Loss modulus (G′′) of 15% oat starch suspensions during heating: (A) OL-9 (B) OL-10 (C) Kent (D) OS-6 (E) OS-7 (F) OS-346 (G) HFO-114 (H) PLP-1. (c) Changes in storage modulus (G′) and changes in Loss modulus (G′′) of 15% oat starch suspensions during a frequency sweep (0.1–100 rad/s at 25ºC): (A) OL-9 (B) OL-10 (C) Kent (D) OS-6 (E) OS-7 (F) OS-346 (G) HFO-114 (H) PLP-1.
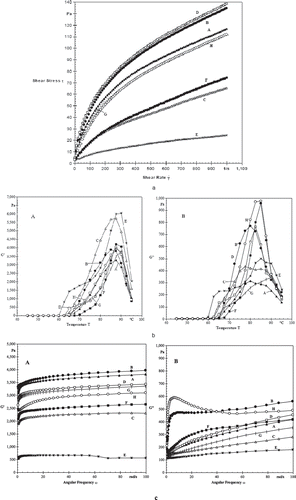
Dynamic shear properties
The data regarding dynamic shear properties of starch suspensions at temperatures ranging from 40°C to 95°C are shown in . It is well known that the effect of temperature on rheological properties needs to be documented because a wide range of temperatures is encountered during processing and storage of fluid food.[Citation44] The rheological properties of the starches measured using a dynamic rheometer showed significant variation in the peak G’ (Storage dynamic modulus, G’’ (Loss modulus) and peak tan δ values. G’ is the amount of energy stored in material and recovered from it per cycle while G’’ is the amount of energy dissipated or lost per cycle of sinusoidal deformation.[Citation45] The maximal G’ value showed considerable variation with the highest value of 5998 Pa for OS-7 and the lowest value of 3284 Pa for OL-9 cv., respectively. G’ and G’’ values of all starch suspensions increased to a maximum and then decreased with continuous heating (). G’ increased with increasing temperature due to swelling of starch granules which come into contact with each other and occupy greater portion of the suspension leading to higher system rigidity. The increase in G’’ can be accounted for leaching of amylose molecules from swollen granules and forming a continuous network and behaving in a viscous manner.[Citation39] The reduction in G’ and G’’ with further heating is the result of extended rupture and disintegration of starch granules and weakening of inter-chain interactions due to increase in molecular mobility and melting of crystallites.[Citation46] G’ was greater than G’’ in all starches showing their more elastic than viscous behaviour. The higher dynamic moduli (G’ and G’’) of starch may be attributed to its highest swelling power as viscoelasticity of gelatinized starch granules dispersion is governed by swelling behaviour of starch granules. tanδ which is ratio of G’’/G’ for all starches was less than 1. tan δ <1 indicates predominantly elastic behaviour, while a tan δ >1 indicates predominantly viscous behaviour. OL-9 cv. starch was significantly different from all other cultivars in its lowest G’, G’’, and tan δ values. The temperature (TG’max) at which the G’ of starch samples recorded the highest value ranged from 85.08°C to 90.08°C for OL-10 and HFO-114 cv., respectively. Breakdown in G’ is the difference between peak G’ at TG’max and the minimum G’ at 90°C.[Citation8] Maximum breakdown was observed in OL-10 cv., while it was lowest in OS-346 cv. starch. The difference in breakdown values among the oat starches may be attributed to the difference in morphological characteristics of starch granules and peak G’ value.
Table 3. Dynamic rheological properties of starches from different oat cultivars.
Frequency sweeps have been used widely to provide further insight into the structure of biomaterials.[Citation47] In the present study, the starch pastes were subjected to frequency sweep tests over a frequency range of 0–100 Hz to examine their viscoelastic nature (Fig. 5c). From the curves, it can be seen that with the increase in angular frequency (ω) there was increase in the values of G’ and G’’ while those of Complex viscosity (ƞ*) decreased showing a low frequency dependency. ƞ* is a measure of the overall resistance to flow. shows the values of G’, G’’, tan δ, and ƞ* values of starch pastes from different oat cultivars at 6.28 rad/s and at 25°C. The values of G’ were much greater than G’’ at all frequency values showing the strong elastic behaviour of starch samples. In case of an ideal elastic behaviour, G’ is expected to be independent of frequency and much higher than G’’ (G’≫ G’’).[Citation48] The value of tan δ were in the range of 0.04–0.22 (), indicating that all the samples were more elastic than viscous. The starch pastes also differed significantly in their complex viscosity values ranging from 338 to 549 Pa.s.
Table 4. Storage modulus (G’), loss modulus (G’’), complex viscosity (ƞ*), and tan δ for oat starch pastes at 6.28 rad/s at 25°C.
Principal component analysis
The measured properties of the starches from different oat cultivars were analysed by principal component analysis (PCA) to learn about similarities and differences of the samples. The results of PCA of starches are shown in and . The loading plot provided information about correlations between measured starch properties (). Amylose content was observed to be positively correlated with yield stress (r = 0.657, p < 0.05). A highly significant positive correlation of G’ with n (r = 0.661, p < 0.05) and a negative correlation with K (r = −0.670, p < 0.05) and ƞ* (r = −0.888, p < 0.05) was observed. G’’ showed a significant positive correlation with tan δ (r = 0.957, p < 0.05), whereas n showed a negative correlation with K (r = −0.865, p < 0.05) and ƞ*(r = −0.771, p < 0.05). K was observed to be positively correlated to ƞ*(r = 0.750, p < 0.05). The first principal component (PC1) and the second principal component (PC2) were described 40.1% and 20.5% of the variance, respectively. Together, the first two principal components represented 60.6% of the total variability. PCA showed that OS-7 cv. was located at far left of the score plot with a large negative score in PC1, and its starch was significantly different from all other cultivars in terms of its measured properties (). PLP-1 and Kent cv. had small negative score in PC1. All other cultivars were located at the right of score plot with a positive score in PC1.
Figure 6. (a) Principal component analysis: loading plot of first principal component (PC1) and second principal component (PC2) describing the variation among measured properties of starches from different oat cultivars. (b) Principal component analysis: score plot of PC1 and PC2 describing the overall variation among the properties of starches from different oat cultivars.
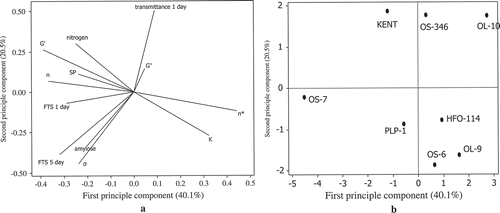
Conclusion
Significant differences among the physicochemical, morphological, and rheological properties of starches from eight oat cultivars were observed which could be attributed to the varietal differences. The starch granules were polygonal to irregular in shape and showed A-type X-ray diffraction pattern. All the starch pastes showed shear thinning behaviour, with typical characteristic of pseudoplastic fluid G’>G’’ in all starches showing their more elastic than viscous behaviour. There was increase to maximum in G’ and G’’ of starch suspensions and then decrease on continuous heating in all the oat cultivars. Correlation analysis data indicated a significant interdependence of physicochemical with structural and rheological properties of oat starches. OS-7 cv. starch was significantly different from all other cultivar in its measured properties as revealed by PCA.
Acknowledgement
The authors would also like to thank Mr Mohinder Singh (Punjab University, Chandigarh, India) for his technical assistance. The authors have declared no conflict of interest.
Funding
The author S Singh would like to acknowledge the UGC-UPE fellowship scheme for their financial support.
Additional information
Funding
References
- Peterson, D.M.; Emmons, C.L.; Hibbs, A.H. Phenolic Antioxidants and Antioxidant Activity in Pearling Fractions of oat Groats. Journal of Cereal Science 2001, 33(1), 97–103.
- Malkki, Y.; Myllymaki, O.; Teinila, K.; Koponen, S. Method for Preparing an Oat Product and a Foodstuff Enriched in the Content of Beta-Glucan. US Patent 2004, 6,797,307.
- Jing, P.; Hu, X.Z. Nutraceutical Properties and Health Benefits of Oats. In Cereals and Pulses: Nutraceutical Properties and Health Benefits; Yu, L.; Tsao, R.; Shahidi, F.; Eds.; Wiley-Blackwell: Oxford, UK, 2012; 221–240.
- Rasane, P.; Jha, A.; Sabikhi, L.; Kumar, A.; Unnikrishnan, V.S. Nutritional Advantages of Oats and Opportunities for Its Processing as Value Added Foods – A Review. Journal of Food Science and Technology 2015, 52(2), 662–675.
- Decker, E.A.; Rose, D.J.; Stewart, D. Processing of Oats and the Impact of Processing Operations on Nutrition and Health Benefits. British Journal of Nutrition 2014, 112, 58–64.
- Martinez O.M.; Whitney, K.; Reuhs, B.L.; Doehlert, A.C.; Simsek, S. Effect of Hydrothermal Treatment on Physicochemical and Digestibility Properties of Oat Starch. Food Research International 2013, 52, 17–25.
- Jobling, S. Improving Starch for Food and Industrial Applications. Current Opinion in Plant Biology 2004, 7, 210–218.
- Kaur, M.; Singh, N.; Sandhu, K.S.; Guraya, H. Physicochemical, Morphological, Thermal and Rheological Properties of Starches Prepared from Kernels of some Indian Mango Cultivars (Magnifera indica L.). Food Chemistry 2004, 85/1, 131–140.
- Yun, M.S.; Kawagoe, Y. Septum Formation in Amyloplasts Produces Compound Granules in the Rice Endosperm and Is Regulated by Plastid Division Proteins. Plant Cell Physiology 2010, 51, 1469–1479.
- Hoover, R.; Smith, C.; Zhou, Y.; Ratnayake, R.M.W.S. Physicochemical Properties of Canadian Oat Starches. Carbohydrate Polymers 2003, 52, 253–261.
- Mirmoghtadaie, L.; Kadivar, M.; Shahedi, M. Effects of Cross-Linking and Acetylation on Oat Starch Properties. Food Chemistry 2009, 116, 709–713.
- Jane, J.; Chen, Y.; Lee, L.F.; McPherson, A.E.; Wong, K.S.; Radosavljevic, M.; Kasemsuwan, T. Effect of Amylopectin Branch Chain Length and Amylose Content on the Gelatinization and Pasting Properties of Starch. Cereal Chemistry 1999, 76, 629–637.
- Berski, W.; Ptaszek, A.; Ptaszek, P.; Ziobro, R.; Kowalski, G.; Grzesik, M.; Achremowicz, B. Pasting and Rheological Properties of Oat Starch and Its Derivatives. Carbohydrate Polymers 2011, 83(2), 665–671.
- Gudmundsson, M.; Eliasson, A.C. Some Physicochemical Properties of Oat Starches Extracted from Varieties with Different oil Contents. Acta Agriculturae Scandinavica 1989, 39, 101.
- Hoover, R.; Vasanthan, T. Studies on Isolation and Characterization of Starch from Oat (Avena nuda) Grains. Carbohydrate Polymers 1992, 19, 285–297.
- Tian, B.Q.; Wang, C.; Wang, L.; Xie, B.J. Granule Size and Distribution of Raw and Germinated Oat Starch in Solid State and Ethanol Solution. International Journal of Food Properties 2016, 19, 709–719.
- Galdeano, M.C.; Mall, S.; Grossmann, M.V. Effects of Plasticizers on the Properties of Oat Starch Films. Materials Science and Engineering: C 2009, 29, 532–538.
- BeMiller, J.; Whistler, R. Starch: Chemistry and Technology; Academic Press: New York, 2009.
- Valetudie, J.C.; Gallant, D.J.; Bouchet, B.; Colonna, P.; Champ, M. Influence of Cooking Procedures on Structure and Biochemical Changes in Sweet Potato. Starch/Starke 1999, 51, 389–397.
- Mandala, I.G. Viscoelastic Properties of Starch and Non-Starch Thickeners in Simple Mixtures or Model Food. In Viscoelasticity: From Theory to Biological Applications; de Vicente, J., Ed.; InTech: England, 2012; 217–236.
- Marcotte, M.; Taherian, A.R.; Trigui, M.; Ramaswamy, H.S. Evaluation of Rheological Properties of Selected Salt Enriched Food Hydrocolloids. Journal of Food Engineering 2001, 48, 157–167.
- Barnes, H.A.; Hutton, J.F.; Walters, K. Viscosity. In An Introduction to Rheology; Elsevier Applied Science: New York, 1989; 11–35.
- Ahmed, J.; Ramaswamy, H.S.; Ayad, A.; Ali, I. Thermal and Dynamic Rheology of Insoluble Starch from Basmati Rice. Food Hydrocolloid 2008, 22, 278–287.
- Lawal, O.S.; Lapasin, R.; Bellich, B.; Olayiwola, T.O.; Cesàro, A.; Yoshimura, M. Rheology and Functional Properties of Starches Isolated from Five Improved Rice Varieties from West Africa. Food Hydrocolloids 2011, 25(7), 1785–1792.
- Yoo, D.; Kim, C.; Yoo, B. Steady and Dynamic Shear Rheology of Rice Starch-Galactomannan Mixtures. Starch/Starke 2005, 57, 310–318.
- AOAC. Official Methods of Analysis of the Association of Official Analytical Chemists; 2 vols. 15th ed. Washington, DC: AOAC, 1990.
- Williams, P.C.; Kuzina, F.D.; Hlynka, I. A Rapid Colorimetric Procedure for Estimating the Amylose Content of Starches and Flours. Cereal Chemistry 1970, 47, 411–420.
- Leach, H.W.; McCown, L.D.; Schoch, T.J. Structure of the Starch Granule I. Swelling and Solubility Patterns of Various Starches and Flours. Cereal Chemistry 1959, 36, 535–545.
- Craig, S.A.S.; Maningat, C.G.; Seib, P.A.; Hoseney, R.C. Starch Paste Clarity. Cereal Chemistry 1989, 66, 173–182.
- Hoover, R.; Ratnayake, W.S. Starch Characteristics of Black Bean, Chick Pea, Lentil, Navy Bean and Pinto Bean Cultivars Grown in Canada. Food Chemistry 2002, 78, 489–498.
- Sandhu, K.S.; Singh, N.; Kaur, M. Characteristics of the Different Corn Types and their Grain Fractions: Physicochemical, Thermal, Morphological and Rheological Properties of Starches. Journal of Food Engineering 2004, 64, 119–127.
- Park, S.; Chung, M.; Yoo, B. Effect of Octenyl succinylation on Rheological Properties of Corn Starch Pastes. Starch/Starke 2004, 56, 399–406.
- Kaur, M.; Sandhu, K.S.; Lim, S. Microstructure, Physicochemical Properties and In Vitro Digestibility of Different Indian Lentil (Lens culinaris) Cultivars. Carbohydrate polymer 2010, 79, 349–355.
- Wang, L.; Xie, B.; Shi, J.; Xue, S.; Deng, Q.; Wei, Y. Physicochemical Properties and Structure from Chinese Rice Cultivars. Food Hydrocolloids 2010, 24, 208–216.
- Reddy, C.K.; Haripriya, S.; Mohamed, A.N.; Suriya, M. Preparation and Characterization of Resistant Starch III from Elephant Foot Yam Amorphophallus paeonifolius) Starch. Food Chemistry 2014, 155, 38–44.
- Kaur, M.; Sandhu, K.S. In vitro Digestibility, Structural and Functional Properties of Starch from Pigeon Pea (Cajanus cajan) Cultivars Grown in India. Food Research International 2010, 43, 263–268.
- Ratnayke, W.S.; Hoover, R.; Warkentin, T. Pea Starch Composition, Structure and Properties: A Review. Starch/Starke 2002, 54, 217–234.
- Wani, I.A.; Sogi, D.S.; Wani, A.A.; Gill, B.S.; Shivhare, U.S. Physicochemical Properties of Starches from Indian Kidney Bean (Phaseolus vulgaris) Cultivars. International Journal of Food Science and Technology 2010, 45, 2176–2185.
- Wani, A.A.; Singh, P.; Shah, M.A.; Wani, I. A.; Gotz, A.; Schott, M.; Zacherl, C. Physicochemical, Thermal and Rheological Properties of Starches Isolated from Newly Released Rice Cultivars Grown in Indian Temperate Climate. LWT- Food Science and Technology 2013, 53, 176–183.
- Morrison, W.R.; Tester, R.F.; Snape, C.E.; Law, R.; Gidley, M.J. Swelling and Gelatinization of Cereal Starches. IV. Some Effects of Lipid-Complexed Amylose and Free Amylose in Waxy and Normal Barley Starches. Cereal Chemistry 1993, 70, 385.
- Hoover, R.; Senanayake, S.P.J.N. Composition and Physiochemical Properties of Oat Starch. Food Research International 1996, 29(1), 15–26.
- Achayuthakan, P.; Suphantharika, M.; Rao, M.A. Yield Stress Components of Waxy Corn Starch-Xanthan Mixtures. Effect of Xanthan Concentration and Different Starches. Carbohydrate Polymers 2006, 65, 469–478.
- Viturawong, Y.; Achayuthakan, P.; Suphantharika, M. Gelatinization and Rheological Properties of Rice Starch/Xanthan Mixtures: Effects of Molecular Weight of Xanthan and Different Salts. Food Chemistry 2008, 111, 106–114.
- Rao, M.A. Flow and Functional Models of Rheological Properties of Fluid Foods, in Rheology of Fluid and Semi-Solid Foods (Ed.M.A.Rao); Aspen Pub.: Maryland, 1999; 25–57.
- Stanley, D.W.; Stone, A.P.; Tung, M.A. Mechanical Properties of Food. In Handbook of Food Analysis, Volume I, Ch.4; Nollet, L.M.L.; Ed.; Marcel Dekker, Inc.: New York, 1996; 93–136.
- Wang, X.; Chen, L.; Li, X.; Xie, F.; Liu, H.; Yu, L. Thermal and Rheological Properties of Breadfruit Starch. Journal of Food Science 2011, 76, 55–61.
- Me´ndez-Montealvo, G.; Garcı´a-Sua´rez, F.J.; Paredes-Lo´pez, O.; Bello-Pe´rez, L.A.; Effect of Nixtamalization on Morphological and Rheological Characteristics of Maize Starch. Journal of Cereal Science 2008, 48, 420–425.
- Rao, M.A. Phase Transitions, Food Texture and Structure. In Texture in Food Volume I: Semi-Solid Foods; McKenna, B.M.; Eds.); Woodhead Publishing: Cambridge, UK, 2003; 36–62.