ABSTRACT
This work studied the potential of self-assembled micelles of casein–dextran conjugates (CD) as a protective carrier for bioactive curcumin. CD was prepared through the Amadori rearrangement of the Maillard reaction. In casein micelles and CD micelles, the addition of curcumin can quench the intrinsic fluorescence of casein gradually. According to the double logarithm equation, the binding constants were determined as 3.9 × 104 and 1.8 × 105 M−1 for the binding of curcumin with casein micelles and CD micelles, respectively. The higher affinity of hydrophobic binding of curcumin with CD micelles was ascribed to the more hydrophobic and compact structure of casein core surrounded by the hydrophilic dextran shell, which was supported by the fluorescence spectrum and fluorescence anisotropy of curcumin. Furthermore, CD micelles were found to be superior to casein micelles for improving the stability and radical scavenging activity of curcumin.
Introduction
Increasing attention has been given to natural curcumin owing to its low intrinsic toxicity but a wide range of biological and pharmacological activities such as antioxidant, antitumor, and anticancer properties.[Citation1–Citation3] Curcumin, a polyphenolic compound isolated from the rhizome of turmeric, has been widely used as food additives and traditional medicine for centuries. Chemically, curcumin is a diferuloylmethane molecule containing two ferulic acid residues linked by a methylene bridge. The beneficial activities of curcumin are related with hydroxyl groups of the benzene rings, double bonds in the alkene part, and the central β-diketone moiety.[Citation4] However, there are two major limitations in the application of curcumin as a routine treatment agent.[Citation5] Firstly, curcumin is practically insoluble in water at acidic and neutral pH values, which significantly reduces its availability in biological systems. Secondly, curcumin is stable at acidic pHs but unstable at neutral and basic pHs, under which conditions curcumin breaks down readily to trans-6-(4′-hydroxy-3′-methoxyphenyl)-2,4-dioxo-5-hexanal, vanillin, ferulic acid, and feruloyl methane.[Citation6,Citation7]
Extensive studies have shown that curcumin can hydrophobically bind with proteins including milk casein,[Citation8–Citation10] β-lactoglobulin,[Citation11] and soy protein,[Citation12,Citation13] which may greatly improve the solubility, stability, and bioactivities of curcumin. However, proteins tend to undergo obvious aggregations at pH values around their isoelectric point, when the ionic strength is above a certain level, and when the temperature exceeds the thermal denaturation temperature. Thus, it is difficult to control the stability, release, and bioavailability of protein-encapsulated bioactive compounds in several environmental conditions. Dickinson et al.[Citation14] and McClements et al.[Citation15,Citation16] previously found that the formation of protein/polysaccharide complexes and their particles can let proteins have improved resistance to aggregation/dissociation over wide ranges of pH, salt, and temperature. Our recent work further demonstrated that bovine serum albumin/carrageenan complexes and bovine serum albumin/carrageenan particles may successfully encapsulate polyphenolic compounds with higher binding affinity and additional benefits compared to native protein.[Citation17,Citation18] Nevertheless, these approaches based on electrostatic protein/polysaccharide complexes are still sensitive to environmental conditions due to the employment of noncovalent interactions.
Maillard reaction is a nontoxic natural reaction, which conjugates proteins and polysaccharides by linking the carbonyl group of polysaccharides to the unprotonated amino group of proteins under controlled conditions. Different proteins such as casein,[Citation19–Citation21] lysozyme,[Citation22] bovine serum albumin,[Citation23,Citation24] and soybean protein[Citation25] were reported to conjugate with polysaccharides, giving significantly increased solubility, stability, and other functional properties such as emulsifying ability. Protein–polysaccharide conjugates can self-assemble into micelles with a hydrophobic protein core and a hydrophilic polysaccharide shell. The micelles of protein–polysaccharide conjugates were previously used to encapsulate hydrophobic compounds such as pyrene,[Citation19] β-carotene,[Citation26] and tea catechin.[Citation21,Citation24,Citation27] However, few work has been reported to use protein–polysaccharide conjugate micelles as the carrier for hydrophobic curcumin so far.
The present work was intended to elucidate the binding behaviours of curcumin with the micelles of casein–dextran conjugates (CD) and the implications on the stability and antioxidant activity of curcumin. We prepared CD through the Amadori rearrangement of Maillard reaction and subsequently characterized the properties of CD micelles including critical micelle concentration (CMC), particle size, and zeta potential. The turbidity measurement revealed that CD micelles had higher stability than casein micelles in the pH range of 2–8. The binding constants of curcumin with casein micelles and CD micelles were obtained through curcumin-induced fluorescence quenching of casein. The binding mechanism of curcumin with casein micelles and CD micelles was further studied by measuring the fluorescence spectrum and fluorescence anisotropy of curcumin. The stability and the radical scavenging activity of free and encapsulated curcumin were also investigated.
Materials and methods
Materials
Curcumin, casein, 2,2’-azinobis(3-ethylbenzothiazoline-6-sulfonic acid) (ABTS), and pyrene were purchased from Sigma-Aldrich Chemical Co. Dextran with molecular weight of 10 kDa was obtained from Sino-pharm Chemical Reagent Co. o-Phthaldialdehyde (OPA) was purchased from Aladdin Chemical Co. All other chemicals used were of analytical reagent grade and water was double distilled.
Synthesis of casein–dextran conjugates
The Maillard reaction was used to conjugate casein with dextran by linking the amino group in the former to the reducing end carbonyl group in the latter.[Citation19] Specifically, casein was dissolved in double-distilled water and was adjusted to pH 7 with 1.0 M sodium hydroxide, and then dextran solution was added dropwise under gentle stirring. The molar ratio of casein to dextran in the mixture was 4:1. The mixed solution was lyophilized for 3 days at −50°C. The freeze-dried powder was heated at 60°C for 20 h at a relative humidity of 79% in a desiccator containing saturated potassium bromide solution. The resultant product of CD was stored at −20°C before use.
Conjugation degree measurement
The conjugation degree of CD was analysed through an o-phthaldialdehyde (OPA) assay, which was expressed as the decrease in free amino groups of casein after Maillard reaction. The OPA reagent was prepared according to Goodno et al.[Citation28] The OPA reagent was mixed with CD for 2 min at room temperature. Then, the absorbance of the mixture was measured at 340 nm immediately using a Shimadzu UV-1800 spectrophotometer at 25°C. The conjugation degree of CD was calculated from the loss in amino groups compared to unreacted control. In the following comparative measurements, the protein concentrations are same in the prepared casein and CD samples, which is further determined by the Bradford method using bovine serum albumin as a standard.
Particle size and zeta potential measurements
The particle size and zeta potential measurements were carried out on a Malvern Zetasizer Nano ZS (Malvern Instruments, London, England) at 25°C. The particle size was controlled in situ by dynamic light scattering at a fixed scattering angle of 173°. The zeta potential was obtained by the Dispersion Technology software according to Smoluchowski approximation in an automatic mode. During the measurements, the samples were diluted 10 times with pH 7 sodium phosphate buffer for analysis and each value was measured at least three times.
Turbidity measurement
Turbidity measurement was carried out in order to compare the stability of casein micelles and CD micelles with a Shimadzu UV1800 spectrometer at 25°C. The turbidity of samples was monitored by the absorbance at 450 nm in the pH range of 2–8. A cuvette with 1 cm pathway was used.
Steady-state fluorescence measurements
Steady-state fluorescence experiments were performed with a Hitachi F-4500 FL Spectrophotometer at 25°C. The values of CMC of casein and CD were determined from measurement of pyrene polarity index (I1/I3). I1/I3 is the ratio of the intensities of first and third vibronic peaks in the fluorescence emission spectrum of pyrene. The emission spectra of pyrene mixed with different concentrations of casein and CD were measured in the range of 350–600 nm with the excitation wavelength at 335 nm.
Intrinsic fluorescence of casein was measured for the samples of casein micelles and CD micelles. The emission spectra of casein were taken from 300 to 450 nm with an excitation wavelength of 290 nm. In the determination of binding constants of curcumin with casein micelles and CD micelles, titrations were conducted by increasing curcumin concentration. The data recorded at maximum fluorescence of casein were used to estimate the binding constant according to the double logarithm equation.[Citation29] Curcumin is poorly soluble in water so it was dissolved in ethanol at 1 g/L as stock. A small quantity of curcumin stock solution was added to water, casein micelles, and CD micelles. The obtained curcumin samples were clear solutions without curcumin precipitates, even after centrifugation at 12,000 rpm. The fluorescence spectra of curcumin in water, casein micelles, and CD micelles were taken from 460 to 650 nm with the excitation wavelength at 424 nm.
Fluorescence polarization technique
A PerkinElmer LS55 Fluorescence Spectrometer with parallel and perpendicular polarizers was used to determine the fluorescence anisotropy (r) of curcumin in water, casein micelles, and CD micelles at pH 7. Curcumin was excited at 424 nm, and the emission spectra were scanned from 460 to 650 nm. The fluorescence intensities were obtained at 0–0°, 0–90°, 90–0°, and 90–90° angle settings of the excitation and emission polarization accessories at 25°C. The value of r was calculated according as[Citation30]
where and
are the polarized fluorescence intensities in the directions parallel and perpendicular, respectively, and G is the grating correction factor, which is the ratio of sensitivities of the instrument for vertically and horizontally polarized light.
UV−Vis absorption measurement
The absorption spectra of 10 µM curcumin in water, casein micelles, and CD micelles were measured on Shimadzu UV-18000 spectrophotometer at 25°C. The maximum absorption of curcumin was recorded at different time intervals within 360 min to test the stability of curcumin in pH 7 at 25°C.
Antioxidant activity of curcumin
The ABTS radical cation decolourization test was used in the assessment of antioxidant activity of 10 µM curcumin.[Citation31] Briefly, ABTS+ solution was prepared by mixing 7.4 mM ABTS with 2.6 mM potassium persulfate and kept in the dark at least for 12 h. The obtained ABTS+ solution was diluted to an absorbance of 0.7 ± 0.02 at 734 nm, and mixed with casein micelles and CD micelles with or without curcumin, respectively. The absorbance spectrum of ABTS+ in each sample was measured by Shimadzu UV-1800 spectrophotometer at 25°C.
Results and discussion
Formation of CD micelles
Maillard reaction gives different products in three reaction stages (early, intermediate, and final stages), which is dependent upon the reaction conditions such as pH, time, temperature, relative humidity, and concentration and type of reactants.[Citation19,Citation20] We controlled Maillard reaction in its early stage to obtain the Amadori rearrangement product mainly [Citation19,Citation32]; that is, no yellow colour occurred in the product of CD. gives a schematic diagram of Maillard reaction between casein and dextran. Casein is a mixture of αs1-, αs2-, β-, and κ-casein fractions in the weight ratio of 3:0.8:3:1, corresponding to 14, 24, 11, and 9 lysine residues in each casein fraction, respectively. According to the data of molecular weight of lysine and casein fraction, an average lysine residue number in each casein is estimated as 13. In addition to other terminus amino group, there is average number of 14 amino groups that can react with the reducing end carbonyl group of dextran in each casein. Through the calculation of the average number of free amino groups before and after Maillard reaction, OPA analysis proves that CD has been formed via the Maillard reaction. The conjugation degree is about 27% in CD; that is, about 10.2 free amino groups are retained in casein after the Maillard reaction, whereas about 3.8 dextran has been conjugated to one casein on average.
Figure 1. The schematic diagram of Maillard reaction of casein with dextran and possible structure of CD micelles.
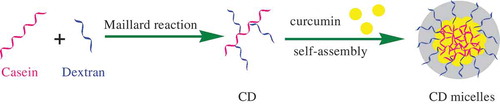
Casein molecules exhibit a strong tendency to self-assemble into casein micelles mainly through the hydrophobic interaction between the hydrophobic portions of casein molecules. As shown in , CD may also self-assemble into micelles but with the special core-shell structure, where hydrophobic protein parts of CD aggregate with each other to form protein core and the shell is composed of the conjugated hydrophilic dextran segments. The micellization of casein and CD is comparatively monitored in pH 7 sodium phosphate buffer using fluorescence probe of pyrene. As shown in , the plots of pyrene polarity index (I1/I3) of casein and CD both decrease gradually with increasing concentration of casein (Ccasein) and level off at high Ccasein values. The values of CMC of casein and CD are determined to be 1.4 and 1.1 g/L, respectively, corresponding to the interceptions of two furnished straight lines in the curves of I1/I3 vs. Ccasein (an example given in ). When Ccasein is above their CMC values, CD micelles often have clearly smaller I1/I3 values than casein micelles, which suggests a more hydrophobic protein core inside CD micelles. On the analysis of surface hydrophobicity of casein using fluorescence technique, Esmaili et al. found that more hydrophobic groups of casein are exposed during heating.[Citation9] Because there is heat treatment in Maillard reaction, it is reasonable to expect that CD with more exposed hydrophobic groups have greater tendency to aggregate relatively tightly into CD micelles. Furthermore, the hydrophilic segments in dextran shell around casein core can form hydrogen bonds with water and hence reduce the unfavourable contacts between water and hydrophobic groups of casein, as well as the Coulombic repulsion between the charged groups of casein.[Citation33] Accordingly, compared to casein micelles, CD micelles are formed with a smaller CMC value and more hydrophobic structure of protein core.
At selected Ccasein = 2 g/L above their CMC values, casein micelles and CD micelles have the average diameters of 78.1 ± 0.7 and 75.3 ± 0.6 nm, respectively. As reported previously,[Citation32] the conjugation of protein with polysaccharide can stabilize a larger surface area of micelles by the hydrophilic moiety of polysaccharide. As a result, casein conjugated with hydrophilic dextran favours the formation of more compact protein core and leads to slightly smaller CD micelles than casein micelles, in agreement with above result of pyrene fluorescence. Meanwhile, at pH 7 higher than the isoelectric point (pI) of casein (pH ≈ 4.6), negative values of zeta potential are measured for both casein micelles (−28.3 mV) and CD micelles (−18.1 mV). The decrease in the absolute value of zeta potential for CD micelles is ascribed to the highly hydratable dextran conjugated to casein, which may decrease the electrophoresis mobility of CD micelles and result in smaller zeta potential.
The stability of casein micelles and CD micelles is compared at different pHs by the turbidity measurement. As shown in , while the turbidity of casein remains small and constant at pH lower than 3 and at pH higher than 6, it increases sharply in the pH range of 3.5–5.5. It is known that casein exists in the form of small micelles in the pH ranges 2–3, and 6–12.[Citation34] Obviously, the sharp increase in turbidity of casein in the pH range of 3.5–5.5 is an indication of the formation of big casein aggregates that are large enough to induce precipitates, owing to almost zero net charge of casein when the pH is near pI of casein. It is also noted that the turbidity of CD is smaller than that of casein in the whole pH range of 2–8. Especially in the pH range of 3.5–5.5, the quite smaller turbidity of CD compared to casein is supported by the observation of no precipitate but transparent dispersion in CD sample. The data of zeta potential reveal that CD micelles carry lower charges than casein micelles. Thus, instead of the electrostatic repulsion, the enhanced stability of CD micelles should be raised from the steric repulsion of the conjugated dextran segments which form a hydrophilic shell surrounding casein core. Herein, the conjugation of casein with dextran may effectively inhibit the aggregation and precipitation behaviours of casein, which is beneficial for the development of controlled-releasable carriers for bioactive compounds.
Binding of curcumin with CD micelles
The intrinsic fluorescence of protein is mainly due to tryptophan residues located in the hydrophobic pockets inside the tertiary structure of protein. While as1-casein and as2-casein contain two tryptophan residues at positions (164 and 199) and (109 and 193), respectively, β-casein and κ-casein have just one tryptophan residue at positions 143 and 76, respectively.[Citation35] As shown in , casein micelles have a characteristic fluorescence maximum at 340.6 nm, whereas at 342.8 nm, CD micelles have a reduced fluorescence maximum of about 74% of casein micelles. The markedly weakened fluorescence intensity of CD micelles gives an evidence to support the occurrence of Maillard reaction of casein with dextran and the shielding effect of dextran shell on the fluorescence of casein. Meanwhile, two wavelength values of fluorescence maximum are observed in the range of 335–350 nm, which indicates that tryptophan residues are buried in hydrophobic microdomains in casein micelles and CD micelles.[Citation34] It is known that the fluorescence intensity decreases with a red shift of maximum wavelength when the protein is denatured and unfolded. In CD micelles assembled by dextran-conjugated casein via the Maillard reaction, little red shift of fluorescence maximum suggests that casein undergoes small conformational change which induced more exposure of tryptophan residues, as discussed in pyrene fluorescence and particle size results.
Figure 4. Fluorescence spectra of casein in casein micelles and casein–dextran conjugate micelles at pH 7 (A). Variations of fluorescence spectra of casein in casein micelles (B) and casein–dextran conjugate micelles (C) as a function of curcumin concentration at pH 7. Spectra a–k correspond to 0, 1, 2, 3, 4, 5, 6, 7, 8, 9, and 10 μM curcumin, respectively. (Insets) Linear plots of for the determination of binding constants of curcumin with casein and casein-dextran conjugate micelles.
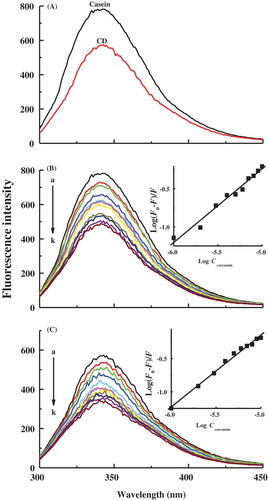
and depict the fluorescence spectra of casein in casein micelles and CD micelles as a function of curcumin concentration at pH 7. The addition of increasing concentration of curcumin usually causes a progressive reduction in the fluorescence intensity of casein, as well as a little blue shift of fluorescence maximum wavelength. Curcumin is a high hydrophobic molecule with a β-diketone moiety flanked by two phenolic groups. Regarding the dissociation of three acid protons, curcumin has three pKa values of 8.38, 9.88, and 10.51 in aqueous solution.[Citation36] At pH 7, curcumin is predominantly in the neutral form, which suggests that binding of curcumin with casein micelles and CD micelles is mainly based on hydrophobic interaction instead of electrostatic force. As discussed above, tryptophan residues are mostly located in the interior hydrophobic microdomains in casein micelles and CD micelles. Therefore, the hydrophobic interaction of curcumin with the hydrophobic groups near tryptophan residues inside protein core of micelles may result in the quenching of fluorescence signal. The hydrophobic binding of the aryl groups of polyphenolic compounds to the hydrophobic pockets of protein was previously identified by Rawel et al. by the addition of urea to destroy the noncovalent interactions.[Citation37]
Fluorescence quenching of tryptophan residues of proteins with polyphenolic compounds is generally initiated by the static quenching from the formation of ground-state complexes between the protein and the quencher.[Citation38,Citation39] It is assumed that there are “n” same and independent binding sites in protein micelles where curcumin binds according to the following model:
where P denotes protein micelles, L is curcumin, and PLn is the complexes of curcumin with protein micelles. For this static quenching, the equilibrium between free and bound curcumin is given by the double logarithm equation[Citation29]
where F0 and F are the intensities of casein fluorescence maximum in the absence and in the presence of different concentrations of curcumin, respectively, Ccurcumin is curcumin concentration, Kb is the binding constant, and n is the number of binding sites. As given in the insets of , the linear fitting plots of the double logarithm curve of were drawn using the changes in fluorescence maximum of casein. From the slope and intercept of the plots, the values of Kb were obtained as 3.9 × 104 and 1.8 × 105 M−1 for the bindings of curcumin with casein micelles and CD micelles, respectively. The value of Kb for the binding of curcumin with casein micelles is usually in the range of 103–104 M−1,[Citation8,Citation10,Citation40] while Nadi et al. recently reported the high Kb values for the binding of curcumin to BSA and casein.[Citation41] These varied Kb values may be ascribed to the reasons such as the material sources, the experimental conditions, and the fitting models. In the current work, the observed higher Kb value of 1.8 × 105 M−1 indicates the increased binding affinity of curcumin with CD micelles compared to that of curcumin with casein micelles. Esmaili et al.[Citation9] and Yazdi et al.[Citation35] found that casein has an increased binding affinity with curcumin at high temperature, which was attributed to the heat-induced exposure of more hydrophobic groups of casein. In our work, heat treatment in the Maillard reaction may cause partial unfolding of casein and finally lead to more hydrophobic microdomains in CD micelles. As a result, curcumin can penetrate inside to establish more hydrophobic contact with tryptophan residues in CD micelles than that of casein micelles.
Curcumin also has intrinsic fluorescence, which is often used to monitor its binding with proteins,[Citation11,Citation13] because of the sensitive responses of the intensity and position of fluorescence maximum of curcumin to the medium environment. gives the spectra of steady-state fluorescence of three curcumin samples at pH 7. Free curcumin shows a low-intensity broad fluorescence peak at around 550 nm, which is similar to the fluorescence features of curcumin in aqueous buffers.[Citation42] However, in casein micelles and CD micelles, the fluorescence spectrum of curcumin is blue shifted to the well-defined peaks at 505.6 and 504.2 nm, respectively, and the intensity sharply increased compared to that in water. The significant blue shifting of the fluorescence peak of curcumin from water to protein micelles can be attributed to the movement of curcumin from a hydrophilic to a hydrophobic microenvironment, because the aryl groups of curcumin feasibly hydrophobically bind to the hydrophobic microdomains of casein micelles and CD micelles. Compared to casein micelles, the decreased intensity and little blue shift of curcumin fluorescence in CD micelles reveal that this kind of micelles can provide more hydrophobic microdomains for the binding of curcumin but brings about somewhat quenching effect on curcumin fluorescence.
Figure 5. Fluorescence spectra of curcumin in water, casein micelles, and casein–dextran conjugate micelles at pH 7.
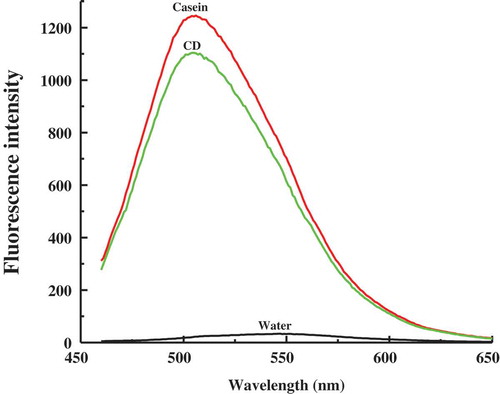
Fluorescence anisotropy (r) of curcumin can directly give information on the binding location of curcumin in casein micelles and CD micelles at pH 7. The r values of curcumin obtained by fluorescence polarization technique are 0.079, 0.14, and 0.16 in water, casein micelles, and CD micelles, respectively. Usually, a higher r value reveals greater restriction of the rotation of the fluorophore during the excited lifetime. The higher r value of curcumin in the presence of casein micelles is the manifestation of the transfer of curcumin from the bulk water to the hydrophobic microdomains of casein micelles, which could give not only smaller polaric but also stronger rigidic microenvironment for curcumin.[Citation29] After encapsulated in CD micelles, curcumin continues to give a slight increase of r value, although this change of r value is not significant compared to the change from water to casein micelles. This result can support our above discussion that CD micelles may offer a more hydrophobic microenvironment for bound curcumin, where curcumin experiences a higher restricted motion in CD micelles and, therefore, a further increase in the anisotropy occurs.
Stability and antioxidant activity of curcumin with CD micelles
In order to study the encapsulation activity of curcumin with casein micelles and CD micelles, we have compared the stability of 10 µM curcumin in the samples of free curcumin, curcumin with casein micelles, and curcumin with CD micelles. gives the changes of the residual amounts of curcumin in various curcumin samples as a function of time in pH 7 at 25°C. (The stability of curcumin in these samples gives the similar changes in the other pH and temperature values.) After 360 min incubation, the residual amounts of curcumin in water, casein micelles, and CD micelles decay to about 23%, 52%, and 73%, respectively. The residual amounts of free curcumin and encapsulated curcumin were fitted to the pseudo-first-order kinetic equation. From the linear regression lines of the residual amount of curcumin against time, the observed pseudo-first-order rate constants of curcumin degradation (kobs) are obtained as 0.0025, 0.0012, and 0.00032 min−1 for free curcumin, curcumin in casein micelles, and curcumin in CD micelles, respectively. The mechanism, kinetics, and products of free curcumin degradation have been well investigated at various pHs.[Citation6,Citation7] At neutral and basic pHs, the rapid degradation of free curcumin is induced by the destruction of the conjugated diene structure due to the loss of a proton from the activated carbon atom in the keto-enol group of curcumin.[Citation43] Both the changes of values of residual amount and kobs of curcumin suggest that protein micellar encapsulation can significantly inhibit curcumin degradation. When curcumin binds to the hydrophobic microdomains of casein micelles and CD micelles, the greatly enhanced stability of curcumin indicates that the keto-enol group of curcumin is effectively protected from aqueous medium. The highest suppression yield of curcumin in CD micelles should be ascribed to their more hydrophobic microenvironment, corresponding well to the highest binding affinity of curcumin with CD micelles. Additionally, in CD micelles, the outer dextran shell around casein core may prevent the interaction of curcumin with water more sufficiently, which plays a positive role in inhibiting degradation of curcumin.
Figure 6. The pseudo-first-order plots for the degradation of curcumin in water, casein micelles, and casein–dextran conjugate micelles at pH 7. The data are normalized to a value of 100 at zero time. Points represent the experimental data and the solid lines were drawn using pseudo-first-order kinetic equation.
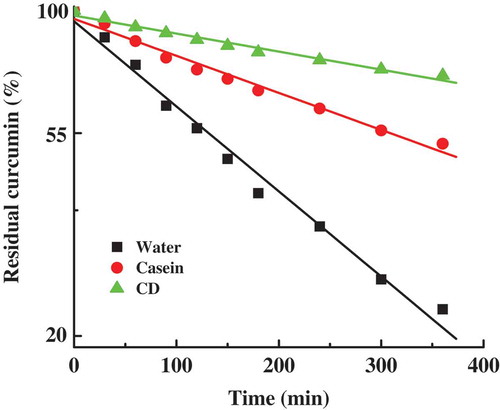
The ABTS method is used to test the radical scavenging ability of 10 µM curcumin, through measuring the reduction of the radical cation ABTS+ as the percentage inhibition of absorbance at 734 nm.[Citation31] presents the absorption spectra of ABTS+ alone and in the presence of casein micelles, CD micelles, curcumin, curcumin with casein micelles, and curcumin with CD micelles. Casein micelles and CD micelles are observed to reduce the absorbance of ABTS+ at 734 nm, which is due to hydrogen donor groups of indole, phenyl, and imidazole in amino acid residues such as tryptophan and methionine cysteine.[Citation44,Citation45] Although curcumin rapidly degrades at physiological pH, the recent work revealed that curcumin itself, instead of its degradation products, is largely responsible for the observed biological actions of curcumin.[Citation46] The high effectiveness of radical scavenging ability of curcumin is mainly contributed by its phenolic groups, which could scavenge ABTS+ radical by donating their H atoms.[Citation42] It is noteworthy that the combination of curcumin with casein micelles or CD micelles can significantly improve radical scavenging activity of curcumin. The added amount of curcumin is same in three curcumin samples. Owing to the high hydrophobicity of curcumin, curcumin is expected to bind to the hydrophobic part of casein micelles and CD micelles. Considering the same protein content in the samples of casein micelles and CD micelles, it is also expected that the same number of curcumin molecules bind in casein micelles and CD micelles. The increased antioxidant activity of curcumin after dispersion in casein micelles was reported.[Citation9,Citation47] In agreement with the highest stability of curcumin in CD micelles, the strongest antioxidant of curcumin in CD micelles suggests that this kind of micelles can provide a more proper microenvironment for the reaction of curcumin with ABTS+, where curcumin can donate H atoms to reduce ABTS+ into nonradical form more easily.
Conclusion
In summary, the self-assembled CD micelles have the advantage over casein micelles of higher structural stability due to the more hydrophobic and compact casein core and the hydrophilic dextran shell. Compared to casein micelles, CD micelles can provide a better hydrophobic microenvironment for the hydrophobic binding of curcumin, exhibiting bigger binding constant, more enhanced stability, and stronger radical scavenging ability. While the Maillard reaction has been widely investigated in terms of the preparation conditions, this work reveals that self-assembled micelles of protein–polysaccharide conjugates could be used as effective encapsulation carriers for bioactive hydrophobic compounds.
Funding
This work is supported by the National Natural Science Foundation of China (Grant 21573071) and the Fundamental Research Funds for the Central Universities (Grant WK1213003).
Additional information
Funding
References
- Sharma, O.P. Antioxidant Activity of Curcumin and Related Compounds. Biochemical Pharmacology 1976, 25, 1811–1812.
- Khar, A.; Ali, A.M.; Pardhasaradhi, B.V.; Begum, Z.; Anjum, R. Antitumor Activity of Curcumin Is Mediated through the Induction of Apoptosis in AK-5 Tumor Cells. FEBS Letters 1999, 445, 165–168.
- Aggarwal, B.B.; Kumar, A.; Bharti, A.C. Anticancer Potential of Curcumin: Preclinical and Clinical Studies. Anticancer Research 2003, 23, 363–398.
- Ruby, A.J.; Kuttan, G.; Babu, K.D.; Rajasekharan, K.N.; Kuttan, R. Anti-Tumour and Antioxidant Activity of Natural Curcuminoids. Cancer Letters 1995, 94, 79–83.
- Anand, P.; Kunnumakkara, A.B.; Newman, R.A.; Aggarwal, B.B. Bioavailability of Curcumin: Problems and Promises. Molecular Pharmaceutics 2007, 4, 807–818.
- Tønnesen, H.H.; Karlsen, J. Studies on Curcumin and Curcuminoids. VI. Kinetics of Curcumin Degradation in Aqueous Solution. Zeitschrift für Lebensmittel-Untersuchung und Forschung 1985, 180, 402–404.
- Wang, Y.J.; Pan, M.H.; Cheng, A.L.; Lin, L.I.; Ho, Y.S.; Hsieh, C.Y.; Lin, J.K. Stability of Curcumin in Buffer Solutions and Characterization of Its Degradation Products. Journal of Pharmaceutical and Biomedical Analysis 1997, 15, 1867–1876.
- Sneharani, A.H.; Singh, S.A.; Rao, A.G.A. Interaction of αS1-Casein with Curcumin and Its Biological Implications. Journal of Agricultural and Food Chemistry 2009, 57, 10386–10391.
- Esmaili, M.; Ghaffari, S.M.; Moosavi-Movahedi, Z.; Atri, M.S.; Sharifizadeh, A.; Farhadi, M.; Yousefi, R.; Chobert, J.-M.; Haertlé, T.; Moosavi-Movahedi, A.A. Beta Casein-Micelle as a Nano Vehicle for Solubility Enhancement of Curcumin; Food Industry Application. LWT–Food Science and Technology 2011, 44, 2166–2172.
- Benzaria, A.; Maresca, M.; Taieb, N.; Dumay, E. Interaction of Curcumin with Phosphocasein Micelles Processed or Not by Dynamic High-Pressure. Food Chemistry 2013, 138, 2327–2337.
- Sneharani, A.H.; Karakkat, J.V.; Singh, S.A.; Rao, A.G.A. Interaction of Curcumin with β-Lactoglobulin: Stability, Spectroscopic Analysis, and Molecular Modeling of the Complex. Journal of Agricultural and Food Chemistry 2010, 58, 11130–11139.
- Tapal, A.; Tiku, P.K. Complexation of Curcumin with Soy Protein Isolate and Its Implications on Solubility and Stability of Curcumin. Food Chemistry 2012, 130, 960–965.
- Teng, Z.; Luo, Y.; Wang, Q. Nanoparticles Synthesized from Soy Protein: Preparation, Characterization, and Application for Nutraceutical Encapsulation. Journal of Agricultural and Food Chemistry 2012, 60, 2712−2720.
- Dickinson, E.; Pawlowsky, K. Effect of ι-Carrageenan on Flocculation, Creaming, and Rheology of a Protein-Stabilized Emulsion. Journal of Agricultural and Food Chemistry 1997, 45, 3799–3806.
- McClements, D.J. Food Emulsions: Principles, Practices, and Techniques; 2nd ed.; CRC Press: Boca Raton, FL, 2004.
- Jones, O.G.; McClements, D.J. Recent Progress in Biopolymer Nanoparticle and Microparticle Formation by Heat-Treating Electrostatic Protein-Polysaccharide Complexes. Advances in Colloid and Interface Science 2011, 167, 49–62.
- Yang, M.; Wu, Y.; Li, J.; Zhou, H.; Wang, X. Binding of Curcumin with Bovine Serum Albumin in the Presence of ι-Carrageenan and Implications on the Stability and Antioxidant Activity of Curcumin. Journal of Agricultural and Food Chemistry 2013, 61, 7150−7155.
- Li, J.; Wang, X. Binding of (–)-Epigallocatechin-3-Gallate with Thermally-Induced Bovine Serum Albumin/ι-Carrageenan Particles. Food Chemistry 2015, 168, 566–571.
- Pan, X.; Mu, M.; Hu, B.; Yao, P.; Jiang, M. Micellization of Casein-Graft-Dextran Copolymer Prepared through Maillard Reaction. Biopolymers 2006, 81, 29–38.
- Morris, G.A.; Sims, I.M.; Robertson, A.J.; Furneaux, R.H. Investigation into the Physical and Chemical Properties of Sodium Caseinate-Maltodextrin Glyco-Conjugates. Food Hydrocolloids 2004, 18, 1007–1014.
- Xue, J.; Tan, C.; Zhang, Xi.; Feng, B.; Xia, S. Fabrication of Epigallocatechin-3-Gallate Nanocarrier Based on Glycosylated Casein: Stability and Interaction Mechanism. Journal of Agricultural and Food Chemistry 2014, 62, 4677−4684.
- Aminlari, M.; Ramezani, R.; Jadidi, F. Effect of Maillard-Based Conjugation with Dextran on the Functional Properties of Lysozyme and Casein. Journal of the Science of Food and Agriculture 2005, 85, 2617–2624.
- Xia, S.; Li, Y.; Zhao, Q.; Li, J.; Xia, Q.; Zhang, X.; Huang, Q. Probing Conformational Change of Bovine Serum Albumin−Dextran Conjugates under Controlled Dry Heating. Journal of Agricultural and Food Chemistry 2015, 63, 4080−4086.
- Xia, S.; Li, Y.; Xia, Q.; Zhang, X.; Huang, Q. Glycosylation of Bovine Serum Albumin via Maillard Reaction Prevents Epigallocatechin-3-Gallate-Induced Protein Aggregation. Food Hydrocolloids 2015, 43, 228−235.
- Takano, K.; Hattori, M.; Yoshida, T.; Kanuma, S.; Takahashi, K. Porphyran as a Functional Modifier of a Soybean Protein Isolate through Conjugation by the Maillard Reaction. Journal of Agricultural and Food Chemistry 2007, 55, 5796−5802.
- Pan, X.; Yao, P.; Jiang, M. Simultaneous Nanoparticle Formation and Encapsulation Driven by Hydrophobic Interaction of Casein-Graft-Dextran and β-Carotene. Journal of Colloid and Interface Science 2007, 315, 456–463.
- Li, Z.; Gu, L. Fabrication of Self-Assembled (−)-Epigallocatechin Gallate (EGCG) Ovalbumin−Dextran Conjugate Nanoparticles and Their Transport Across Monolayers of Human Intestinal Epithelial Caco-2 Cells. Journal of Agricultural and Food Chemistry 2014, 62, 1301−1309.
- Goodno, C.C.; Swaisgood, H.E.; Catignani, G.L. A Fluorimetric Assay for Available Lysine in Proteins. Analytical Biochemistry 1981, 115, 203–211.
- Barik, A.; Priyadarsini, K.I.; Mohan, H. Photophysical Studies on Binding of Curcumin to Bovine Serum Albumin. Photochemistry and Photobiology 2003, 77, 597–603.
- Shinitzky, M.; Barenholz, Y. Fluidity Parameters of Lipid Regions Determined by Fluorescence Polarization. Biochimica et Biophysica Acta 1978, 515, 367–394.
- Re, R.; Pellegrini, N.; Proteggente, A.; Pannala, A.; Yang, M.; Rice-Evans, C. Antioxidant Activity Applying an Improved ABTS Radical Cation Decolorization Assay. Free Radical Biology & Medicine 1999, 26, 1231–1237.
- Mu, M.; Pan, X.; Yao, P.; Jiang, M. Acidic Solution Properties of β-Casein-Graft-Dextran Copolymer Prepared through Maillard Reaction. Journal of Colloid and Interface Science 2006, 301, 98–106.
- Wang, X.; Wang, J.; Wang, Y.; Yan, H. Effect of the Nature of the Spacer on the Aggregation Properties of gemini Surfactants in an Aqueous Solution. Langmuir 2004, 20, 53–56.
- Liu, Y.; Guo, R. pH-Dependent Structures and Properties of Casein Micelles. Biophysical Chemistry 2008, 136, 67–73.
- Yazdi, S.R.; Corredig, M. Heating of Milk Alters the Binding of Curcumin to Casein Micelles. Food Chemistry 2012, 132, 1143–1149.
- Bernabé-Pineda, M.; Ramírez-Silva, M.T.; Romero-Romo, M.; González-Vergara, E.; Rojas-Hernández, A. Determination of Acidity Constants of Curcumin in Aqueous Solution and Apparent Rate Constant of Its Decomposition. Spectrochimica Acta Part A 2004, 60, 1091–1097.
- Rawel, H.M.; Meidtner, K.; Kroll, J. Binding of Selected Phenolic Compounds to Proteins. Journal of Agricultural and Food Chemistry 2005, 53, 4228–4235.
- Shpigelman, A.; Israeli, G.; Livney, Y.D. Thermally-Induced Protein–Polyphenol co–Assemblies: Beta Lactoglobulin–Based Nanocomplexes as Protective Nanovehicles for EGCG. Food Hydrocolloids 2010, 24, 735–743.
- Wang, Y.; Wang, X. Binding, Stability, and Antioxidant Activity of Quercetin with Soy Protein Isolate Particles. Food Chemistry 2015, 188, 24–29.
- Sahu, A.; Kasoju, N.; Bora, U. Fluorescence Study of the Curcumin-Casein Micelle Complexation and Its Application as a Drug Nanocarrier to Cancer Cells. Biomacromolecules 2008, 9, 2905–2912.
- Nadi, M.M.; Kooshk, M.R.A.; Mansouri, K.; Ghadami, S.A.; Amani, M.; Ghobadi, S.; Khodarahmi, R. Comparative Spectroscopic Studies on Curcumin Stabilization by Association to Bovine Serum Albumin and Casein: A Perspective on Drug-Delivery Application. International Journal of Food Properties 2015, 18, 638–659.
- Began, G.; Sudharshan, E.; Sankar, K.U.; Rao, A.G.A. Interaction of Curcumin with Phosphatidylcholine: A Spectrofluorometric Study. Journal of Agricultural and Food Chemistry 1999, 47, 4992−4997.
- Jovanovic, S.V.; Steenken, S.; Boone, C.W.; Simic, M.G. H-Atom Transfer is a Preferred Antioxidant Mechanism of Curcumin. Journal of the American Chemical Society 1999, 121, 9677–9681.
- Levine, R.; Mosoni, L.; Berlett, B.; Stadtman, E. Methionine Residues as Endogenous Antioxidants in Proteins. Proceedings of the National Academy of Sciences (USA) 1996, 93, 15036–15040.
- Dong, S.; Wei, B.; Chen, B.; Mcclements, D.J.; Decker, E.A. Chemical and Antioxidant Properties of Casein Peptide and Its Glucose Maillard Reaction Products in Fish Oil-in-Water Emulsions. Journal of Agricultural and Food Chemistry 2011, 59, 13311−13317.
- Nimiya, Y.; Wang, W.; Du, Z.; Sukamtoh, E.; Zhu, J.; Decker, E.; Zhang, G. Redox Modulation of Curcumin Stability: Redox Active Antioxidants Increase Chemical Stability of Curcumin. Molecular Nutrition & Food Research 2016, 60, 487–494.
- Pan, K.; Zhong, Q.; Baek, S.J. Enhanced Dispersibility and Bioactivity of Curcumin by Encapsulation in Casein Nanocapsules. Journal of Agricultural and Food Chemistry 2013, 61, 6036−6043.