ABSTRACT
Marine oils, such as seal blubber and menhaden oils, offer a wide range of health benefits and play a critical role in many functions in the human body. These oils are highly vulnerable to oxidation due to their high content of polyunsaturated fatty acids. In this study, the stabilizing effect of blending of seal blubber and menhaden oils with antioxidant rich-wheat germ oil was investigated. Conjugated dienes and thiobarbituric acid reactive substances were used as indicators of oxidative stability. Fatty acid composition of the original oils and blends was determined using gas chromatography, while tocopherol content was assessed using high-performance liquid chromatography-mass spectrometry. The results showed that blending with wheat germ oil increased the tocopherol content of seal blubber and menhaden oils. The oxidative stability of blended seal blubber and menhaden oils was improved, and higher oxidative stability was positively correlated with higher percentage of added wheat germ oil.
Introduction
Oils from marine mammals, fish, and microalgae have been well recognised as preferable supplementary sources of long-chain omega-3 polyunsaturated fatty acids (PUFAs). For instance, seal blubber oil (SBO) contains high contents of omega-3 fatty acids such as eicosapentaenoic acid (EPA), docosapentaenoic acid (DPA), and docosahexaenoic acid (DHA), while all these fatty acids can also be found in menhaden oil (MHO).[Citation1,Citation2] On the other hand, the oil derived from Crypthecodinium cohnii microalgae contains about 40% DHA.[Citation3] A large body of studies has demonstrated the health benefits of these PUFAs in humans. As the main components of membrane lipids, DHA supports normal development of the brain, eyes, and the nervous system, especially for infants and foetuses, and thus addition of DHA in the diet of pregnant and lactating women as well as infant formula is strongly recommended.[Citation4–Citation7] Furthermore, PUFA such as EPA plays a vital role in inflammation, regulating blood flow, immune response, and ion transport by serving as a precursor of signalling eicosanoid molecules. In addition, long-chain omega-3 PUFAs can be converted to other anti-inflammatory molecules, such as resolvins, maresins, and omega-3-oxylipins,[Citation8] which may also explain the function of omega-3 fatty acids in alleviating cancer,[Citation9] cardiovascular disease,[Citation10] psychiatric disorders,[Citation11] Parkinson’s disease,[Citation12] and inflammatory conditions.[Citation13] However, these oils are highly vulnerable to oxidation due to their high content of PUFA.
As a rich source of omega-3 fatty acids, seal blubber and fish oils have attracted attention from food and food supplement industries. These oils have been directly used as food supplement to address health problems.[Citation14] In food industry, SBO and MHO are used in bakery goods, such as bread, as well as baby foods, salad dressings, and dairy products, among others.[Citation15] In addition, the microencapsulated SBO and MMHO have been incorporated into the formulations of dry foods and other beverages.[Citation16] Oxidation of lipid can degrade the quality of foods and produces toxic oxidation products, and the low oxidative stability of marine omega-3 oils has been a major challenge for their application in foods or being used as pharmaceutical ingredients. For many lipid-containing foods, special measures are taken to reduce or prevent oxidation, including removal of oxygen as well as photosensitisers, and addition of natural and/or synthetic antioxidants. In this study, the effect of blending with antioxidant rich-wheat germ oil (WGO) on the oxidative stability of SBO and MHO was investigated.
WGO is well known as a tocopherol-rich food lipid, which is procured from the germ of wheat kernel. It contains more than 55% PUFAs, mainly linoleic and alpha-linolenic acids.[Citation17] Octacosanol, a 28-carbon long-chain saturated primary alcohol, has also been found as one of the minor components of WGO, which is believed to serve as a physical performance enhancing agent as well as for improving Parkinson’s disease and lowering plasma cholesterol.[Citation18,Citation19]
Oil blending has commonly been employed in the pharmaceutical and food industries.[Citation20] For instance, omega 3 oils have been added to other oils in order to maximise the health benefits of the resultant formulations. Blended oils from fish oil and flax seed oil are considered as new pharmaceutical products with maximised benefits from both.[Citation21] In the food industry, palm oil has been used to enhance the stability of cooking oils such as canola oil, during food processing and high-temperature deep-fat frying. Addition of WGO to SBO and MHO may not only be expected to improve their oxidative stability with the increased content of tocopherols but also to enhance health benefits contributed from the addition of its minor component, octacosanol.
The purpose of the present study was to examine the effect of blending SBO and MHO with WGO on their oxidative stability. Changes of tocopherol content and fatty acid composition in blended SBO and MHO was assessed by high-performance liquid chromatography-mass spectrometry (HPLC-MS) and gas chromatography (GC), respectively. Oxidative stability of blended oils was monitored by comparing conjugated dienes (CD) and thiobarbituric acid reactive substances (TBARS) values following accelerated oxidation at 60°C for 72 h with those of the original oils.
Materials and methods
Materials
The compound 2-thiobarbituric acid and standards of tocopherols were purchased from Sigma-Aldrich (Mississauga, ON, Canada). Standard fatty acid methyl esters (FAMEs; GLC-461) were procured from Nu-Check (Elysian, MN). WGO was purchased from Jedwards International, Inc. (Braintree, MA). SBO was received as a gift from a local marine oil company in Newfoundland (St. John’s, NL, Canada), and MHO was received as a gift from Omega Protein (Houston, TX). All solvents used were of analytical grade and purchased from Fisher Scientific (Nepan, ON, Canada).
Blending SBO and MHO with WGO
SBO (19 or 18 g) and MHO (19 or 18 g) were physically blended with 5% (1 g) and 10% (2 g) of WGO, respectively. All the oil samples tested were flushed with nitrogen gas and stored at −20°C for up to 14 days before analysis.
Determination of fatty acid composition of SBO, MHO, WGO, and their blended counterparts by GC analysis
Fatty acid compositions of SBO, MHO, WGO, SBO with 5% of WGO, SBO with 10% of WGO, MHO with 5% of WGO, MHO with 10% of WGO were determined following their conversion to the corresponding methyl esters.[Citation22] A Hewlett-Packard 5890 Series II gas chromatograph (Agilent, Palo Alto, CA) equipped with a SUPELCOWAX-10 column (30 m length, 0.25 mm diameter, 0.25 μm film thickness; Supelco Canada Ltd., Oakville, ON, Canada) was used to analyse the FAMEs. The FAMEs were identified by comparing their retention times with those of a known standard mixture, and the results were presented as weight percentage.
Determination of tocopherols content by HPLC-MS
Assessment of tocopherol content in SBO, MHO, WGO, and their blends was conducted in this study. The tested oil samples were prepared by dissolving 0.1 g of oil in 2 mL of methonal–acetonitrile–isopropanol (41:59:300, v/v/v). Tocopherol standards were prepared by dissolving a known amount of tocopherol mixture (consisting of four tocopherol analogues) in the same solvent followed by dilution to different concentrations. Prior to HPLC analysis, both samples and standards were filtered using a 0.45 μm syringe filter (Whatman, Clifton, NJ).
Tocopherol contents in the prepared samples and standards were determined by reversed phase HPLC-MS, using an Agilent 1100 HPLC unit (Agilent Technologies, Palo Alto, CA), equipped with a UV–diode array detector (UV-DAD). Separation was achieved on a C-18 column (4.6 mm × 250 mm coupled with a guard column, Agilent) by gradient elution with a methanol–acetonitrile–isopropanol mobile phase at a flow of 0.8 mL/min; and fractions were detected at 295 nm. The mobile phase started with methanol–acetonitrile–isopropanol (41:59:0, v/v/v) and was maintained for 15 min. The mobile phase was gradually changed to methanol–acetonitrile–isopropanol (16.5:23.5:60, v/v/v) from 15 to 25 min, and then it reached 100% of isopropanol from 25 to 35 min, and was kept there for 10 min. The mobile phase was changed to its initial state, methanol–acetonitrile–isopropanol (41:59:0, v/v/v) in 5 min, and it was maintained for 10 min in order to recondition the column. LC flow was analysed online by a mass spectrometric detector system (LC-MSD-Trap-SL, Agilent) using positive ion APCI (atmospheric pressure chemical ionisation). The operating conditions used were 121 V for the fragmentor voltage, drying temperature of 350°C, APCI temperature of 400°C, nebuliser pressure of 60 psi, drying gas flow of 7 L/min. Each tocopherol standard and sample (50 μL) was injected by using an autosampler. Tocopherols were detected at 295 nm by a UV detector and identified by comparing their retention times with those of known tocopherol standards. A standard curve was constructed for each tocopherol homolog (peak area vs. concentration). Tocopherol concentrations in samples were calculated using the standard curve and expressed as milligram tocopherol per 100 g oil.
Oxidative stability tests
CD test
Oxidative stability of SBO, MHO, WGO, and their blends was determined under Schaal oven conditions at 60°C for 72 h. Each day (24 h) under these conditions is equivalent to 1 month of storage at ambient temperatures. Oils (0.4–0.5 g) were placed in loosely capped test tubes (10 mm diameter and 4.0 cm height) and stored at 60°C in a forced-air oven (Thelco, Model 2; Precision Scientific Co., Chicago, IL). Samples were removed from the oven at 0, 6, 12, 24, 48, and 72 h, cooled to room temperature, flushed with nitrogen, capped, and stored at −20°C for up to 7 days until analysed. CD in the oils was determined according to the IUPAC (Citation1987) method 20505.[Citation23]
TBA test
The determination of TBARS was carried out as described by the AOCS method Cd 19–90.[Citation24]
Statistical analysis
All tests were done in triplicate. The results obtained were presented as mean ± standard deviation. Paired t-test was performed to analyse the significant differences between data (p < 0.05) using SPSS statistics.
Results and discussion
Fatty acid composition of SBO, MHO, WGO, and their blends
The fatty acid composition of SBO, MHO, WGO, and their blends are shown in and . The fatty acid composition of SBO, MHO, WGO reported here are in good agreement with those from previous work, and the differences are mainly due to different batches of the oils used.[Citation25,Citation26] WGO is a rich source of unsaturated fatty acids, including the PUFA C18:2ω6 and the monounsaturated fatty acid C18:1ω9 (). More than 80% of the total fatty acids were present as C18:2ω6 (55.53%) and C18:1ω – 9 (25.4%). Alpha-linolenic acid (C18:3ω3) was the only omega-3 fatty acid that was detected in WGO at 3.6%. The saturated fatty acids detected were C14:0, C16:0, and C18:0.
Table 1. Fatty acid composition of original seal blubber oil (SBO), seal blubber oil blended with 5% and 10% wheat germ oil, and original wheat germ oil (WGO).
Table 2. Fatty acid composition of original menhaden oil (MHO), menhaden oil blended with 5% and 10% of wheat germ oil, and original wheat germ oil (WGO).
Seal bubbler oil before and after blending with WGO
SBO is an important source of omega-3 fatty acids, including C18:3ω3 (0.42%), C18:4ω3 (0.89%), C20:5ω3 (4.74%), C22:5ω3 (3.5%), and C22:5ω3 (6.08%) (). The fatty acids C14:1ω9 (1.27%), C16:1ω7 (19.03%), C17:1 (0.28%), C18:1ω9 (25.2%), C18:1ω11 (6.68%), C18:2ω6 (2.01%), and C22:1ω11 (2.14%) were detected as unsaturated forms. In addition, C14:0 (4.32%), C16:0 (5.89%), and C18:0 (0.75%) were saturated fatty acids detected in SBO. The change in total content of unsaturated fatty acids between the original SBO and its blends was insignificant (p > 0.05) (). However, the content of the total PUFA showed a significant increase in the blended SBO compared with that of the content in the original SBO.
Menhaden oil before and after blending with WGO
All the omega-3 fatty acids from SBO were also detected in the original MHO, such as C18:3ω3 (0.71%), C18:4ω3 (1.8%), C20:5ω3 (16.26%), C22:5ω3 (1.88%), and C22:6ω3 (5.77%) (). The total content of omega-3 fatty acids in MHO was significantly higher than that of SBO. On the other hand, unsaturated fatty acids, including C14:1ω9 (0.59%), C16:1ω7 (18.56%), C17:1 (1.47%), C18:1ω9 (9.1%), C18:1ω11 (4.51%), C18:2ω6 (0.88%), and C20:1ω9 (0.81%) were found in MHO. Only C14:0 (12.77%), C16:0 (18.56%), and C18:0 (2.74%) were the saturated fatty acids detected in MHO (). The total content of unsaturated fatty acids of the original MHO and its blends did not change significantly (p > 0.05). However, a significant increase in total PUFA was observed in the MHO blends compared with that of the original MHO.
Tocopherol content
Alpha-tocopherol is the natural antioxidant in marine oils and was the major tocopherol homologue detected in both SBO and MHO.[Citation27] The results () showed that the alpha-tocopherol content was 14.5 mg/100 g in SBO, while it was 8.92 mg/100 g in MHO; these values are higher than those reported previously.[Citation25,Citation28,Citation29] Tocopherol contents in this work were directly analysed after dissolving of a small amount of oil sample in the solvent without saponification, and thus the accuracy of determination was improved as loss of tocopherol during alkaline saponification and extraction was prevented. The higher alpha-tocopherol content so obtained may also be due to the different refining process and characteristics of the test oil in this work. All four tocopherol homologues including alpha-, beta-, gamma-, and delta tocopheols were detected in WGO, and its total tocopherol content was 124 mg/100 g, which is 8 times that in SBO and 13 times that in MHO ( and ). These results demonstrated that WGO is a rich source of tocopherols, and the addition of WGO to SBO and MHO led to an increase of tocopherol content in both oils.[Citation17] Based on the results, a significant (p < 0.05) increase in the content of α-tocopherol and appearance of other tocopherol homologues was noted in both blended SBO and MHO. The total tocopherol contents of SBO with 5% WGO and SBO with 10% WGO blends increased to 19.98 and 25.18 mg/100 g, respectively; while those in blended MHO reached 14.68 (5%WGO) and 20.42 (10% of WGO) mg/100 g.
Table 3. Tocopherol contents (mg/100 g of oil) in original seal blubber oil (SBO), menhaden oil (MHO), wheat germ oil (WGO), and their blends.
Oxidative stability
CD test
The CD value of lipids, measured by their absorption at 234 nm, is an indicator of primary oxidation products as CD are formed due to a shift in the double-bond positions upon oxidation of lipids that contain dienes or polyenes.[Citation30] shows the CD contents of the original SBO, WGO, and their blends under accelerated Schaal oven condition at 60°C.
Figure 2. Conjugated dienes values and TBARS values of SBO and its blends (top) and MHO and its blends (bottom) stored under Schaal oven condition at 60°C; SBO, original seal blubber oil; MHO, original menhaden oil; and WGO, original wheat germ oil.
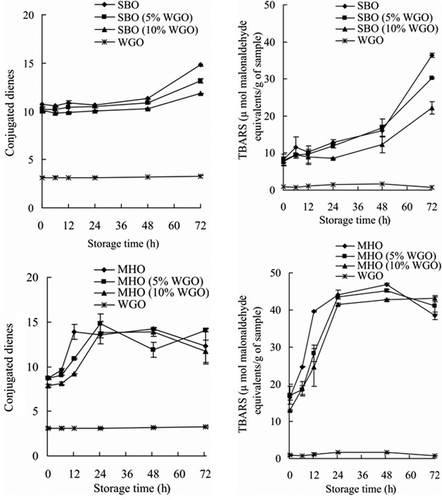
The CD values of both original SBO and its blends, SBO with 5% WGO and SBO with 10% WGO, increased throughout the entire experimental period due to the formation and accumulation of lipid hydroperoxides, which are the major primary oxidation products.[Citation3] Formation of lipid hydroperoxides coincides with that of CD upon oxidation.[Citation27,Citation31] CD values increased slowly from 0 to 48 h when primary oxidation products, hydroperoxides, were generated and released during lipid oxidation. However, the amount of hydroperoxide present was fairly low at the beginning of oxidation chain reaction. As the storage time was extended to 72 h, large amounts of primary oxidation products were produced due the abundance of free radicals, and this sharply increased CD values which reached the highest values at 72 h (). Values obtained at 0, 12, and 24 h did not show any significant difference between the original SBO and SBO with 5% WGO blend, while those of the latter were significantly lower when compared with those of the original SBO, indicating better oxidative stability of SBO with 5% WGO blend. The results showed significantly lower CD values at 6, 24, 48, and 72 h for SBO with 10% WGO blend when compared with those of the original SBO, while no significant difference existed at other test points. The CD values did not show any increase for the original WGO, which indicated its excellent stability under accelerated oxidation conditions. As the most important lipid-soluble antioxidants, tocopherols can effectively decrease lipid oxidation by scavenging free radicals produced from lipid peroxidation chain reactions, and thus may prevent the oxidation reaction from continuing.[Citation32,Citation33] The content of α-tocopherol by itself was more than 32.85 mg/100 g of WGO, and the total tocopherol content of WGO was 124 mg/100 g. The large amount of tocopherols helped WGO to maintain good oxidative stability under accelerated oxidation conditions as mixed tocopherols possess strong capacity in preventing lipid oxidation.[Citation34,Citation35] The changes of tocopherols content in WGO was monitored throughout the experimental period, and 4% of total tocopherols were lost at the 72 h test point (). Beta-, gamma-, and delta-tocopherols showed better stability than alpha-tocopherol during the accelerated oxidation, as the latter had a significantly (p < 0.05) higher loss (>4.5%) compared with the loss (<3.1%) of others, indicating its better sparing effect compared with those of other tocopherols in our system. Although the results from fatty acid composition analysis showed no significant changes in the total content of unsaturated fatty acids between the original SBO and its blends (), there was an increase of total PUFA content in the oil blends, due to a contribution from WGO, which may contribute to their high vulnerability to oxidation in the absence of introduced tocopherols from WHO. Thus, the improved oxidative stability of SBO with 5% WGO and SBO with 10% WGO blends was due to the increased tocopherols content contributed by the addition of WGO. The tocopherols content increased by 38% and 74% in SBO with 5% WGO and SBO with 10% WGO blends, respectively, compared with that in the original SBO ().
Figure 3. Changes of tocopherol contents in the original wheat germ oil stored under Schaal oven condition at 60°C.
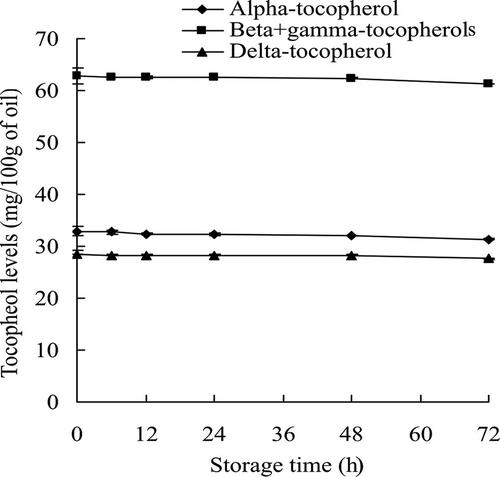
The CD values of MHO, MHO with 5% WGO, and MHO with 10% WGO monitored at each test point are shown in . The CD values of MHO with 5% WGO blend were significantly lower than those of MHO at 6, 12, and 48 h, but showed no significant difference at other test points. Significant difference existed in the CD values between MHO with 10% WGO blend and MHO at 0, 6, and 12 h, while no significant difference was observed at other test points. MHO with 5% WGO and MHO with 10% WGO blends showed a better oxidative stability when comparing their CD values with those of the original MHO. The results from fatty acid composition analysis did not reveal any significant changes in the total content of unsaturated fatty acids between the original MHO and its blends with WGO (). However, the total PUFA increased in the oil blends compared with that of the original MHO, which may lead to a lower stability. Therefore, the enhanced stability of MHO blends with WGO is mainly due to the increased content of tocopherols from the addition of WGO, as the total tocopherols increased to 14.68 and 20.42 mg/100 g of oil in MHO with 5% WGO and MHO with 10% WGO blends, respectively, while it was 8.92 mg/100 g of oil in the original WGO.
TBA test
The TBA test has commonly been employed to assess the level of secondary oxidation products. The rate of formation of secondary oxidation products is mainly affected by three factors, namely the amount of hydroperoxides as primary oxidation products, the level of tocopherols, and the nature of hydroperoxides which may be decomposed to secondary oxidation products at different rates under the same conditions.[Citation36] The type of the hydroperoxide is determined by the nature of fatty acid oxidised.
The results as shown in summarise TBARS values of SBO and its blends, which increased progressively until reaching their highest values during the storage period in both SBO and its blends, SBO with 5% WGO and SBO with 10% WGO. The TBARS values increased in a slow manner from 0 to 48 h, and started having sharp increase after 48 h and reached their highest values at 72 h ().[Citation37,Citation38] Meanwhile, the TBARS values of the original WGO were fairly low and did not show a sharp increase during the entire oxidation period, which coincided with the low CD values of this oil (). The low TBARS values of WGO indicate its high tocopherol content effectively prevented lipid oxidation. The results did show a significantly lower TBARS value for SBO with 5% WGO blend when compared with that of SBO at 72 h, while showing no significant difference at other test points. On the other hand, the TBARS values of SBO with 10% WGO blend were significantly lower than those of the original SBO at both 24 and 72 h, while no significant difference existed at other test points. Based on the results of TBARS, SBO with 5% WGO and SBO with 10% WGO blends were more stable than the original SBO due to increased tocopherols content although an increased total PUFA was observed in the oil blends.
The TBARS results obtained for MHO and its blends showed an increasing trend from 0 to 48 h, but decreased at 72 h (). The TBARS values of MHO with 5% WGO blend were significantly lower than those of the original MHO at 6, 12, and 48 h, but had no significant difference at other test points. MHO with 10% WGO blend had significantly lower TBARS values than MHO from 0 to 72 h, but showed a higher value at 72 h after reaching the peak value. Compared with the original MHO, a better oxidative stability of both MHO with 5% WGO and MHO with 10% WGO blends was demonstrated as shown by their lower TBARS values.
Conclusions
The oxidative stability of SBO and MHO was improved when blended with different levels of WGO, mainly due to the increased content of tocopherols, especially alpha-tocopherol. The effect of the increased PUFA content on the stability of oil blends was overcome by the effect of the increased tocopherol content. Therefore, WGO served as a good stabiliser for omega-3 marine oils with high contents of PUFAs.
Funding
Fereidoon Shahidi thanks the Natural Sciences and Engineering Research Council (NSERC) of Canada for providing financial support in the form of a discovery grant. The authors declare no conflict of interest.
Additional information
Funding
References
- Wanasundara, U.N.; Shahidi, F. Positional Distribution of Fatty Acids in Triacylglycerols of Seal Blubber Oil. Journal of Food Lipids 1997, 4, 51–64.
- Kim, I.; Hill, C.G., Jr. Lipase-Catalyzed Acidolysis of Menhaden Oil with Pinolenic Acid. Journal of the American Oil Chemists’ Society 2006, 83, 109–115.
- Hamam, F.; Shahidi, F. Synthesis of Structured Lipids via Acidolysis of Docosahexaenoic Acid Single Cell Oil (DHASCO) and Docosapentaenoic Acid (DPA). Journal of Agricultural and Food Chemistry 2004, 52, 2900–2906.
- Shahidi, F.; Ambigaipalan, P. Omega-3 Polyunsaturated Fatty Acids and their Health Benefits. Annual Reviews in Food Sciences and Technology 2018, 9(1). doi:10.1146/annurer-food-1113417-095850
- Denomme, J.; Stark, K.D.; Holub, B.J. Directly Quantitated Dietary (n-3) Fatty Acid Intakes of Pregnant Canadian Women are Lower than Current Dietary Recommendations. Journal of Nutrition 2005, 135, 206–211.
- Haag, M.;. Essential Fatty Acids and the Brain. Canadian Journal of Psychiatry 2003, 48, 195–203.
- Craig-Schmidt, M.C.; Stieh, K.E.; Lien, E.L. Retinal Fatty Acids of Piglets Fed Docosahexaenoic and Arachidonic Acids from Microbial Sources. Lipids 1996, 31, 53–59.
- Serhan, C.N.; Yang, R.; Martinod, K.; Kasuga, K.; Pillai, P.S.; Porter, T.F.; Oh, S.F.; Spite, M. Maresins: A Novel Macrophage Mediators with Potent Anti-Inflammatory and Proresolving Actions. Journal of Experimental Medicine 2008, 206, 13–23.
- Chen, Y.Q. Modulation of Prostate Cancer Genetic Risk by omega-3 and omega-6 Fatty Acids. Journal of Clinical Investigation 2007, 117, 1866–1875.
- Wang, C.C.; Harris, W.S.; Chung, M.; Lichtenstein, A.H.; Balk, E.M.; Kupelnick, B.; Jordan, H.S.; Lau, J. n−3 Fatty Acids from Fish or Fish-Oil Supplements, but Not Alpha-Linolenic Acid, Benefit Cardiovascular Disease Outcomes in Primary- and Secondary-Prevention Studies: A Systematic Review. American Journal of Clinical Nutrition 2006, 84, 5–17.
- Nemets, H.; Nemets, B.; Apter, A.; Bracha, Z.; Belmaker, R.H. omega-3 Treatment of Childhood Depression: A Controlled, Double-Blind Pilot Study. American Journal of Psychiatry 2006, 163, 1098–1100.
- Bousquet, M.; Saint-Pierre, M.; Julie, C.; Salem, N.; Cicchetti, F.; Calon, F. Beneficial Effects of Dietary omega-3 Polyunsaturated Fatty Acid on Toxin-Induced Neuronal Degeneration in an Animal Model of Parkinson’s Disease. Federation of American Societies for Experimental Biology 2008, 22, 1213–1225.
- Chandrasekara, B.; Troyer, D.A.; Venkatraman, J.T.; Fernandes, G. Dietary omega-3 Lipids Delay the Onset and Progression of Autoimmune Lupus Nephritis by Inhibiting Transforming Growth Factor Beta Mrna and Protein Expression. Journal of Autoimmunity 1995, 8, 381–393.
- Osterud, B.; Elveroll, E.; Barstad, H.; Brox, J.; Halvorsen, H.; Lia, K.; Olsen, J.L.; Olsen, R.L.; Sissense, C.; Rekdal, O.; Vognild, E. Effect of Marine Oils Supplementation on Coagulation and Cellular Activation in Whole Blood. Lipids 1995, 20, 1111–1118.
- Bimbo, A.P. Technology of Production and Industrial Utilization of Marine Oils. In Marine Biogenic Lipids, Fats and Oils; Ackman, R.G.; Ed.; CPC Press Inc.: Boca Raton, Florida, 1889; 401–433.
- Newton, I.S. Food Enrichment with Long-Chain N-3 PUFA. Food Technology 1996, 7, 169–177.
- Wang, T.; Johnson, L.A. Refining High-Free Fatty Acid Wheat Germ Oil. Journal of the American Oil Chemists’ Society 2001, 78, 71–76.
- Hargrove, J.L.; Greenspan, P.; Hartle, D.K. Nutritional Significance and Metabolism of Very Long Chain Fatty Slcohols and Acids from Dietary Waxes. Experimental Biology and Medicine 2004, 229, 215–226.
- Taylor, J.C.; Rapport, L.; Lockwood, G.B. Octacosanol in Human Health. Nutrition 2003, 19, 192–195.
- Chu, Y.; Kung, Y.A. A Study on Vegetable Oil Blends. Food Chemistry 1998, 62, 191–195.
- Harper, C.R.; Jacobson, T.A. Usefulness of omega-3 Fatty Acids and the Prevention of Coronary Heart Disease. American Journal of Cardiology 2005, 96, 1521–1529.
- Maguire, L.S.; O’Sullivan, S.M.; Galvin, K.; O’Connor, T.P.; O’Brien, N.M. Fatty Acid Profile, Tocopherol, Squalene, and Phytosterol Content of Walnuts, Almonds, Peanuts, Hazelnuts and the Macadamia Nut. International Journal of Food Science 2004, 55, 171–178.
- IUPAC. Standard Methods and Recommended Practices of the American Oil Chemists’ Society; 4th edn.; AOCS: Champaign, IL, 1987.
- AOCS. Official Methods and Recommended Practices of the American Oil Chemists’ Society; 4th edn.; AOCS: Champaign, IL, 1990.
- Wang, J.; Shahidi, F. Effect of Enzymatic Randomization on Positional Distribution and Stability of Seal Blubber and Menhaden Oils. Journal of Agricultural and Food Chemistry 2011, 59, 4232–4237.
- Molero G mez, A.; Mart nez de la Ossa, W. Quality of Wheat Germ Oil Extracted by Liquid and Supercritical Carbon Dioxide. Journal of the American Oil Chemists’ Society 2000, 77, 969–974.
- Hamam, F.; Shahidi, F. Acidolysis Reactions Lead to Esterification of Endogenous Tocopherols and Compromised Oxidative Stability of Modified Oils. Journal of Agricultural and Food Chemistry 2006, 54, 7319–7323.
- Wang, J.; Kralovec, E.R.S.J.; Shahidi, F. Effect of Chemical Randomization on Positional Distribution and Stability of omega-3 Oil Triacylglycerols. Journal of Agricultural and Food Chemistry 2010, 58, 8842–8847.
- Wanasundara, U.N.; Shahidi, F. Effects of Processing and Squalene on Composition and Oxidative Stability of Seal Blubber Oil. Journal of Food Lipids 1999, 6, 159–172.
- Logani, M.K.; Davies, R.E. Lipid Oxidation: Biologic Effects and Antioxidants-A Review. Lipids 1980, 15, 485–495.
- Farmer, E.H.; Sutton, D.A. Peroxidation in Relation to Olefinic Structure. Transactions of the Faraday Society 1946, 42, 228–232.
- Herrera, E.; Barbas, C. Vitamin E: Action, Metabolism and Perspectives. Journal of Physiology and Biochemistry 2001, 57, 43–56.
- Traber, M.G.; Atkinson, J. Vitamin E, Antioxidant and Nothing More. Free Radical Biology and Medicine 2007, 43, 4–15.
- Liu, M.; Wallin, R.; Wallmon, A.; Saldeen, T. Mixed Tocopherols Have a Stronger Inhibitory Effect on Lipid Peroxidation than Alpha-Tocopherol Alone. Journal of Cardiovascular Pharmacology 2002, 39, 714–721.
- Wagner, K.; Isnardy, B.; Elmadfa, I. γ- and δ-Tocopherols are More Effective than α-Tocopherol on the Autoxidation of a 10% Rapeseed Oil Triacylglycerol-In-Water Emulsion with and without a Radical Initiator. European. Journal of Lipid Science and Technology 2004, 106, 44–51.
- Nevdakh, O.P.; Agabekov, V.E.; Mitskevich, N.I. Kinetics of the Formation and Decomposition of Hydroperoxide in the Oxidation of 8-Pentadecanone. Reaction Kinetics and Catalysis Letters 1984, 27, 225–230.
- Strange, E.D.; Konstance, R.P.; Lu, D.; Smith, P.W.; Onwulata, C.I.; Holsinger, V.H. Oxidative and Functional Stability during Storage of Butter Oil Encapsulated with Sucrose or Flour. Journal of Food Lipids 1997, 4, 245–260.
- Senanayake, S.P.J.N.; Shahidi, F. Chemical and Stability of Structured Lipids from Borage (Borage officinalis L). And Evening Primrose (Oenothera biennis L.) Oils. Journal of Food Science 2001, 67, 2038–2052.