ABSTRACT
Oenological properties, volatile profiles, and sensory characteristics between Chinese Hong Qu rice wine (HQW) and Chinese Wheat Qu rice wine (WQW) were investigated. Forty and thirty-five volatile compounds in HQW and WQW were, respectively, identified. According to the door activity values (OAVs > 1), isoamyl acetate, ethyl octanoate, ethyl tetradecanoate, nonanal, and 2-methoxy-phenol were the major flavour contributors for HQW and WQW. Principal component analysis (PCA) showed that middle-chain fatty-acid (C6-C12) ethyl esters were the dominant representative flavour in HQW and WQW. Sensory evaluation indicated that the aroma of esters and alcohols differed largely between HQW and WQW. By partial least squares regression (PLSR), middle-chain fatty-acid-ethyl-esters (FAEEs) had a strong impact on the sensory attributes of fruit aroma, continuation, and full body in HQW and WQW.
Introduction
It is known that fermentation contributed to preserve and enhance the nutritional value of foods. As a traditional fermented alcoholic beverage, Chinese rice wine has been consumed for a long time all over the world, and it is widely used in medicine in the eastern-south of China.[Citation1] As a saccharification starter, “Qu” in Chinese rice wine provides nutrition for yeast multiplication and substances of flavour and aroma. Its role in Chinese rice wine is similar to the so-called koji of Japanese sake.[Citation2] In general, the quality of Chinese rice wine is directly related to the starters. Based on different saccharification starters (Qu), Chinese rice wine is mainly divided into Chinese Hong Qu rice wine (HQW; Hong Qu is made by extruded wheat and mainly fermented by Monascus) and Chinese Wheat Qu rice wine (WQW; Wheat Qu is made by wheat and purebred by Aspergillus). WQW exhibits a bright yellow color, subtle sweet flavour, and pleasant fragrance, and occupies a dominant market share in Chinese rice wine.[Citation3]HQW shows a bright red color, subtle sweet flavour, and multiple health-promoting functions due to the application of Hong Qu in the brewing process, which can significantly reduce the total cholesterol, low-density lipoprotein cholesterol, and total triacylglycerol concentrations.[Citation4,Citation5]Despite its unique appearance and specific health benefits, the development of HQW is still very slow for its lack of modern brewing technology and limited scientific research, compared with WQW.[Citation6] Thus, it is important to explore the difference between WQW and HQW during brewing.
Traditional HQW was successively produced by saccharification and fermentation stages. The brewing process mainly relied on empirical evaluation. Besides, the fermentation rate of traditional HQW was limited by the high initial glucose concentration generated from starch by saccharification. During the late fermentation stage, the inhibition effect of glucose on the activity of glucoamylase resulted in the weakening of secondary fermentation and prolonging the fermentation time.[Citation7] WQW was produced by simultaneous saccharification and fermentation, which could reduce energy consumption.[Citation8,Citation9] Besides, it had a simple brewing process and high fermentation rate. Although traditional manufacturing methods have been playing a significant role in HQW-making during the past few decades, the delay in obtaining real-time fermentation information and the instability of the HQW quality made manufacturers gradually pay more attention to the application of simple and easily controlled brewing technologies.[Citation10–Citation13] Therefore, comparing the different properties of HQW and WQW which are manufactured by simultaneous saccharification and fermentation is necessary.
Wine aroma is one of the most important factors governing the sensory perceivable wine quality and consumer preference. Meanwhile, the key aroma of Chinese rice wine mainly depends on the volatile compounds, which are produced by microbial metabolism. Thus, changes in volatile compounds and microorganisms during brewing need to be studied to improve the aroma quality of Chinese rice wine. A number of previous reports had studied intensively the microorganism diversity of HQW during fermentation[Citation14–Citation19] and the aroma profiles of WQW.[Citation20–Citation23] Nevertheless, articles on the specific aroma profiles in HQW are still very scarce. Studies on the quantification of volatile compounds in HQW during the brewing process are essential for the Chinese rice wine industry and are under investigation.
Therefore, the aim of this paper is to study the difference in the oenological property, volatile profile, and sensory characteristic between WQW and HQW produced by simultaneous saccharification and fermentation during different brewing stages. The volatile compounds of HQW and WQW in varying stages were monitored by gas chromatograph and mass spectrum (GC-MS). The important contributors to the overall aroma of wine are reflected by door activity values (OAVs). The characteristics of volatile profiles during wine brewing were analysed by carrying out PCA. The sensory characteristics of HQW and WQW were evaluated by descriptive sensory analysis. Partial least squares regression (PLSR) was performed to study the relationships between volatile aroma compounds and sensory attributes.
Materials and methods
Strain, starters, and chemicals
Saccharomyces cerevisiae BR 20, isolated in our lab with outstanding fermentation ability during spontaneous fermentation of rice wine, was used as the fermented strain. The BR 20 strain has been deposited in the China General Microbiological Culture Collection with accession number CGMCC 9445. “Hong Qu” was obtained from a company specialized in making Hong Qu (Fujian Gutian Diyuan Hong Qu Factory, Fujian Province, China). “Wheat Qu” came from a well-known local rice wine brewery (Shanghai Jinfeng wine Co., Ltd., Shanghai, China). Ethanol (special grade reagent) was purchased from China National Pharmaceutical Group Corporation (Shanghai, China). Ultrapure water was produced using a Milli-Q purification system (Milli-pore, Bedford, MA, USA). 4-Methyl-2-pentanol (99%) and other reagents were purchased from Sigma-Aldrich (Shanghai, China).
Preparation of yeast starter culture
One loop of 3-day-old slant cultures of S. cerevisiae BR 20 was inoculated into 50 mL malt extract broth in a 100-mL flask, and incubated under shaking (160 rpm) at 28°C for 18 h. The culture of BR 20 strain was then inoculated into malt broth with 15 Brix at a ratio of 10% (v/v) inoculum density and incubated under shaking (160 rpm) at 28°C for 2 d. The culture was used as a starter culture for the following experiments.
Winemaking
The brewing processes were designed as shown in . Glutinous rice was soaked in water for 24 h and Hong Qu was soaked for 12–16 h at 20°C. The soaked rice was steamed for 30 min, cooled at room temperature, and then transferred into a wide-mouth bottle with water, Qu, and yeast starter culture. The solution was mixed well and placed in an incubator at 28°C for 5 d, then migrated to an incubator at 20°C for another 15 days. The ratio of rice, Qu, yeast starter, and water was 10: 1: 1: 8. On the 20th day of fermentation, the wine mash was successively processed through filter pressing, clarification, and sterilization to yield the Chinese rice wine.
Sampling
Routine samples were obtained every three days during fermentation. Samples were respectively collected at the 20th day of fermentation, after clarification (CLE), and after sterilization (STE). The fermented wine mashes were centrifuged to obtain supernatants for analysis. All samples were stored at −20°C.
Determination of oenological property
Reducing sugar content in wine samples was determined by the dinitrosalicylic acid method.[Citation24] Total acidity (in terms of lactic acid) was measured by a China official method for the determination of total acidity.[Citation25] Ethanol content was quantified with a distillation method.[Citation26] To determine the amino acid nitrogen, 5 mL of wine sample and catalyser were digested at 420°C for 150 min and analysed by an automatic Kjeldahl apparatus (Kjeltec 8400, FOSS Co., Sweden).
Volatile flavour compounds analysis
Each of the wine sample (5 mL) was diluted with deionized water to a final concentration of 6% ethanol (v/v). The diluted samples were added into 20 mL headspace glass vials with 2 g of sodium chloride and 10 μL internal standard of 4-methyl-2-pentanol (250 μg/mL in absolute ethanol). The fibre (50 μm DVB/CAR/PDMS, SUPELCO Co., Bellefonte, PA, USA) headed into a solid phase microextraction (SPME) device (SUPELCO Co., Bellefonte, PA, USA) was inserted into the vials, then the vials were shaken at 50°C for 30 min to extract and absorb volatile compounds. The volatile compounds were then desorbed at 250°C into the GC inlet with an auto-sampler in 7 min. A GC-MS system (SCIONSQ-456, Bruker Daltonics Inc., USA) equipped with an HP-INNOWAX column (60 m × 0.25 mm × 0.25 μm, Agilent Technologies, USA) was programmed from 40°C (holding for 3 min) to 210°C at 6°C/min, then 210°C to 230°C at 8°C/min (holding for 15 min). Helium was delivered at a flow rate of 1 mL/min as the carrier gas. The mass detector was operated in electron impact mode at an ionizing voltage of 70 eV. The ion source temperature was set at 220°C.[Citation27] The extracted volatile compounds were identified by comparing the spectra with a mass spectrum library search (NIST 1.6 and Wiley 6.0). The Kovats index (KI) of unknown compounds was determined by sample injection with a homologous series of alkanes (C7–C30). The quantity of volatile compounds was determined by using the internal standard method. Positive identification was carried out by comparing the mass spectra and KIs of the authentic standards. Tentative identification was performed by comparing the mass spectra and KIs from the literature. Semiquantitative data of the volatile compounds were calculated according to the following formula:[Citation2]
where C is the relative concentration of the analysed sample, Cis is the final concentration of the internal standard in the sample, Ac is the peak area of the analysed sample, and Ais is the peak area of the internal standard.
OAV determination
OAV was calculated[Citation28] according to OAVi = Ci /OTi, where Ci is the concentration of the compound i in the sample and OTi is its door detection threshold concentration, which is found in the literature. Compounds with OAV > 1 are regarded as significant contributors to the overall aroma of Chinese rice wine as they are above their door threshold concentration.
Descriptive sensory analysis
The sensory evaluation of WQW and HQW was conducted by a panel of 10 judges (five males and five females), which had rich experiences on wine descriptive analysis. There was a uniform source of lightening, absence of noise, and distracting stimuli. Around 15-mL samples were presented in clear, tulip-shaped glasses marked with a random order and covered with petri dishes. A total of 11 descriptors of appearance (color and turbidity), aroma (alcohol, fruit, and cereal), taste/flavor (sweet, sour, and bitter), and mouthfeel (astringency, continuation, and full body) were selected by the panel during preliminary training sessions to describe the samples. Standards used to define the aroma and taste descriptor were included in the training and wine-tasting test. Intensity ratings were scored on a scale from 0 to 9 (0: none; 1–2: very weak; 3–4: ordinary; 5–6: moderate; 7–8: strong; 9: very strong).
Statistical analysis
Chemical analysis was carried out in triplicate for each wine sample, and the results were expressed as mean values ± standard deviations (SD). The mean differences were considered significant at p < 0.05. One-way ANOVA, followed by the Duncan test, was performed to analyse the significant differences between data. PCA using a correlation matrix with no rotation was performed to investigate the relationships between the different brewing stages of Chinese rice wines and volatile compounds. PLSR was used to explore the relationship between wine samples, volatile compounds, and sensory descriptors. SPSS ver. 19.0 (SPSS Inc., Chicago, IL, USA), Origin ver. 9.0 (Origin Lab Inc., Hampton, MS, USA), UNSCRAMBLER ver. 9.7 (CAMO ASA, Oslo, Norway), and Microsoft Office 2014 (Microsoft Corp., Redmond, WA, USA) were used to deal with the GC-MS and chemical analysis data (all of the GC-MS data had been conveyed by the diluted factor).
Results and discussions
Oenological properties of HQW and WQW during different brewing stages
The oenological properties of HQW and WQW during the different brewing stages (percentage of ethanol by volume, reducing sugar, total acidity, and amino acid nitrogen) are shown in (little change was observed between the “20 d” wine samples and the “CLE” samples). In general, all oenological parameters evaluated were in the ranges established by China regulations for Chinese rice wine.[Citation26] Although the curves belonging to the two wines showed similar profiles, which were typical of Chinese rice wine fermentation, differences in the fermentation performance between HQW and WQW were significant. During fermentation, starch was transformed to ethanol by the saccharification enzymes of starters (Qu) and the fermentation of yeast. Thus, the contents of reducing sugar in both HQW and WQW were sharply decreased to dryness (reducing sugar content was below 15 g/L) in the first five days (primary fermentation), and slowly decreased in the secondary fermentation. However, ethanol content showed a large increase during fermentation and slightly declined after clarification and sterilization in HQW and WQW. After fermentation, both HQW and WQW possessed a considerable level of ethanol (17.69%, v/v and 18.10%, v/v, respectively), in comparison with the previous studies of Chinese rice wine.[Citation23,Citation29] WQW exhibited a higher descent rate of reducing sugar and growth rate of ethanol than HQW. As for total acidity, it basically increased with the acid metabolites, which mainly came from yeast, bacteria, and mold during fermentation. Total acidity of WQW and HQW were progressively increased during fermentation, while WQW exhibited higher total acidity than that of HQW. Amino acid nitrogen is a minor constituent group in wine; however, it greatly contributes to the quality of the product and, in fact, it is responsible for the “body” sensation.[Citation30] The amino acid nitrogen content determined in this study was higher than those detected by other researchers.[Citation29,Citation31] Because of the hydrolysis of protein in rice and the autolysis of yeast cells, a steadily rising trend was observed in amino acid nitrogen during fermentation. The content of amino acid nitrogen in HQW was higher than that of WQW. After clarification and sterilization, there was no significant change in the concentration of reducing sugar, total acidity, and amino acid nitrogen. However, continuous decrease occurred for the ethanol content in both WQW and HQW. WQW exhibited a higher saccharification rate; however, there was a lower concentration of amino acid nitrogen in contrast to HQW. Aspergillus purebred breeding of Wheat Qu and the functional constituent of Hong Qu might be responsible for it.
Identification and determination of volatile flavour compounds in HQW and WQW during different brewing stages
The volatile flavour compounds strongly influenced the typical taste and door of Chinese rice wine.[Citation15,Citation23] A total of 40 and 35 compounds were determined in HQW and WQW during brewing (), respectively. GC-MS data of the third day and twelfth day representing the primary fermentation and secondary fermentation are also listed, respectively. Although the aroma compounds contents of the sixth day and the ninth day both revealed slowly increasing tendency, there was no further change after 12 days of fermentation. The volatile aroma compounds during different brewing stages were significantly different between the HQW and WQW samples (p < 0.05). Among all the volatile compounds determined, 32 compounds were positively identified through the comparison of mass spectral data with those of authentic standards, and another eight compounds were tentatively identified through the comparison of mass spectral data with a database and the literature. These compounds in HQW belonged to different chemical groups: esters (24), alcohols (nine), acids (one), aldehydes (four), and volatile phenols (two). However, there were 20 esters, eight alcohols, four aldehydes, two phenols, and one acid in WQW. The main difference between HQW and WQW is in the numbers of esters and alcohols, especially esters.
Table 1. Identification and relative contents of volatile compounds during different brewing stages of HQW and WQW analysed by SPME-GC-MS. (n = 3).
The OAV is an important factor reflecting the contribution of individual volatile compounds to the overall aroma of wine.[Citation32,Citation33] It has been accepted that volatile compound with concentration above its door threshold (OAV > 1) could significantly contribute its flavour notes to the overall aroma of wine.[Citation34,Citation35] OAVs were calculated using the results from GC-MS analysis and a list of their aroma descriptors is provided in supplemental Table S2. A total of 14 volatile compounds with OAV > 1 in HQW and WQW after sterilization were found in the present study, including two alcohols, nine esters, two aldehydes, and one phenol. Normally, the aroma-active compounds with higher OAVs most likely became the major contributors to the overall aroma of Chinese rice wine, although aroma synergy or suppression was possible when some species coexisted.[Citation36] In HQW, nonanal (green, slightly pungent) had the highest average OAV (560), followed by isoamyl acetate (banana, 383) and 2-methoxy-phenol (phenolic, 93). However, in WQW, isoamyl acetate exhibited the highest OAV (477), followed by nonanal (360) and ethyl octanoate (pineapple, pear, floral, 306). The OAVs of isoamyl acetate, ethyl octanoate, ethyl tetradecanoate, nonanal, and 2-methoxy-phenol were significantly different between HQW and WQW, suggesting that these compounds could be the major odorants that resulted in the different flavour between HQW and WQW.
Esters occupied the largest number of overall volatile compounds during different brewing stages, followed by alcohols and aldehydes. Within the esters, most of them were ethyl esters. Ethyl esters were formed through enzymatic and chemical esterification of fatty acids interacting with ethanol during fermentation and the long-term aging process.[Citation37–Citation39] Among ethyl esters, the major compounds determined were ethyl acetate, ethyl-2-hydroxypropanoate, ethyl octanoate, ethyl benzoate, diethyl succinate, ethyl tetradecanoate, ethyl hexadecanoate, (Z)-9-octadecenoic acid ethyl ester, and linoleic acid ethyl ester in HQW and WQW. Among the alcohols, 2-phenylethanol, 2-methyl-1-propanol, and 3-methyl-1-butanol exhibited relatively higher contents, which accounted for 90.46% of the total quantity of alcohols during brewing. With regard to aldehydes, benzaldehyde was the predominant compound, which accounted for nearly 85% of the total aldehydes content in both HQW and WQW during brewing. This compound was responsible for the almond flavour of wines. Minor compounds were also important contributors to wine aroma, as in the case of phenols, which contribute to the diversity and complexity of wine.[Citation1] The main phenol was 2-methoxy-phenol, whose OAV was >1 in both HQW and WQW. Other significant compounds found, such as acids, which exist in a small portion, are also important to wine aroma. Chinese verb goes: “no acid, no flavour”, whereas most acids were nonvolatile organic acids produced by the microbes.[Citation2] Only acetic acid was detected by the equipment.
Changes of major volatile compounds of HQW and WQW during different brewing stages
The total contents for major volatile compounds (alcohols, esters, and aldehydes) and the related changes in WQW and HQW during different brewing stages are shown in and . In terms of alcohols, the total content of alcohols in manufactured WQW (59.85 mg/L) was significantly higher than that of manufactured HQW (62.36 mg/L). Alcohols were mainly generated by yeast metabolism; thus the content of alcohols in both WQW and HQW experienced a progressive increase (0–63.61 mg/L in HQW and 0–65.21 mg/L in WQW) during fermentation and a decline after clarification and sterilization (63.61–59.85 mg/L in HQW and 65.21–62.36 mg/L in WQW) (). Alcohols identified in the present study were all higher alcohols, which were secondary natural products of alcoholic beverages.[Citation40] However, excessive content of higher alcohols in wine might cause dizziness, and much attention has been paid to its control.[Citation41] Unlike 2-phenylethanol, which has a floral scent, and is a standard for judging the quality of Chinese rice wine, 3-methyl-1-butanol and 2-methyl-1-butanol in high concentrations possessed fusel-like doors, which need to be controlled.[Citation42,Citation43] However, moderate concentrations of higher alcohols contribute to the aromatic complexity of the wine. The contents of 2-phenylethanol, 3-methyl-1-butanol, and 2-methyl-1-butanol in WQW were all higher than that of HQW.
Figure 3. Changes of alcohols (a), aldehydes (b), short-chain FAEE (c), middle-chain FAEE, (d) and long-chain FAEE (e) of HQW and WQW during different brewing stages. HQW and WQW during different brewing stages (n = 3, * 0.01<p < 0.05, ** p < 0.01).
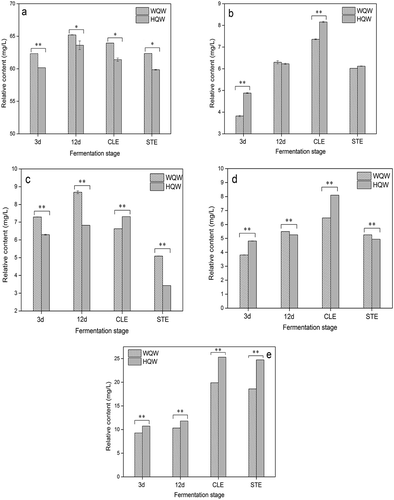
The content of esters detected in manufactured HQW (35.06 mg/L) was significantly higher than in WQW (30.21 mg/L). Within the esters, most of them were fatty-acid-ethyl-esters (FAEEs). Those compounds were mainly produced during alcoholic fermentation by yeast, in reactions between alcohols and acetyl-CoA, and contribute to the fruity and flowery character of wine.[Citation44] FAEE of short chain (C2-C5), middle chain (C6-C12) and long chain (C13-C18) experienced a steady rise in both HQW and WQW during fermentation (–). After clarification, middle-chain FAEE content increased by 17.64% and 54.28% in WQW and HQW, respectively. Long-chain FAEE content had mass increase of 92.64% and 114.14% in WQW and HQW, respectively. Short-chain FAEE content decreased by 31.07% in WQW; however, it increased by 7.18% in HQW. Following sterilization, sharp decreases occurred in the content of short-chain FAEE and middle-chain FAEE; however, it had little effect on the content of long-chain FAEE (fluctuating from 19.9 mg/L to 18.6 mg/L in WQW and 25.29 mg/L to 24.72 mg/L in HQW). This might be on account of the disparity of their chemical groups. Moreover, HQW had a higher content of middle-chain and long-chain FAEE than WQW, whereas WQW exhibited a higher content of short-chain FAEE.
With regard to the aldehydes, the sum of aldehydes in the manufactured HQW (6.12 mg/L) was a little higher than that of WQW (6.02 mg/L). During fermentation and after clarification in both HQW and WQW, the aldehydes content showed a sharp increase. By way of sterilization, great loss was observed in both wines (falling from 7.36 mg/L to 6.02 mg/L in WQW and 8.16 mg/L to 6.12 mg/L in HQW). Benzaldehyde, as it occupied the highest portion of the total aldehydes, the concentration of which decreased by 30.7% and 21.4% in HQW and WQW after sterilization, was responsible for the great loss.
The total contents of alcohols, FAEE, and aldehydes in both HQW and WQW sharply increased in the primary fermentation and kept slightly increasing during the secondary fermentation. According to the analysis above, significant changes in volatile compounds were observed in WQW and HQW during fermentation. After sterilization, a great loss of volatile compounds occurred in both wines. The rice wine brewed with Hong Qu exhibited a richer quantity of esters, especially the long-chain FAEE, and less content of alcohols than WQW, whereas the concentration of aldehydes concentrations in the two wines was similar.
Characteristic of volatile flavour compounds in HQW and WQW during different brewing stages
PCA was carried out to determine the characteristic of the volatiles in the two wines, using the concentrations of the volatile compounds in the HQW and WQW during different brewing stages as analytical variables. Generally, the loadings show the relative importance of each individual volatile compound and the distribution of the different volatile compounds will reflect the differences observed between HQW and WQW during different brewing stages.[Citation22] The results of PCA shown in and revealed the association between the volatile compounds in HQW and WQW during the different brewing stages, respectively.
Figure 4. Variables plot for 40 volatile compounds (a) and scores (b) for the different brewing stages of HQW by PCA.
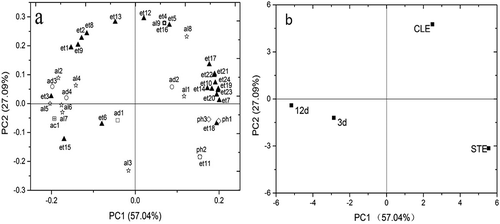
Figure 5. Variables plot for 35 volatile compounds (a) and scores (b) for the different brewing stages of WQW by PCA.
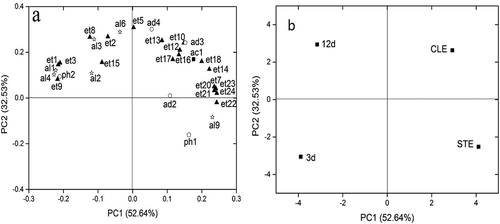
As shown in , it provided a bi-plot showing the loading plots as well as the score plots of HQW, whereby the first and second principal components (PC 1 and PC 2) explained 57.04% and 27.09% of the total variance, which reflected 84.13% of the total flavour information in HQW during brewing (). The positive axis of PC 1 was highly influenced by diethyl succinate, ethyl-2-hydroxypropanoate, ethyl tetradecanoate, ethyl hexadecanoate, ethyl heptadecanoate, ethyl(9E)-9-octadecenoate, (Z)-9-octadecenoic acid ethyl ester, linoleic acid ethyl ester, γ-nonanolactone, and 2-methoxy-phenol. These compounds exhibited a significant increase in concentration after clarification. In addition, the positive axis of PC 2 was highly related with ethyl decanoate and ethyl benzoate, which were also found in the highest concentration after clarification. The relationship in different volatile compounds of WQW was showed in , which PC1 explained 52.64% of the overall variance with ethyl hexadecanoate, ethyl heptadecanoate, ethyl(9E)-9-octadecenoate, (Z)-9-octadecenoic acid ethyl ester, and linoleic acid ethyl ester had the strongest effect on its positive side. Specifically, these compounds increased greatly after clarification. In addition, PC 2 accounted for 32.53% of the variance while ethyl hexanoate, ethyl octanoate, 1-hexanol, and 4-methyl-benzaldehyde were its greatest contributors. However, these compounds exist in trace amounts except for ethyl octanoate.
Based on , it seemed difficult to properly discriminate between the aroma of wine during the primary fermentation and secondary fermentation in HQW. However, the results of PCA shown in revealed a good separation of the different brewing stages in WQW. Both in the HQW and WQW, the aroma profiles after clarification had the highest score, which represented the volatile flavour characteristic of all the brewing stages in Chinese rice wines. The flavour of HQW after clarification was closely associated with 1-octanol, 2-phenylethanol, ethyl hexanoate, and 2-phenethylacetate ethyl valerate. However, the flavour of WQW after clarification was characterized by higher contents of ethyl2-hydroxy-4-methylvalerate, ethyl decanoate, ethyl dodecanoate, 2-phenethylacetate, γ-nonanolactone, benzaldehyde, and acetic acid.
Although the substances closely associated with the two wines after clarification were not identical, middle-chain FAEE were the important substances of volatile compounds after clarification in both HQW and WQW. Moreover, long-chain FAEE, which showed a significant effect on the volatile compounds, exhibited a great increase after clarification in both wines.
Sensory evaluation
To profile the sensory characteristics of HQW and WQW, descriptive sensory analysis was conducted. The mean intensity ratings of the two wines are presented in . HQW exhibited high levels of redness while WQW seemed to have a high level of yellowness. The attribute levels of sweet flavour, sour flavour, and astringency mouthfeel were generally similar in the two wines. HQW showed a bit higher level of bitter flavour and continuation mouthfeel than WQW. HQW was intense in fruit aroma, cereal aroma, and full body mouthfeel, whereas WQW exhibited high levels of alcohol aroma. In contrast to WQW, the higher intensity in aroma of fruity and cereal and lower alcohol-aroma intensity in HQW were in agreement with the experimental results in . These results indicated that both HQW and WQW had their own flavour characteristics; the discrimination mainly focused on the aroma of esters and alcohols. Meanwhile, HQW presented a more mellow taste while WQW exhibited more alcohol aroma.
Relationship between sensory attributes and volatile compounds
To study the relationships between sensory attributes and volatile compounds, multivariate PLSR was performed. All volatile compounds after sterilization were designed as independent variables and nine sensory data (fruit aroma, cereal aroma, alcohol aroma, sweet, astringency, sour, bitter, full body, and continuation) were designed as dependent variables. PLSR modelling between the matrices of volatile compounds and sensory attributes provided a two-factor model explaining 100% of the variance in X (volatile compounds) and 76% of that in Y (sensory attributes). The small ellipse indicated 50% of the explained variance and the big ellipse is the unit-circle indicating 100% of the explained variance.[Citation45,Citation46] It was entailed that aroma compounds between the two ellipses can be considered as correlated with sensory attributes, and those inside the inner ellipse are poorly connected with sensory attributes.
The resultant correlation loading plot of the first two principal components shown in indicated that almost all of the 40 compounds and nine sensory attributes were located between the small and big ellipses, except for the attributes of sweet and astringency, ethyl butanoate, ethyl decanoate, ethyl 4-hydroxybutanoate, γ-nonanolactone, and (Z)-9-octadecenoic acid ethyl ester, indicating they were well explained by the PLSR model. The PC1 axis showed a contrast between fruit aroma, full body, continuation, sour, and bitter on the positive dimension and alcohol aroma in the negative dimension. The continuation was strongly correlated to ethyl hexanoate, which had a high OAV. Fruit aroma and full body were highly associated with ethyl-2-hydroxypropanoate, diethyl succinate, ethyl benzoate, and ethyl hexanoate. Cereal aroma was significantly correlated with ethyl hexadecanoate, ethyl heptadecanoate, ethyl (9E)-9-octadecenoate, linoleic acid ethyl ester, 1-propanol, nonanal, 2-methoxy-phenol, and 2-methoxy-4-vinylphenol. Ethyl acetate, isoamyl acetate, ethyl 2-hydroxy-4-methyvalerate, benzaldehyde, 2-methyl-1-butanol, and 1-hexanol showed a significant contribution to alcohol aroma. PLSR revealed that middle-chain FAEE (C6-C12) had a strong impact on the sensory attributes of fruit aroma, continuation, and full body, which was in agreement with the results of PCA analysis.
Conclusion
Since HQW possesses a number of health-promoting functions, it also exhibited a richer quantity of esters, especially the long-chain FAEE than WQW. Using PCA, we found middle-chain FAEEs were the representative flavour in HQW and WQW. Moreover, long-chain FAEE also showed a significant effect on the volatile compounds in both wines. Sensory descriptive evaluation indicated that HQW presented a mellow taste while WQW exhibited a more alcohol aroma. PLSR also revealed that middle-chain FAEE had a strong impact on the sensory attributes of fruit aroma, continuation, and full body. A significant loss of volatile compounds occurred in both wines after sterilization, which means new sterilization methods are in urgently required. In addition, through further studies on the correlation between wine flavour and the major microbes, our work may have implications for figuring out the correlation between microorganisms and wine flavour during brewing.
LJFP_A_1325900_Supplemental_Table_2.doc
Download MS Word (88 KB)Funding
This research work was financially supported by the Capacity building project of Shanghai (15150502400), the project of industry transformation and upgrading of Shanghai (CXY-2016-016), the Shanghai Natural Science Fund (15ZR1428900), and the Graduate student innovation fund project of Shanghai (JWCXSL1402).
Supplemental data
Supplemental data for this article can be accessed on the publisher's website.
Additional information
Funding
References
- Lu, Q.Y.; Lee, R.P.; Huang, J.J.; Yang, J.P.; Henning, S.M.; Hong, X.T.; Heber, D.; Li, Z.P.; Quantification of Bioactive Constituents and Antioxidant Activity of Chinese Yellow Wine. Journal of Food Composition and Analysis. 2015, 44, 86–92.
- Mo, X.L.; Fan, W.L.; Xu, Y.; Changes in Volatile Compounds of Chinese Rice Wine Wheat Qu during Fermentation and Storage. Journal of the Institute of Brewing. 2009, 115(4), 300–307.
- Mo, X.L.; Xu, Y.; Fan, W.L.; Characterization of Aroma Compounds in Chinese Rice Wine Qu by Solvent-Assisted Flavor Evaporation and Headspace Solid-Phase Microextraction. Journal of Agriculture and Food Chemistry. 2010, 58(4), 2462–2469.
- Lv, X.C.; Cai, Q.Q.; Ke, X.X.; Chen, F.; Rao, P.F.; Ni, L.; Characterization of Fungal Community and Dynamics during the Traditional Brewing of Wuyi Hong Qu Glutinous Rice Wine by Means of Multiple Culture-Independent Methods. Food Control. 2015, 54, 231–239.
- Kim, C.M.; Yi, S.J.; Cho, I.J.; Ku, S.K.; Red-Koji Fermented Red Ginseng Ameliorates High Fat Diet-Induced Metabolic Disorders in Mice. Nutrients. 2013, 5(11), 4316–4332.
- Huang, Z.Q.; Zheng, C.Y.; Gong, L.Q.; Chen, Y.X.; Ni, L.; Present Situations and Development Strategies of Fujian Hong Starter Yellow Rice Wine. Liquor-Making Science and Technology. 2013, 7, 113–116.
- Wu, Q.; Chen, B.; Xu, Y.; Regulating Yeast Flavor Metabolism by Controlling Saccharification Reaction Rate in Simultaneous Saccharification and Fermentation of Chinese Maotai-Flavor Liquor. International Journal of Food Microbiology. 2015, 200, 39–46.
- Cao, Y.; Xie, G.; Wu, F.; Lu, C.; J. A Study on Characteristic Flavor Compounds in Traditional Chinese Rice Wine: Guyue Longshan Rice Wine. Journal of the Institute of Brewing 2010, 116(2), 182–189.
- Wang, P.X.; Mao, J.; Meng, X.Y.; Li, X.Z.; Liu, Y.Y.; Feng, H.; Changes in Flavour Characteristics and Bacterial Diversity during the Traditional Fermentation of Chinese Rice Wines from Shaoxing Region. Food Control. 2014, 44, 58–63.
- Zhao, J.; Xia, L.M.; Simultaneous Saccharification and Fermentation of Alkaline-Pretreated Corn Stover to Ethanol Using a Recombinant Yeast Strain. Fuel Processing Technology. 2009, 90(10), 1193–1197.
- Xu, E.B.; Wu, Z.Z.; Long, J.; Wang, F.; Xu, X.M.; Jin, Z.Y.; Jiao, A.Q.; Improved Bioaccessibility of Phenolics and Antioxidant Activity of Glutinous Rice and Its Fermented Chinese Rice Wine by Simultaneous Extrusion and Enzymatic Hydrolysis. Journal of Function Foods. 2015, 17, 214–226.
- Wu, Z.Z.; Xu, E.B.; Long, J.; Zhang, Y.J.; Wang, F.; Xu, X.M.; Jin, Z.Y.; Jiao, A.Q.; Monitoring of Fermentation Process Parameters of Chinese Rice Wine Using Attenuated Total Reflectance Mid-Infrared Spectroscopy. Food Control. 2015, 50, 405–412.
- Xu, E.B.; Long, J.; Wu, Z.Z.; Li, H.Y.; Wang, F.; Xu, X.M.; Jin, Z.Y.; Jiao, A.Q.; Characterization of Volatile Flavor Compounds in Chinese Rice Wine Fermented from Enzymatic Extruded Rice. Journal of Food Science. 2015, 80(7), C1476–C1489.
- Lv, X.C.; Jia, R.B.; Li, Y.; Chen, F.; Chen, Z.C.; Liu, B.; Chen, S.J.; Rao, P.F.; Ni, L.; Characterization of the Dominant Bacterial Communities of Traditional Fermentation Starters for Hong Qu Glutinous Rice Wine by Means of MALDI-TOF Mass Spectrometry Fingerprinting, 16S Rrna Gene Sequencing and Species-Specific Pcrs. Food Control. 2016, 67, 292–302.
- Zheng, J.; Liang, R.; Zhang, L.Q.; Wu, C.D.; Zhou, R.Q.; Liao, X.P.; Characterization of Microbial Communities in Strong Aromatic Liquor Fermentation Pit Muds of Different Ages Assessed by Combined DGGE and PLFA Analyses. Food Research International. 2013, 54(1), 660–666.
- Lv, X.C.; Huang, R.L.; Chen, F.; Zhang, W.; Rao, P.F.; Ni, L.; Bacterial Community Dynamics during the Traditional Brewing of Wuyi Hong Qu Glutinous Rice Wine as Determined by Culture-Independent Methods. Food Control. 2013, 34(2), 300–306.
- Lv, X.C.; Huang, X.L.; Zhang, W.; Rao, P.F.; Ni, L.; Yeast Diversity of Traditional Alcohol Fermentation Starters for Hong Qu Glutinous Rice Wine Brewing, Revealed by Culture-Dependent and Culture-Independent Methods. Food Control. 2013, 34(1), 183–190.
- Lv, X.C.; Huang, Z.Q.; Zhang, W.; Rao, P.F.; Ni, L.; Identification and Characterization of Filamentous Fungi Isolated from Fermentation Starters for Hong Qu Glutinous Rice Wine Brewing. The Journal of General and Applied Microbiology. 2012, 58(1), 33–42.
- Lv, X.C.; Weng, X.; Zhang, W.; Rao, P.F.; Ni, L.; Microbial Diversity of Traditional Fermentation Starters for Hong Qu Glutinous Rice Wine as Determined by PCR-Mediated DGGE. Food Control. 2012, 28(2), 426–434.
- Welke, J.E.; Zanus, M.; Lazzarotto, M.; Alcaraz Zini, C.; Quantitative Analysis of Headspace Volatile Compounds Using Comprehensive Two-Dimensional Gas Chromatography and Their Contribution to the Aroma of Chardonnay Wine. Food Research International. 2014, 59, 85–99.
- Zheng, C.Y.; Gong, L.T.; Huang, Z.Q.; Liu, Z.B.; Zhang, W.; Ni, L.; Analysis of the Key Volatile Aroma Compounds in Sweet Hongqu Glutinous Rice Wine. Journal of Chinese Institute of Food Science and Technology. 2014, 14(5), 209–214.
- Luo, T.; Fan, W.L.; Xu, Y.; Characterization of Volatile and Semi-Volatile Compounds in Chinese Rice Wines by Headspace Solid Phase Microextraction Followed by Gas Chromatography-Mass Spectrometry. Journal of the Institute of Brewing. 2008, 114(2), 172–179.
- Tian, Y.T.; Huang, J.M.; Xie, T.T.; Huang, L.Q.; Zhuang, W.J.; Zheng, Y.F.; Zheng, B.D.; Oenological Characteristics, Amino Acids and Volatile Profiles of Hongqu Rice Wines during Pottery Storage: Effects of High Hydrostatic Pressure Processing. Food Chemistry. 2016, 203, 456–464.
- Miller, G.;; Use of Dinitrosalicylic Acid Reagent for Determination of Reducing Sugar. Analytical Chemistry. 1959, 31(3), 426–428.
- GB/T 5517; 2010. Official Methods of Analysis; Determination of Total Acidity: Beijing, China.
- GB/T 13662; 2008. Chinese Rice Wine; Beijing, China.
- Jung, H.; Lee, S.J.; Lim, J.H.; Kim, B.K.; Park, K.J.; Chemical and Sensory Profiles of Makgeolli, Korean Commercial Rice Wine, from Descriptive, Chemical, and Volatile Compound Analyses. Food Chemistry. 2014, 152, 624–632.
- Yilmaztekin, M.;; Characterization of Potent Aroma Compounds of Cape Gooseberry (Physalis peruvianal.) Fruits Grown in Antalya through the Determination of Odor Activity Values. International Journal of Food Properties 2013, 17(3), 469–480.
- Wu, Z.Z.; Xu, E.B.; Long, J.; Wang, F.; Xu, X.M.; Jin, Z.Y.; Jiao, A.Q.; Measurement of Fermentation Parameters of Chinese Rice Wine Using Raman Spectroscopy Combined with Linear and Non-Linear Regression Methods. Food Control. 2015, 56, 95–102.
- Caliari, V.; Burin, V.M.; Rosier, J.P.; BordignonLuiz, M.T.; Aromatic Profile of Brazilian Sparkling Wines Produced with Classical and Innovative Grape Varieties. Food Research International. 2014, 62, 965–973.
- Pan, H.Q.; Cao, Y.; Shi, H.Y.; Lu, J.; Xie, G.F.; Effect of Post-Fermentation Technology on Amino Acid Nitrogen Generating during Chinese Rice Wine Brewing. Journal of Food Science and Biotechnology. 2016, 35(2), 144–150.
- Ouyang, X.Y.; Yuan, G.S.; Ren, J.; Wang, L.Y.; Wang, M.Z.; Li, Y.H.; Zhang, B.L.; Zhu, B.Q.; Aromatic Compounds and Organoleptic Features of Fermented Wolfberry Wine: Effects of Maceration Time. International Journal of Food Properties 2016. doi:10.1080/10942912.2016.1233435.
- Wang, L.; Hu, G.Y.; Lei, L.B.; Lin, L.; Wang, D.Q.; Wu, J.X.; Identification and Aroma Impact of Volatile Terpenes in Moutai Liquor. International Journal of Food Properties 2015. doi:10.1080/10942912.2015.1064442.
- Noguerol-Pato, R.; González-Álvarez, M.; González-Barreiro, C.; Cancho-Grande, B.; Simal-Gándara, J.; Aroma Profile of Garnacha Tintorera-Based Sweet Wines by Chromatographic and Sensorial Analyses. Food Chemistry. 2012, 134(4), 2313–2325.
- Cai, J.; Zhu, B.Q.; Wang, Y.H.; Lu, L.; Lan, Y.B.; Reeves, M.J.; Duan, C.Q.; Influence of Pre-Fermentation Cold Maceration Treatment on Aroma Compounds of Cabernet Sauvignon Wines Fermented in Different Industrial Scale Fermenters. Food Chemistry. 2014, 154, 217–229.
- Feng, Y.Z.; Su, G.W.; Zhao, H.F.; Cai, Y.; Cui, C.; Sun- Waterhouse, D.X.; Zhao, M.M.; Characterisation of Aroma Profiles of Commercial Soy Sauce by Odour Activity Value and Omission Test. Food Chemistry. 2014, 167, 220–228.
- Yu, H.Y.; Dai, X.; Yao, G.Y.; Xiao, Z.; Application of Gas Chromatography-Based Electronic Nose for Classification of Chinese Rice Wine by Wine Age. Food Analytical Methods. 2013, 7(7), 1489–1497.
- Domizio, P.; Manazzu, I.; Ciani, M.; Impact of Mother Sediment on Yeast Growth, Biodiversity, and Ethanol Production during Fermentation of Vinsanto Wine. International Journal of Food Microbiology. 2009, 129(1), 83–87.
- Cheong, K.W.; Tan, C.P.; Mirhosseini, H.; Hamid, N.S.A.; Osman, A.; Basri, M.; Equilibrium Headspace Analysis of Volatile Flavor Compounds Extracted from Soursop (Annona muricata) Using Solid-Phase Microextraction. Food Research International. 2010, 43(5), 1267–1276.
- Romano, P.; Suzzi, G.; Comi, G.; Zironi, R.; Higher Alcohol and Acetic Acid Production by Apiculate Wine Yeasts. Journal of Applied Microbiology. 1992, 73, 126–130.
- Zhou, J.D.; Jiang, Y.J.; Zou, H.J.; Tan, L.H.; Shen, B.; Changes of Primary Higher Alcohols during the Traditional and New Rice Wine Fermentation Process. China Brewing. 2012, 31(8), 29–32.
- Chen, S.; Luo, T.; Xu, Y.; Fan, W.L.; Zhao, G.A.; Effects of Yeast Strains and Raw Materials on Β-Phenylethanol Production in Chinese Rice Wines. China Brewing. 2009, 4, 23–26.
- Callejon, R.; Clavijo, A.; Ortigueira, P.; Troncoso, A.; Paneque, P.; Morales, M.; Volatile and Sensory Profile of Organic Red Wines Produced by Different Selected Autochthonous and Commercial Saccharomyces cerevisiae Strains. Analytical Chimica Acta. 2010, 660(1–2), 68–75.
- Ubeda, C.; Callejón, R.M.; Troncoso, A.M.; Peña-Neira, A.; Morales, M.L.; Volatile Profile Characterisation of Chilean Sparkling Wines Produced by Traditional and Charmat Methods via Sequential Stir Bar Sorptive Extraction. Food Chemistry. 2016, 207, 261–271.
- Xiao, Z.B.; Yu, D.; Niu, Y.W.; Chen, F.; Song, S.Q.; Zhu, J.C.; Zhu, G.Y.; Characterization of Aroma Compounds of Chinese Famous Liquors by Gas Chromatography–Mass Spectrometry and Flash GC Electronic-Nose. Journal of Chromatography B 2014, 945–946, 92–100.
- Tian, H.X.; Shen, Y.B.; Yu, H.Y.; Chen, C.; Aroma Features of Honey Measured by Sensory Evaluation, Gas Chromatography-Mass Spectrometry and Electronic Nose. International Journal of Food Properties 2016. doi:10.1080/10942912.2016.1213744.
- Sally Rahayu, Y.Y.; Yoshizaki, Y.; Yamaguchi, K.; Okutsu, K.; Futagami, T.; Tamaki, H.; Sameshima, Y.; Takamine, K.; Key Volatile Compounds in Red Koji-Shochu, a Monascus-Fermented Product, and Their Formation Steps during Fermentation. Food Chemistry. 2017, 224, 398–406.
- Ferreira, V.; Ortin, N.; Escudero, A.; Lopez, R.; Cacho, J.F.; Chemical Characterization of the Aroma of Grenache Rose Wines: Aroma Extract Dilution Analysis, Quantitative Determination, and Sensory Reconstitution Studies. Journal of Agricultural and Food Chemistry. 2002, 50, 4048–4054.
- Gomez-Miguez, M.J.; Cacho, J.F.; Ferreira, V.; Vicario, I.M.; Heredia, F.J.; Volatile Components of Zalema White Wines. Food Chemistry. 2007, 100, 1464–1473.
- Jiang, B.; Xi, Z.M.; Luo, M.J.; Zhang, Z.W.; Comparison on Aroma Compounds in Cabernet Sauvignon and Merlot Wines from Four Wine Grape-Growing Regions in China. Food Research International. 2013, 51, 482–489.
- Ferreira, V.; Lopez, R.; Cacho, J.F.; Quantitative Determination of the Odorants of Young Red Wines from Different Grape Varieties. Journal of the Science of Food and Agriculture. 2000, 80, 1659–1667.
- Tao, Y.S.; Zhang, L.; Intensity Prediction of Typical Aroma Characters of Cabernet Sauvignon Wine in Changli County (China). LWT: Food Science and Technology. 2010, 43, 1550–1556.
- Liang, H.Y.; Chen, J.Y.; Reeves, M.; Han, B.Z.; Aromatic and Sensorial Profiles of Young Cabernet Sauvignon Wines Fermented by Different Chinese Autochthonous Saccharomyces cerevisiae Strains. Food Research International. 2013, 51, 855–865.